Browse Course Material
Course info.
- Prof. Lionel C. Kimerling

Departments
- Materials Science and Engineering
As Taught In
- Electrical Engineering
- Electronic Materials
Learning Resource Types
Principles of engineering practice, case studies.
Case Study and Project Reports will be presented by the assigned Teams. The process is as follows:
Prof. Kimerling will lead a short in-class discussion on the approach for each Case Study or Project. Q&A is encouraged to clarify specific details.
The Instructors and TAs will moderate online Discussion Forums: within this Forum students should post their team’s tentative outline, develop concepts, discuss sources and preliminary findings. Instructors and TA will provide feedback within this Discussion Forum.
An optional office meeting with Prof. Kimerling is available if desired by any Group.
On the day of presentations, each Group must present a 20 minute presentation of 5-6 slides. Each member of the Group must present one slide from this presentation. Slides must be posted to the Web site the night before.
Students are expected to bring hard copies of all presentations to class.
Corrected slides and a final 2-page report must be posted to the Web site two days after presentation.
Grade assignment for the Case Studies and Projects will account for the following:
- presentation and writing skills
- clarity and rationality of the design execution
- presentation of background, issues, alternatives and conclusions
All student work is presented with permission of the authors.
Assignments | Resources |
---|---|
An Ethical Engineer? ( ) | Pinker, Steven. “ .” New York Times, January 13, 2008. |
Big Infrastructure Engineering ( ) | |
Social Construction of TV History ( ) | |
Semiconductors and Learning Curves; Steel Technology and Big Infrastructure ( ) | “Semiconductors and Learning Curves.” ( ) “Steel Technology and Big Infrastructure.” ( ) |

You are leaving MIT OpenCourseWare
Thank you for visiting nature.com. You are using a browser version with limited support for CSS. To obtain the best experience, we recommend you use a more up to date browser (or turn off compatibility mode in Internet Explorer). In the meantime, to ensure continued support, we are displaying the site without styles and JavaScript.
- View all journals
- ADVERTISEMENT FEATURE Advertiser retains sole responsibility for the content of this article
A case study in development of material science
Produced by
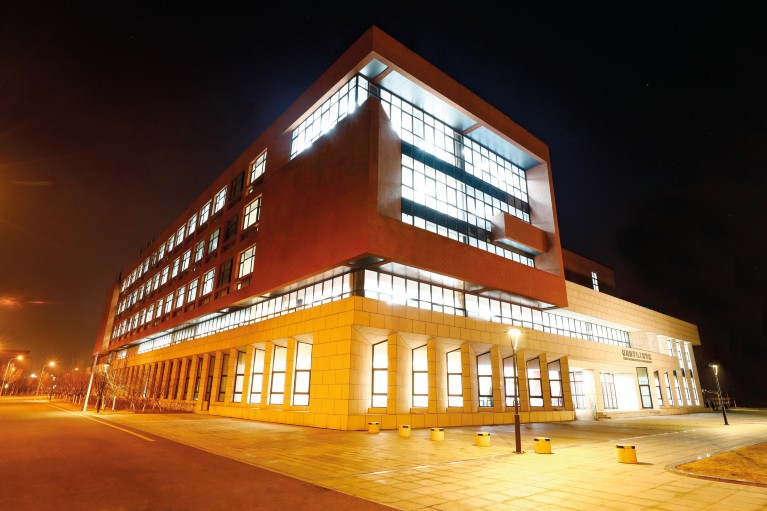
Credit: Nankai University
China’s significant increase in research investment has already brought a boom in the country’s research output. The gains are probably best illustrated in the field of material science, in which the number of publications has grown from almost none in the early 1980s to the largest in the world now. With applications ranging from manufacturing and electronics, to energy and biomedicine, material science is of profound interest to government funders and a field on which national economies rely heavily.
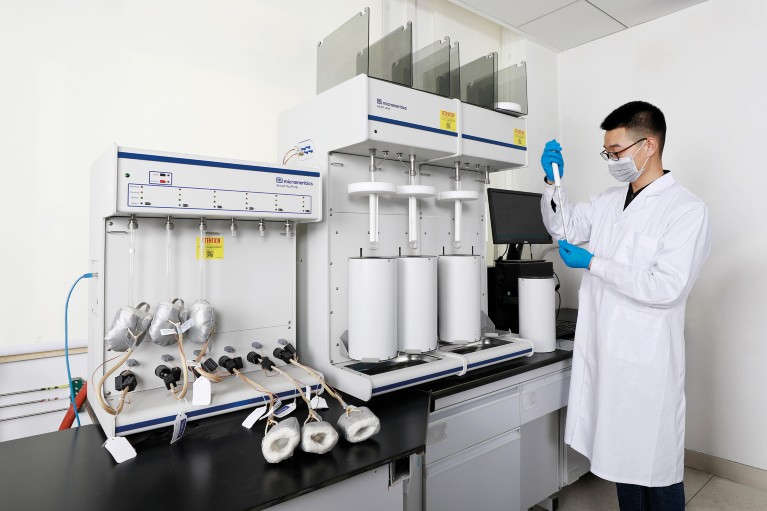
Data from the Essential Science Indicators (ESI) in 2018 show that Nankai is ranked among the global top 1.2‰, or 97th, in material science, up from 116th in mid-2017. It leads among Chinese universities in the number of citations per publication in journals listed in the Science Citation Index (SCI). Two MSE faculty members were listed among the 2018 Global Highly Cited Researchers by Clarivate Analytics.
Booming research results
One area of leading research performance at MSE is rare earth functional materials, with 30 highly cited papers tracked by ESI. “China has abundant rare earth resources, but a comprehensive scheme for using them is yet to be developed,” said Yan Chunhua, a member of the Chinese Academy of Sciences (CAS), and head of the MSE’s Centre for Rare Earth and Inorganic Functional Materials. The centre’s researchers develop novel technological processes of rare earth products and explore new applications of rare earth materials, ranging from energy and catalysis, to high-precision manufacturing and sensors. Their results have led to more than 10 patents.
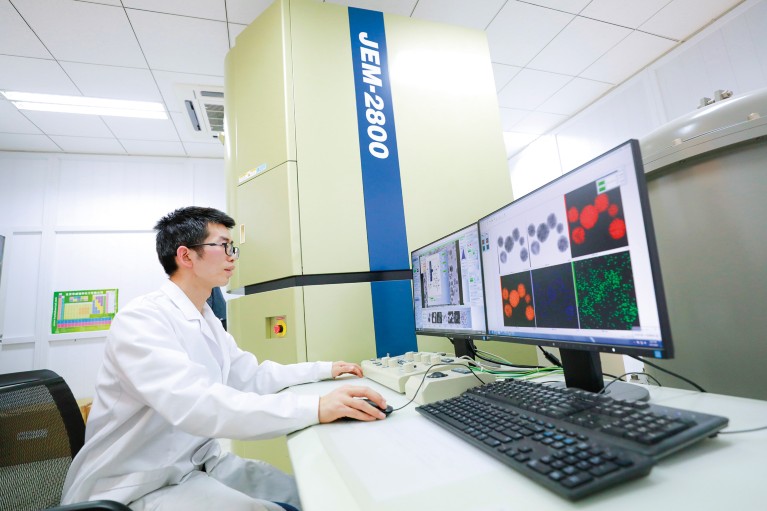
Prize-winning science
At the MSE Centre for Optical, Electrical, and Magnetic Materials, Bu Xian-He, the centre head and dean of MSE, leads a group that focuses on constructing inorganic solid materials for energy storage and conversion. They won the second prize of the National Natural Science Award in 2014 for their work on the synthesis, structure modulation, and properties of coordination polymers. Researchers at the centre also work on inorganic-organic hybrid magnetic and polymer materials, and construction of functional molecular aggregate materials.
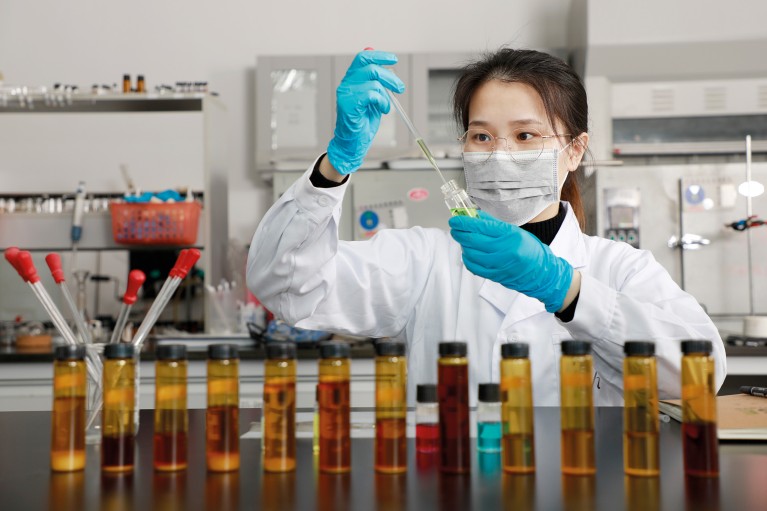
MSE researchers on new catalytic materials have established a new methodology for characterizing highly active intermediates in catalytic reactions by integrating technologies of chemical capture, spectroscopic analysis and computational simulation, which has facilitated the study of molecular sieve materials. They have also developed the AlPO-34 molecular sieve, a crystalline material with regular pores the size of a molecule for catalysis. Their results have gained 20 national patents.
To meet growing needs for new materials and devices for information and energy, researchers at the MSE Centre for Photonics/Electronic Materials have made progress in silicon-based illumination devices. They have designed nano-layered compound films with specific photoelectric properties and developed their applications in sensors, supercapacitors and other devices.
Outlook to the future
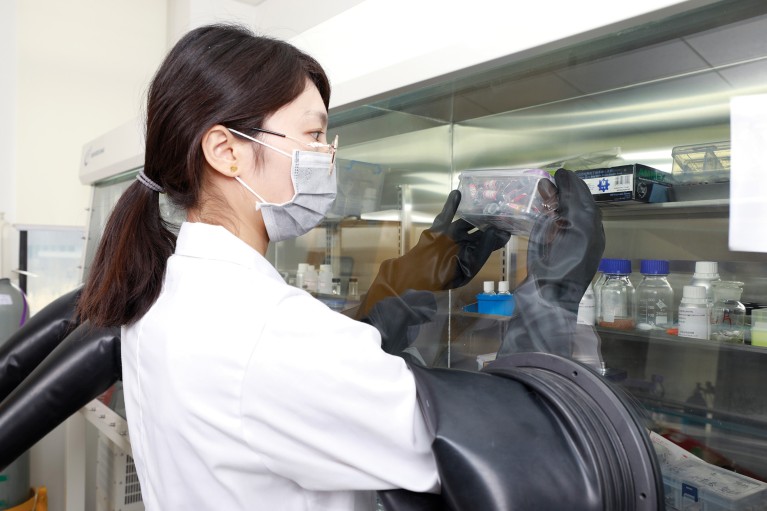
In an effort to develop cross-disciplinary and application-based research, the school is growing its computational materials science and materials genome research. Investments are also being made to advance research on biomedical, environmental, and intelligent materials and devices to better meet societal demands. In educational programmes, MSE encourages the integration of natural science and engineering to form interdisciplinary teams.
As Nankai University has received government support for building a world-class university, MSE is leveraging university and government resources to expand its current provincial and national key research platforms. Continual investment and infrastructure support will ensure a vibrant future for MSE.
+86 (0)22 85358786
http://mse.nankai.edu.cn
Quick links
- Explore articles by subject
- Guide to authors
- Editorial policies
- Hispanoamérica
- Work at ArchDaily
- Terms of Use
- Privacy Policy
- Cookie Policy
Pushing Boundaries with Bamboo: A Structural Engineering Case Study
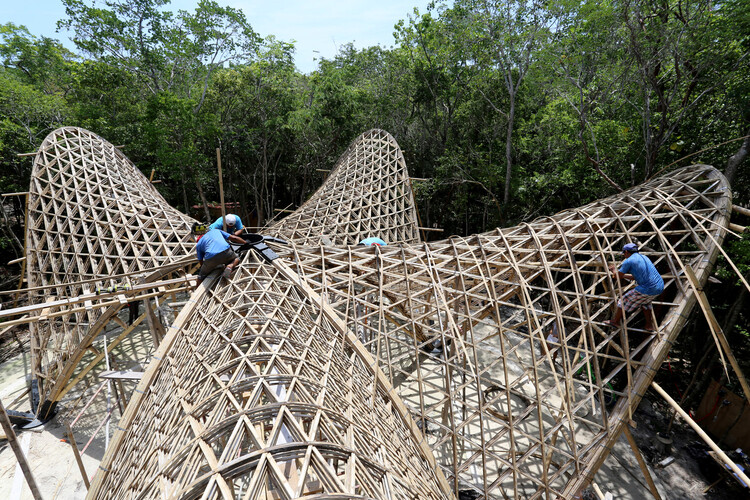
- Written by Rodrigo Istchuk
- Published on September 26, 2023
In a world grappling with environmental challenges, forward-thinking architects and engineers are increasingly leaning towards more sustainable solutions. While steel and concrete have long dominated the construction industry, bamboo is now stepping into the limelight as a compelling alternative. Thanks to its potent mix of strength, flexibility, and eco-friendliness, bamboo is fast becoming the go-to material for those keen on pushing the boundaries of sustainable architecture.
Bridging this transformative moment in sustainable design is the timely arrival of the "Bamboo Structures" eBook series, which this article is based on. Acting as a touchstone in the field, the first volume focuses on the structural design process behind the Luum Temple —a masterclass in bamboo engineering. The guide serves as more than just a theoretical text; it is a comprehensive manual teeming with real-world insights, cutting-edge research, and practical expertise.
The use of bamboo as a structural material is far from a new phenomenon; it has a rich history that stretches back thousands of years. Despite its ancient roots, much of this traditional knowledge has been underestimated as the modern construction sector became overly focused on mineral-based materials. Today, however, bamboo is experiencing a global resurgence, bringing to light a harmonious blend of ancient wisdom with modern engineering. This fusion not only offers new avenues for sustainable architecture but also feels like a long-awaited, inevitable evolution of the construction industry.
The Luum Temple: An Icon of Sustainable Design
Nestled in the lush landscapes of Tulum, Mexico, the Luum Temple is more than just an eye-catching architectural marvel; it's a pioneering example of what happens when traditional wisdom meets modern engineering. Designed to stand against 250 km/h hurricane winds and significant seismic forces, this structure embodies the compelling possibilities that arise when we harness bamboo’s unique properties as a structural material.
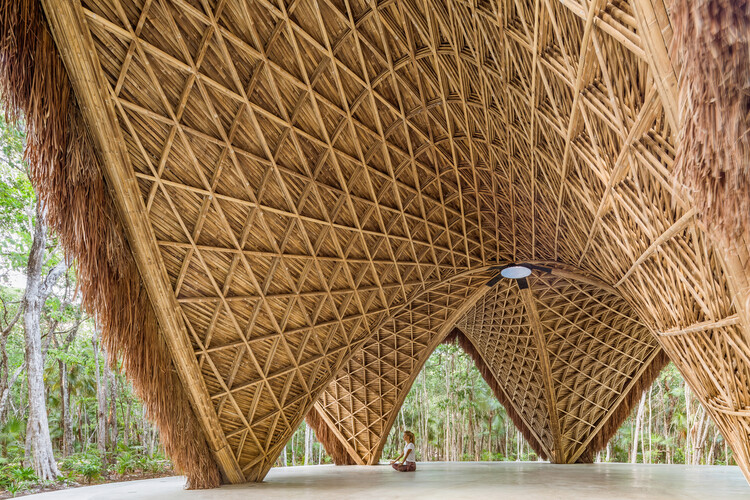
The architectural vision was conceptualized by the CO-LAB Design Office , brought to life by Architectura Mixta , and inspired by the iconic work of legendary architect Felix Candela . The engineering was led by Esteban Morales , who describes the structural system as five intersecting hyperbolic paraboloids made of bamboo arches and split bamboo beams.
Bamboo’s tensile strength-to-weight ratio often surpasses that of steel, making it not just strong but also lightweight. Flexibility is another advantage: especially when used in split form, bamboo enables architects to craft organic, flowing shapes that defy traditional geometrical constraints, facilitating the adoption of innovative structural approaches that rely on shape stiffness and biomimicry. Bamboo ’s rapid growth and ability to sequester carbon also position it as an environmentally superior alternative to traditional construction materials, including timber.
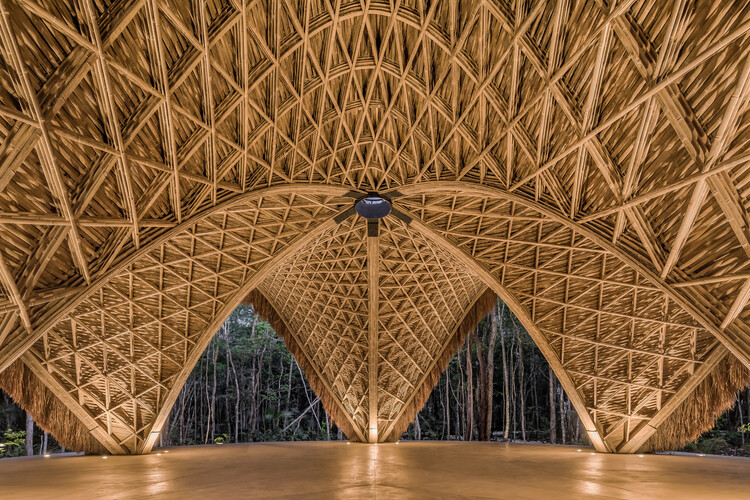
On the other hand, working with bamboo also requires special care in certain aspects. Due to its lightweight nature, particular attention must be paid to foundations, cross-bracing and structural stiffness, which are essential to counterbalance its vulnerability to wind forces. Although bamboo's low shear strength presents a challenge, an adequate comprehension of its properties allows for creative systems to compensate for this, and a critical focus on treatment is also non-negotiable. The material can also be prone to insect attacks and has a limited lifespan, making it important to include proactive 'preservation by design' approaches as an integral part of any bamboo-based construction.
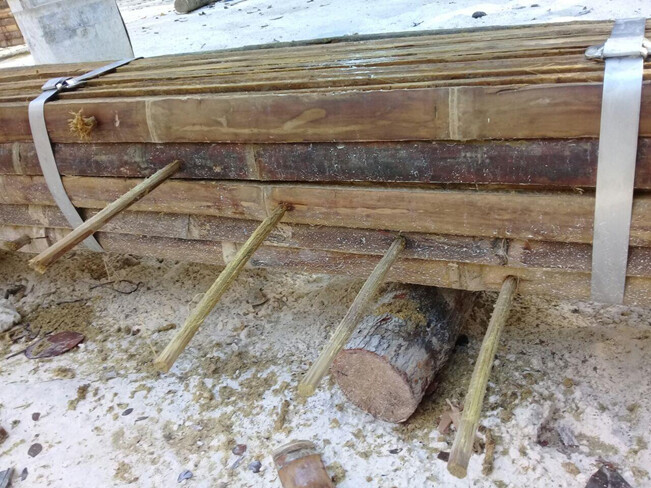
The Luum Temple embraces a balance between these advantages and challenges. The interconnected roof diaphragm, for instance, is made of cross-layers of bamboo mats —a modern nod to empirical techniques of the past that give the structure impressive resistance to lateral loads. To account for bamboo's natural flex and movement, the structure incorporates articulated foundations and a central compression ring. This approach not only captures bamboo's intrinsic strengths but also circumvents its limitations, demonstrating a model way to build sustainably and innovatively.
The Engineering Process
A defining feature of the Luum Temple project was its successful integration of up-to-date bamboo engineering concepts with a timeless architectural framework. Rather than merely following standard procedures, the engineering team ventured into new territory by developing custom methods, all while adhering to internationally recognized standards. This inventive approach adeptly navigated through Mexico's intricate regulatory framework surrounding bamboo construction and also set a solid precedent for future bamboo projects.
The technical workflow of the project was facilitated by a robust software toolkit. The structural design process started with AutoCAD 3D, where the structure’s geometry was modeled and compatibilized for the next steps. Following this, structural analysis was carried out using specialized structural analysis software SAP2000 v.14.
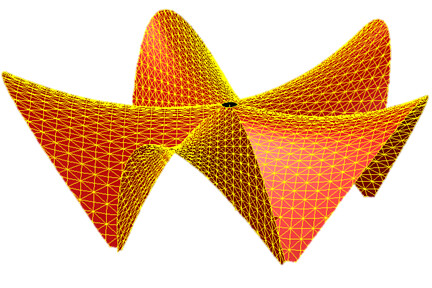
The structure was divided into three main element types to orient the design process:
- Arches , spanning in the edges of the structure and radially from the center;
- Joists , spanning in layers and connecting the main arches over several layers;
- Roof diaphragm , conformed by the rigid surface resulting from several layers of joist triangulation and split weaving below the roof.
As the project is located in a region prone to hurricane winds and seismic activities, the engineering strategy emphasized shape stiffness, triangulation, and a robust diaphragm. These elements were specifically selected to offset lateral forces that could compromise the structure. Consequently, each component in the structure was interconnected and designed to function cohesively, ensuring resilience against some of nature's most extreme forces.
In terms of load magnitudes, considerable seismic loads and 250km/h hurricane wind loads governed the design. These were combined with dead loads (weight of the structure and roofing) and live loads to obtain the design internal forces for each element of the structure. For this, the allowable stress method was employed, complying with the most adequate approach for structural bamboo design. The loads were then multiplied by the prescribed load coefficient for each of the required load combinations according to bamboo-specific structural codes. Finally, the maximum internal forces found in the most stressed arch, joist, and roof diaphragm elements were considered for the final design of the structural elements.
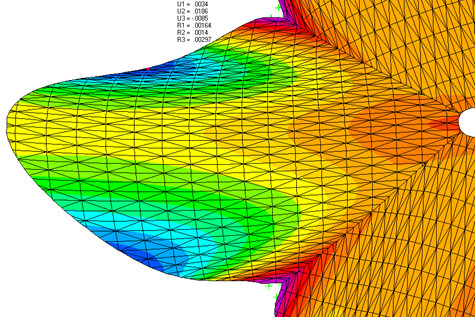
Equipped with structural insights, such as the internal forces acting in the structure for each load combination, the structural components were designed based on bamboo-specific building codes, such as the NSR-10 (Colombia) and the ISO 22156 (International). Specialized software like WoodWorks® Connections, SFS Timber Work, and APF Wood Joint were then employed for the design of the connecting elements between structural components.
At the uppermost part of the structure, the primary arches converge, interconnected by a central steel compression ring. This design feature ensures each arch tip converges towards a centralized point, reminiscent of a star's radiating arms. Drawing a parallel to airplane wings, which flex and bounce to a certain extent during flight to prevent structural failure, bamboo structures adopt a similar engineering principle. To ensure the bamboo components can flex and adapt during adverse events like storms or earthquakes without fracturing, the central compression ring incorporates robust hinged connections, as illustrated in the subsequent images.
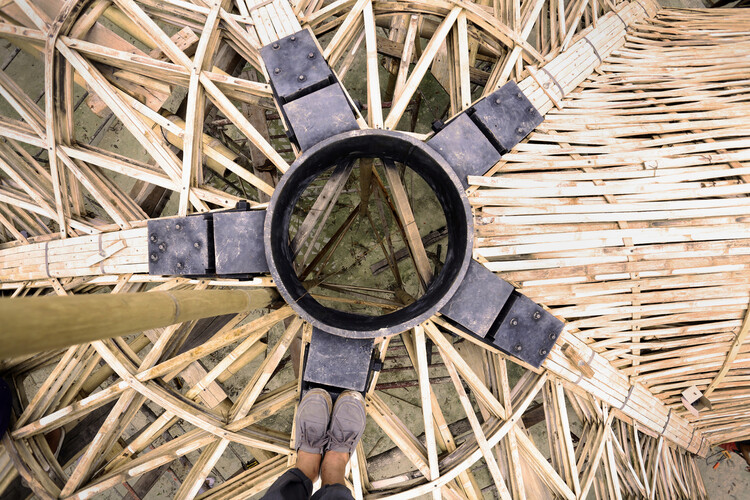
In this project, a detailed analysis was undertaken to determine the mechanical connections at the base. Designed in a hinged manner, similar to the central compression ring, the connections between bamboo arches and the foundation permit rotational movement. This design strategy ensures that bamboo elements are spared from excess shear and bending forces, which aren't their strengths. Instead, the bamboo primarily experiences tension and compression forces parallel to its fibers.
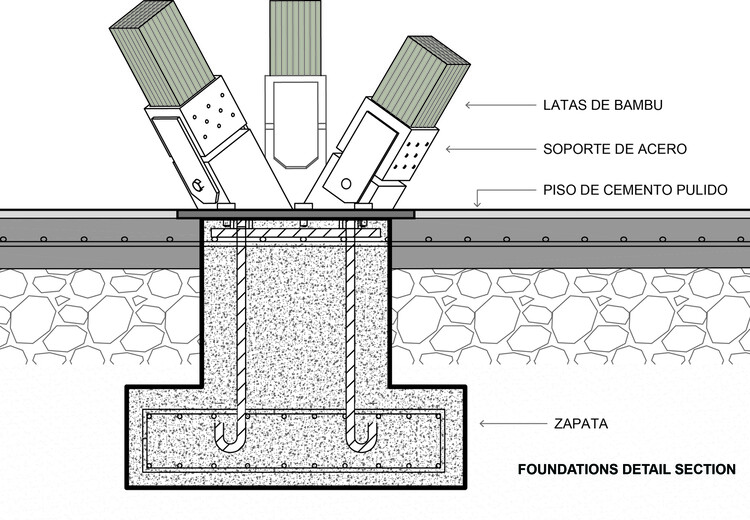
As we've seen, bamboo isn't just a rapidly renewable resource —it's a structural material with the potential to change the landscape of sustainable architecture in the upcoming decades. From its historical roots to its modern-day applications, bamboo proves that when tradition meets innovation, magic happens.
Unlock the Potential of Bamboo Structures
For those serious about pioneering the next wave of green construction and understanding the practical aspects of using bamboo as a structural material, the "Bamboo Structures" eBook series can be an essential guide. This recent publication dives deep into methodologies, design details, and example applications, serving as an invaluable resource for both novices and experts in the field. Developed based on the structural calculation methods used for the Luum Temple project, this first volume addresses a long-awaited resource gap, offering a clear lens into bamboo structural design for the wider audience.
Learn more about the “Bamboo Structures” eBook here.
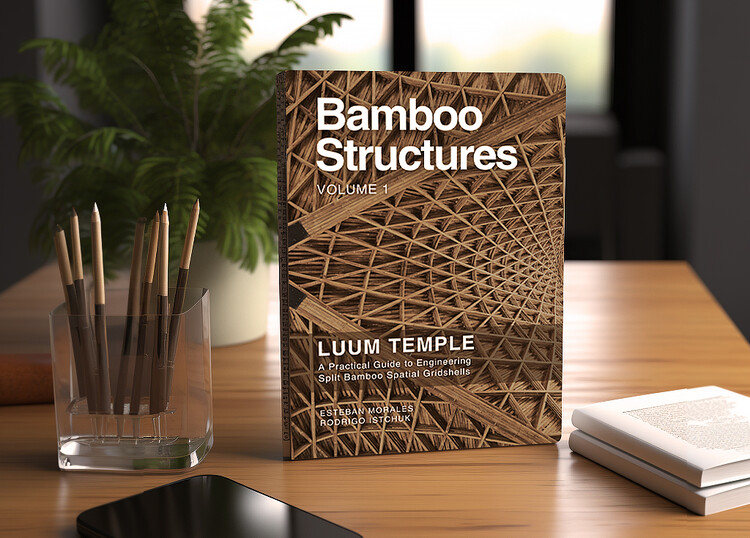
Image gallery
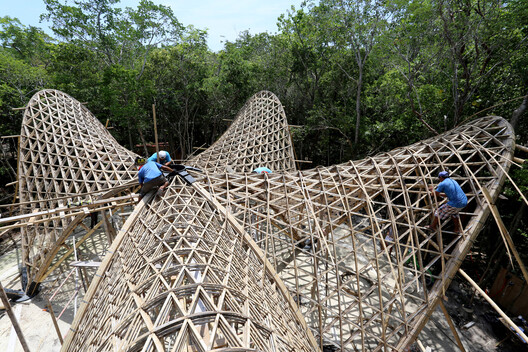
- Sustainability
世界上最受欢迎的建筑网站现已推出你的母语版本!
想浏览archdaily中国吗, you've started following your first account, did you know.
You'll now receive updates based on what you follow! Personalize your stream and start following your favorite authors, offices and users.
Mechanical Properties of Engineering Materials: Relevance in Design and Manufacturing
- In book: Introduction to Mechanical Engineering (pp.3-41)

- Mountain State University
Abstract and Figures

Discover the world's research
- 25+ million members
- 160+ million publication pages
- 2.3+ billion citations
- Rachasit Jeencham
- Nantawat Chiaoketwit
- Piya-on Numpaisal

- Stephen O. Olanrewaju

- Theophilus T. Ewetumo

- Dejana Pejak Simunec
- Adrian Trinchi

- Ahmet Yokuş
- Arinze Everest Chinweze

- Heru Sukanto

- Joko Triyono

- Poom Narongdej
- Mana Hassanpour
- Nicolas Alterman
- Ehsan Barjasteh

- S. P. (Stuart P.) Keeler
- ENG FRACT MECH
- G.R. Johnson
- Rolf E. Hummel
- R. Von Mises
- Gorton M. Goodwin
- Frederick Winslow Taylor
- Recruit researchers
- Join for free
- Login Email Tip: Most researchers use their institutional email address as their ResearchGate login Password Forgot password? Keep me logged in Log in or Continue with Google Welcome back! Please log in. Email · Hint Tip: Most researchers use their institutional email address as their ResearchGate login Password Forgot password? Keep me logged in Log in or Continue with Google No account? Sign up
MSE 5090: Case Studies in Material Selection
Case study topics.
Reserve desk - Wadley, H. and R. Vancheeswaran. "The Intelligent Processing of Materials: An Overview and Case Study", January, 1998. 19-30. This case study analyzes new process models and simulation tools that are used to "explore the processibility of different composite systems and identify optimum process schedules; in conjunction with microstructure sensors, they can now be used for model-based feedback control." | |
Reserve desk - " , Getting Good Wood (or Aluminum) on the Ball", Mechanical Engineering, October, 1990. 40 - 47. This article discusses the increasing technical sophistication of the baseball bat design and material choice to produce high-performance bats. | |
Reserve desk (student case study) - Smith, J., "Materials Selection for a Bicycle Frame", 1993. This case study explores "the suitability of 6061 aluminum, 6Al/4V titanium, graphite fiber reinforced epoxy, 4130 steel, and a SiC/Al metal matrix composite" for use in bicycle frames. | |
Reserve desk (student case study) - Cann, M., "Material Selection for a High Performance Ice Axe", 1998. This case study is by an engineer for Black Diamond Equipment, Inc., a Salt Lake City manufacturer of climbing equipment. The goal is to "research different axe shaft materials and come to a final recommendation based on the identified functional requirements and constraints." | |
Reserve desk - Lewis, G., A Vaulting Pole, Chapter 14, Prentice Hall, Englewood Cliffs, New Jersey, 1990. 132 - 137. "From the viewpoint of material selection, the objective is to choose a material that has a very high value of specific endurance strength and whose use would result in the lowest values of (a) the weight of the vaulting pole, for a given "pole stiffness", and (b) the total cost of the pole (raw material plus fabrication costs). | |
Reserve desk (student case study) - Harper, D., "Case Study: A Look inside Snowboard Construction Components", 1998. This case study "compares and contrasts an ideal case study with one performed by DNR, USA. The study involves replacing a European produced rubber component found in the tip and tail regions of the Santa Cruz freestyle snowboards with a domestically produced material with similar material properties. This study takes a material engineering approach to choosing a material and compares it to the actual steps that were taken to reach a solution." | |
Reserve desk - Lewis, G., Skateboard Wheels, Chapter 16, Prentice Hall, Englewood Cliffs, New Jersey, 1990. 143 - 148. This case study focuses on the selection of the materials for skateboard wheels. This is a case where the "best material" based on data is not the one used because of cost and other constraints. | |
Reserve desk - Anderson, D. "Material Demands for ", MRS Bulletin, August, 1988. 30 - 35. In this article the author presents a cursory look at optical neural networks and focuses on architectures that employ photo refractive materials. He concludes with "we need a medium that is to neural networks what silicon is to digital computers. Optical approaches reveal their promises, and we look to materials technology advances to realize them." Does that medium now exist? | |
Reserve desk (student case study) - Baczuk, J. and G. Gordon, "Lightweight, Dimensionally Stable, Polymer-Ceramic Composites for Space Systems & Commercial Applications", 1998. LoTEC, Inc., a Low Thermal Expansion ceramics company in SLC specializes in NZP, a material that exhibits near-zero thermal expansion . The applications for which this material has an advantage are discussed , along with constraints in manufacturing the material. | |
Reserve desk (student case study) - Larson, R., "An Investigation into the Possibility and Feasibility of Using Thermoplastic Elastomers in Automobile Tires", 1993. This case study was motivated by the unrecyclability of tires. In addition to recyclability, thermoplastics have a simpler manufacturing process. They do have other problems, including the limitation of having the molding be reversible by heat. In the conclusion, several questions are proposed for additional research. | |
Reserve desk (student case study) - Laramee, R., "Case Study, Thiokol Corporation, Aerospace Propulsion Nozzle Material Evaluation", 1990. "This case study relates to the engineering evaluation of downsizing by 50% the shuttle boosters to meet the propulsion requirements of the Advanced Launch Systems (ALS)." | |
Reserve desk - Lewis, G., Sounding Board of an Orchestral Harp, Chapter 13, Prentice Hall, Englewood Cliffs, New Jersey, 1990. 125 - 131. This case study focuses on the problem of "board creep." When the harp is tuned elastic deformation occurs. Eventually the strain may cause the sounding board to separate from the the sounding box, rendering the instrument unplayable. | |
Reserve desk (student case study) - Lobo, S., "Design Criteria and Selection of an Optimal Blood Analogue Fluid", 1993. This case study attempts to select a fluid with "optimally-matched fluid mechanical parameters" to blood and "to recommend the standardized use of such a fluid in subsequent flow investigations of blood." | |
Reserve desk (student case study) - Mulder, M., "Material Selection for Small Vascular Grafts", 1993. "This study was conducted in response to questions raised by a group of investors who are interested in exploring the financial incentives of investing in small diameter vascular grafts...Vascular grafts are porous polymer tubes which are used to replace natural blood vessels or reroute blood around an obstructed vessel." | |
Reserve desk - Lewis, G., Question 53, Coatings for Human Teeth, Prentice Hall, Englewood Cliffs, New Jersey, 1990. 399 - 402. Brief description of enamel, dentin, and cementum, and all parts of the human tooth from materials science viewpoint. Identifies material requirements for a human tooth coating. Compares performance of natural enamel with candidate materials. | |
Reserve desk (student case study) - Pardey, H., "Replacement for Hard Chrome Surfaces in Hydraulic Cylinders", 1993. "This case study is to determine an alternate material to replace the hard chrome surfacing on diamond drill hydraulic chuck cylinders and pistons." | |
Reserve desk (student case study) - Chandra, D., "Case study of selection of Materials for Automobile Body Panels". "In the present study selection of materials for automobile body panel is elucidated and a performance index is prepared for materials chosen." The purpose is to find "new materials which can replace high energy consuming conventional materials." | |
Reserve desk (student case study) - Billings, E., "Electrosurgical Scalpels". In this case study, the materials restrictions for materials to be used in as electrosurgical scalpels were analyzed and used to select materials for this application." | |
Reserve desk - Destefani, J., "Advances in Intermetallics", Advanced Materials & Processes, 2/98. 37 - 41. This article looks at the processing and properties of intermetallic compounds. Composites discussed include Ni Al, Ti Al, and NiAl. | |
Reserve desk - Lewis, G., Flat Spiral Spring Chapter 23, Prentice Hall, Englewood Cliffs, New Jersey, 1990. 194 - 197. "The objective is to use the spring to store energy and to recover the same either slowly or rapidly, depending on the function of the spring. Thus the flat spiral spring finds itself in applications such as clocks, watches, and gramophones, as are found in antique shops or in private bric-a-brac collections." | |
Reserve desk - Bowman, R. and R. Noebe, "Up-and-Coming IMCs", Advanced Materials & Processing, 8/89. 35 - 40. This article discusses the development and testing of Intermetallic-matrix composites (IMCs). It also features the fabrications methods, including powder cloth, fiber/foil, arc and plasmal thermal spray. | |
Reserve desk - Lewis, G., Pressure Capsule and Outer Hull of a Deep Sea-Operating Vehicle, Chapter 1, Prentice Hall, Englewood Cliffs, New Jersey, 1990. 1 - 9. "The objective in flywheel design being to store the maximum amount of kinetic energy per unit mass of the flywheel, the two criteria of performance are thus stored kinetic en`ergy and flywheel mass. In analyzing for the optimum material, the discussion is limited to thin flywheels so that plane stress conditions may be assumed." | |
Reserve desk - Jagielinski, T. "Materials for Future High Performance Magnetic Recording Heads", MRS Bulletin, March, 1990. 36 - 43. This article states that "Conventional ferrite heads will be eliminated from high performance recording systems and ...The future lies with multilayers and artificial structures in which properties can be engineered independently during the fabrication process." Eight years later, is this true? | |
Reserve desk - "Diamond Thin Film: Hot New Material for the 90's", Design News, 4-10-89. 70 - 75. This article explores the processing and potential uses for diamond film. It suggests that "the 1990s could become the decade of the diamond." Was he right? | |
Reserve desk - Hadden, Cdr, P., "Selection of materials for a modern warship", Royal Naval Engineering College, Manadon, Plymouth, Devon PL5 3AG. "In the design of a modern warship, operational effectiveness must be balanced against cost, reliability, and maintainability. This article illustrates the extent to which the design process affects the selection of materials employed in the construction of the latest class of anti-submarine warfare ship, the Type 23, and emphasizes the increasing extent that cost plays." | |
Reserve desk - Lewis, G., Pressure Capsule and Outer Hull of a Deep Sea-Operating Vehicle, Chapter 10, Prentice Hall, Englewood Cliffs, New Jersey, 1990. 96 - 103. "Both the deep submersible and the deep submergence vehicles require critical designs to obtain the optimum mix of operating depth and payload. One way of achieving such a mix is through proper selection of materials for the key structural components, the pressure capsule and the outer hull." | |
Reserve desk - Lewis, G., Thermal Shock Resistance, Chapter 7, Prentice Hall, Englewood Cliffs, New Jersey, 1990. 56 - 73. "Thermal shock is the phenomenon whereby a body experiences instantaneous or stepwise changes in temperature due to rapid change in either external temperature or the rate of internal heat generation. As a result of these changes, internal thermal stresses are generated that may weaken the material or, in extreme cases, lead to fracture." | |
Reserve desk - Lewis, G., Shape Memory Effect Applications, Chapter 11, Prentice Hall, Englewood Cliffs, New Jersey, 1990. 96 - 103. "The shape memory effect (SME) is the ability of certain alloys to 'remember' the dimensions that a basic shape (wire, rod, sheet, table) possessed at a previous point in its thermomechanical history and to work to recover those dimensions upon heating above and/or cooling below a characteristic temperature, termed the transformation temperature or temperature range." | |
Reserve desk - Lewis, G., Receiver of a High-Temperature Solar Energy Thermal System, Chapter 24, Prentice Hall, Englewood Cliffs, New Jersey, 1990. 198 - 201. "The receivers in SETS are the key components that determine the operating temperature at which power conversion or thermal processes can take place. The achievement of high operating temperatures (greater than 800°C.) makes possible higher-efficiency engines and an increased number of useful industrial processes. | |
Reserve desk - Lewis, G., Drive shaft of a Medium-sized Car, Question 2, Prentice Hall, Englewood Cliffs, New Jersey, 1990. 243 - 249. "The use of filamentary composite materials in automotive vehicle components is on the increase. (a) Demonstrate the plausibility of this statement. (b) State the attractive features of these materials for one automotive vehicle component, the drive shaft of a medium-sized car." | |
Reserve desk (student case study) - Rudert, J. " Selection of a Material for the Walls and Ceiling of a Low-Cost Paint Booth", 1998. "This case study concerns the selection of a material for the walls and ceilings of a low-cost automotive paint booth. The constraints include maximum stiffness per unit weight, ease of assembly of the final product, toxicity of the material during processing and in case of a fire, cost. | |
. | Reserve desk - Lewis, G., Materials for Hypervelocity Vehicles, Chapter C1, Prentice Hall, Englewood Cliffs, New Jersey, 1990. 433 - 440. "The selection of materials for use on external surfaces of hypervelocity vehicles is governed primarily by the need to protect these sections from the very high temperatures created by aerodynamic heating during flight. To keep the weight of these vehicles to the minimum possible, high-temperature-resistant but light-weight materials, rather than thermal protection shields, are preferred." |
. | Reserve desk (student case study) - Knudsen, M., "Materials Selection and Performance in the Chemical Process Industries: Case Studies", 1998. "Case studies taken from three actual projects in differing industries where specific materials selection was required were reviewed. The Ashby method was used in each case...The Ashby system worked well for these cases." |

Engineering Databases
- Academic Complete E-books
- Academic Search Complete
- Access Engineering
- ACM Digital Library
- American Chemical Society
- Applied Science and Technology Source
- ASM Handbooks
- ASME Digital Collection
- ASTM Compass Standards
- Auto Repair Source
- AVON - Academic Video Online
- Dictionary of Natural Products
- Digital Commons
- EBSCO eBooks Collection
Engineering Case Studies
- Google Scholar
- Grammarly Premium
- IEEE Xplore
- Journal Citation Reports
- Journal List A-Z
- JSTOR Collection
- Knovel Science & Engineering Datasets
- LinkedIn Learning
- MeLCat: Music, Books, Audio & More from all over Michigan
- SAE Mobilus
- Science Direct
- Small Engine Repair Reference Center
- Springer Journals and eBooks
- Web of Science
- WorldCat: Books, DVDs, CDs & Articles from around the world
- About the Database
- Creating Persistent Links

Creating a Persistent Link:
Engineering Case Studies has an option for persistent links inside each resource.
To locate the persistent link, select the resource you wish to share. Beneath the resource, you will see an option to "embed/link":

From here, you can choose to copy the persistent link from the pop-up window:

Using Engineering Case Studies

The analysis of engineering failures is an essential part of many engineering curricula today. This focus enables modern engineers and scholars to learn what not to do and how to create designs with a greater chance of success. Key to learning is establishing the nature of each failure—structural, corrosive, electrical, etc.—and understanding that element.
The engineering field needs a comprehensive and authoritative resource to provide in-depth, impartial analysis of key engineering failures. Engineering Case Studies Online is a collection that will grow to include 250 hours of video and 50,000 pages of text resources to meet this growing need. Content is displayed alongside targeted learning objects designed to facilitate detailed understanding of the causes and impact of these failures. Materials in the collection include:
• Feature-length video documentaries of major failures.
• Monographs explaining cases in detail and describing key engineering concepts and issues.
• Simulations depicting precisely what went wrong.
• Primary footage of accidents, including related news segments.
• Audio footage and transcripts.
• Testimonies from participants, victims, and witnesses.
• Images, accident reports, blueprints, and other key archival content whenever available.
• Bibliographies of authoritative materials from newspapers, Web sites, and journals.
• Specially written cases that explore engineering ethics.
The collection provides in-depth coverage for more than 50 of the most frequently taught and seminal case studies around the world together. Example cases include:
• Air France flight 4590 | • Comet airliners | • Space shuttle Challenger |
• Apollo 13 | • Deepwater Horizon oil rig | • Tacoma Narrows bridge |
• The Big Dig | • Ford Pinto | • Titanic |
• Boeing 787 Dreamliner | • Fukushima Daiichi nuclear power plant | • TWA flight 800 |
• Chernobyl power plant | • Hyatt Regency walkway | • Plus many more |
All materials in Engineering Case Studies Online are carefully cultivated by expert editors, with the assistance of an advisory board of engineering librarians and faculty. Materials come from a wide range of content partners, including the Digital Rights Group, BBC, Future Media, TVF International, as well as leading academic publishers such as Princeton University Press, John Wiley & Sons, Harvard University Press, and engineering associations such as the American Society of Civil Engineers.
More than 60 percent of video material in the collection was produced since 2000, and new content is added regularly. The database is global in focus, and originates from the United States, the United Kingdom, and Australia, with selections from across Europe and Asia. And because Engineering Case Studies Online addresses cases from a variety of angles, including their legal and ethical implications, the collection has broad applications for fields including architecture, business, law, urban planning, health and safety, environmental studies, science, sociology, media, and technology.
- Last Updated: Sep 3, 2024 3:38 PM
- URL: https://libguides.kettering.edu/EngineeringDatabases
|
|
|
- Products & Services
- Engineering News
- Standards Library
- Reference Library
I forgot my password.
Don't have an account?
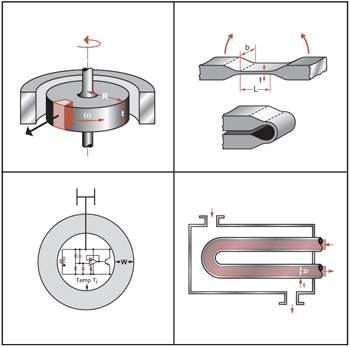
6.1 Introduction and Synopsis
Here we have a collection of case studies illustrating the screening methods of Chapter 5. They are deliberately simplified to avoid obscuring the method under layers of detail. In most cases little is lost by this: the best choice of material for the simple example is the same as that for the more complex, for the reasons given in Chapter 5. More realistic case studies are developed in later chapters.
Each case study is laid out in the same way:
the problem statement, setting the scene,
the model, identifying function, constraints, objectives, and free variables, from which emerge the attribute limits and material indices,
the selection in which the full menu of materials is reduced by screening and ranking to a short-list of viable candidates,
the postscript, allowing a commentary on results and philosophy.
Techniques for seeking supporting information are left to later chapters.
The first few examples are simple but illustrate the method well. Later examples are less obvious and require clear thinking to identify and distinguish objectives and constraints. Confusion here can lead to bizarre and misleading conclusions. Always apply common sense: does the selection include the traditional materials used for that application? Are some members of the subset obviously unsuitable? If they are, it is usually because a constraint has been overlooked: it must be formulated and applied.
Most of the case studies use the hard-copy charts of Chapter 4; Sections 6.17 and 6.18 illustrate the use of computer-based...
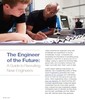
UNLIMITED FREE ACCESS TO THE WORLD'S BEST IDEAS
SIGN UP TO SEE MORE
GlobalSpec collects only the personal information you have entered above, your device information, and location data. GlobalSpec may share your personal information and website activity with our clients for which you express explicit interest, or with vendors looking to reach people like you. GlobalSpec will retain this data until you change or delete it, which you may do at any time.
Contact Preferences
By submitting your registration, you agree to our Privacy Policy .
This is embarrasing...
An error occurred while processing the form. Please try again in a few minutes.
Customize Your GlobalSpec Experience
Select your free newsletters, industry newsletters, select your free product alerts.
Breadcrumbs Section. Click here to navigate to respective pages.

Forensic Materials Engineering
DOI link for Forensic Materials Engineering
Get Citation
Most books on forensic engineering focus on civil engineering failures rather than consumer or general mechanical products. Unique both in scope and style, this treatment is built upon case studies of real accidents, broadly focused on consumer products, and dedicated to problem solving through scientific principles. Each well-illustrated case stud
TABLE OF CONTENTS
Chapter 1 | 24 pages, introduction, chapter 2 | 36 pages, materials in distress, chapter 3 | 24 pages, establishing the load transfer path, chapter 4 | 46 pages, a "toolbox" for forensic engineers, chapter 5 | 40 pages, failure due to manufacturing faults, chapter 6 | 44 pages, fluid transport, chapter 7 | 40 pages, failure of storage vessels, chapter 8 | 32 pages, accidents in the workplace, chapter 9 | 32 pages, failure of medical implements, chapter 10 | 30 pages, component failure in road traffic accidents, chapter 11 | 16 pages, fraudulent insurance claims, chapter 12 | 14 pages, criminal cases, chapter 13 | 50 pages, intellectual property cases.
- Privacy Policy
- Terms & Conditions
- Cookie Policy
- Taylor & Francis Online
- Taylor & Francis Group
- Students/Researchers
- Librarians/Institutions
Connect with us
Registered in England & Wales No. 3099067 5 Howick Place | London | SW1P 1WG © 2024 Informa UK Limited
Information
- Author Services
Initiatives
You are accessing a machine-readable page. In order to be human-readable, please install an RSS reader.
All articles published by MDPI are made immediately available worldwide under an open access license. No special permission is required to reuse all or part of the article published by MDPI, including figures and tables. For articles published under an open access Creative Common CC BY license, any part of the article may be reused without permission provided that the original article is clearly cited. For more information, please refer to https://www.mdpi.com/openaccess .
Feature papers represent the most advanced research with significant potential for high impact in the field. A Feature Paper should be a substantial original Article that involves several techniques or approaches, provides an outlook for future research directions and describes possible research applications.
Feature papers are submitted upon individual invitation or recommendation by the scientific editors and must receive positive feedback from the reviewers.
Editor’s Choice articles are based on recommendations by the scientific editors of MDPI journals from around the world. Editors select a small number of articles recently published in the journal that they believe will be particularly interesting to readers, or important in the respective research area. The aim is to provide a snapshot of some of the most exciting work published in the various research areas of the journal.
Original Submission Date Received: .
- Active Journals
- Find a Journal
- Proceedings Series
- For Authors
- For Reviewers
- For Editors
- For Librarians
- For Publishers
- For Societies
- For Conference Organizers
- Open Access Policy
- Institutional Open Access Program
- Special Issues Guidelines
- Editorial Process
- Research and Publication Ethics
- Article Processing Charges
- Testimonials
- Preprints.org
- SciProfiles
- Encyclopedia
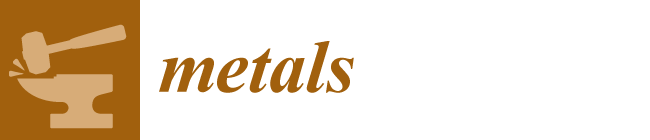
Article Menu

- Subscribe SciFeed
- Recommended Articles
- Google Scholar
- on Google Scholar
- Table of Contents
Find support for a specific problem in the support section of our website.
Please let us know what you think of our products and services.
Visit our dedicated information section to learn more about MDPI.
JSmol Viewer
Additive manufacturing of metal materials for construction engineering: an overview on technologies and applications.

1. Introduction
2. metal additive manufacturing technologies, 2.1. materials, 2.2. overview remarks, 2.3. features of 3d printing methods, 3. printing process parameters for metal am, 3.1. laser-related parameters, 3.2. scan-related parameters, 3.3. powder-related parameters, 3.4. temperature-related parameters, 3.5. printing directions and orientations, 3.6. effects of process parameters on the properties of 3d-printed metals, 4. metal additive manufacturing in construction, 4.1. optimized structural node by arup, 4.2. mx3d pedestrian bridge, 4.3. takenaka connector, 4.4. am steel reinforcement for concrete, 4.5. joining aluminium profiles, 4.6. future applications, 5. the potentials of metal am in topological optimization, 5.1. nonconventional geometries, 5.1.1. topology optimization.
Click here to enlarge figure
- SIMP method
- Level-Set method
- The application of topological optimization
5.1.2. Lightweight Components
5.2. use of am in repair of existing structures.
- Preparation stage. In the first step, considerations concern the cost-effectiveness of the repair/reinforcement of the degraded component. Next, a geometric check is performed between the worn elements and the nominal model. This comparison generates an error map, which highlights the errors between the two models. Finally, the repair area can be identified and judgements made on the extent of the damage.
- Production stage. In this stage, the previously identified area is repaired/reinforced through AM or hybrid manufacturing processes.
- Post-repair stage. In the final step, a geometric inspection is performed to verify the correct execution. In addition, the restored element can be mechanically characterised by means of material strength tests.
6. Conclusions
Author contributions, data availability statement, conflicts of interest.
- Xiang, H.; Zhou, Y.; Zhang, X.; Li, J.; Huang, Y.; Mou, G.; Wu, C. Supportfree Printing in Laser Powder Bed Fusion: Formation Mechanisms of Discontinuity, Dross and Surface Roughness. Opt. Laser Technol. 2024 , 177 , 111201. [ Google Scholar ] [ CrossRef ]
- Housholder, R.F. Molding Process. U.S. Patent 4247508, 27 January 1981. [ Google Scholar ]
- Wong, K.V.; Hernandez, A. A Review of Additive Manufacturing. ISRN Mech. Eng. 2012 , 2012 , 208760. [ Google Scholar ] [ CrossRef ]
- Chen, L.-Y.; Qin, P.; Zhang, L.; Zhang, L.-C. An Overview of Additively Manufactured Metal Matrix Composites: Preparation, Performance, and Challenge. Int. J. Extrem. Manuf. 2024 , 6 , 052006. [ Google Scholar ] [ CrossRef ]
- Coon, C.; Pretzel, B.; Lomax, T.; Strlič, M. Preserving Rapid Prototypes: A Review. Herit. Sci. 2016 , 4 , 40. [ Google Scholar ] [ CrossRef ]
- Abdulhameed, O.; Al-Ahmari, A.; Ameen, W.; Mian, S.H. Additive Manufacturing: Challenges, Trends, and Applications. Adv. Mech. Eng. 2019 , 11 , 1–27. [ Google Scholar ] [ CrossRef ]
- Singh, R.; Gupta, A.; Tripathi, O.; Srivastava, S.; Singh, B.; Awasthi, A.; Rajput, S.K.; Sonia, P.; Singhal, P.; Saxena, K.K. Powder Bed Fusion Process in Additive Manufacturing: An Overview. Mater. Today Proc. 2019 , 26 , 3058–3070. [ Google Scholar ] [ CrossRef ]
- Ligon, S.C.; Liska, R.; Stampfl, J.; Gurr, M.; Mülhaupt, R. Polymers for 3D Printing and Customized Additive Manufacturing. Chem. Rev. 2017 , 117 , 10212–10290. [ Google Scholar ] [ CrossRef ]
- Herzog, D.; Seyda, V.; Wycisk, E.; Emmelmann, C. Additive Manufacturing of Metals. Acta Mater. 2016 , 117 , 371–392. [ Google Scholar ] [ CrossRef ]
- Bhatia, A.; Sehgal, A.K. Additive Manufacturing Materials, Methods and Applications: A Review. Mater. Today Proc. 2021 , 81 , 1060–1067. [ Google Scholar ] [ CrossRef ]
- Pratheesh Kumar, S.; Elangovan, S.; Mohanraj, R.; Ramakrishna, J.R. Review on the Evolution and Technology of State-of-the-Art Metal Additive Manufacturing Processes. Mater. Today Proc. 2021 , 46 , 7907–7920. [ Google Scholar ] [ CrossRef ]
- Wang, Y.; Zhou, Y.; Lin, L.; Corker, J.; Fan, M. Overview of 3D Additive Manufacturing (AM) and Corresponding AM Composites. Compos. Part A Appl. Sci. Manuf. 2020 , 139 , 106114. [ Google Scholar ] [ CrossRef ]
- Fijoł, N.; Aguilar-Sánchez, A.; Mathew, A.P. 3D-Printable Biopolymer-Based Materials for Water Treatment: A Review. Chem. Eng. J. 2022 , 430 , 132964. [ Google Scholar ] [ CrossRef ]
- Jiang, J.; Xu, X.; Stringer, J. Support Structures for Additive Manufacturing: A Review. J. Manuf. Mater. Process. 2018 , 2 , 64. [ Google Scholar ] [ CrossRef ]
- Karar, G.C.; Kumar, R.; Chattopadhyaya, S. An Analysis on the Advanced Research in Additive Manufacturing. In Advances in Production and Industrial Engineering. Part of the Lecture Notes in Mechanical Engineering Book Series ; Springer: Singapore, 2021; pp. 229–277. ISBN 9789811555183. [ Google Scholar ]
- ISO/ASTM 52900 ; Additive Manufacturing—General Principles—Terminology. ASTM—American Society for Testing and Materials: West Conshohocken, PA, USA, 2015.
- Durai Murugan, P.; Vijayananth, S.; Natarajan, M.P.; Jayabalakrishnan, D.; Arul, K.; Jayaseelan, V.; Elanchezhian, J. A Current State of Metal Additive Manufacturing Methods: A Review. Mater. Today Proc. 2021 , 59 , 1277–1283. [ Google Scholar ] [ CrossRef ]
- Shrinivas Mahale, R.; Shamanth, V.; Hemanth, K.; Nithin, S.K.; Sharath, P.C.; Shashanka, R.; Patil, A.; Shetty, D. Processes and Applications of Metal Additive Manufacturing. Mater. Today Proc. 2021 , 54 , 228–233. [ Google Scholar ] [ CrossRef ]
- Badoniya, P.; Srivastava, M.; Jain, P.K.; Rathee, S. A State-of-the-Art Review on Metal Additive Manufacturing: Milestones, Trends, Challenges and Perspectives ; Springer: Berlin/Heidelberg, Germany, 2024; Volume 46, ISBN 0123456789. [ Google Scholar ]
- Lee, J.Y.; An, J.; Chua, C.K. Fundamentals and Applications of 3D Printing for Novel Materials. Appl. Mater. Today 2017 , 7 , 120–133. [ Google Scholar ] [ CrossRef ]
- Ngo, T.D.; Kashani, A.; Imbalzano, G.; Nguyen, K.T.Q.; Hui, D. Additive Manufacturing (3D Printing): A Review of Materials, Methods, Applications and Challenges. Compos. Part B Eng. 2018 , 143 , 172–196. [ Google Scholar ] [ CrossRef ]
- Wang, J.C.; Dommati, H.; Hsieh, S.J. Review of Additive Manufacturing Methods for High-Performance Ceramic Materials. Int. J. Adv. Manuf. Technol. 2019 , 103 , 2627–2647. [ Google Scholar ] [ CrossRef ]
- Wang, Y.; Chen, R.; Liu, Y. A Double Mask Projection Exposure Method for Stereolithography. Sens. Actuators A Phys. 2020 , 314 , 112228. [ Google Scholar ] [ CrossRef ]
- Mazzoli, A. Selective Laser Sintering in Biomedical Engineering. Med. Biol. Eng. Comput. 2013 , 51 , 245–256. [ Google Scholar ] [ CrossRef ]
- Redwood, B.; Schoffer, F.; Garret, B. The 3D Printing Handbook. Technologies, Design and Applications ; 3D Hubs B.V.: Amsterdam, The Netherlands, 2020. [ Google Scholar ]
- Giri, J.; Sunheriya, N.; Sathish, T.; Kadu, Y.; Chadge, R.; Giri, P.; Parthiban, A.; Mahatme, C. Optimization of Process Parameters to Improve Mechanical Properties of Fused Deposition Method Using Taguchi Method. Interactions 2024 , 245 , 87. [ Google Scholar ] [ CrossRef ]
- Alafaghani, A.; Qattawi, A.; Alrawi, B.; Guzman, A. Experimental Optimization of Fused Deposition Modelling Processing Parameters: A Design-for-Manufacturing Approach. Procedia Manuf. 2017 , 10 , 791–803. [ Google Scholar ] [ CrossRef ]
- Hafsa, M.N.; Kassim, N.; Ismail, S.; Kamaruddin, S.A.; Hafeez, T.M.; Ibrahim, M.; Samsudin, Z.H. Study on Surface Roughness Quality of FDM and MJM Additive Manufacturing Model for Implementation as Investment Casting Sacrificial Pattern. J. Mech. Eng. 2018 , 5 , 25–34. [ Google Scholar ]
- Emiliani, N.; Porcaro, R.; Pisaneschi, G.; Bortolani, B.; Ferretti, F.; Fontana, F.; Campana, G.; Fiorini, M.; Marcelli, E.; Cercenelli, L. Post-Printing Processing and Aging Effects on Polyjet Materials Intended for the Fabrication of Advanced Surgical Simulators. J. Mech. Behav. Biomed. Mater. 2024 , 156 , 106598. [ Google Scholar ] [ CrossRef ]
- Yang, Y.; Bharech, S.; Finger, N.; Zhou, X.; Schröder, J.; Xu, B.X. Elasto-Plastic Residual Stress Analysis of Selective Laser Sintered Porous Materials Based on 3D-Multilayer Thermo-Structural Phase-Field Simulations. npj Comput. Mater. 2024 , 10 , 117. [ Google Scholar ] [ CrossRef ]
- Rajesh, R.; Sudheer, S.; Kulkarni, M.V. Selective Laser Sintering Process—A Review. Int. J. Curr. Eng. Sci. Res. (IJCESR) 2015 , 2 , 91–100. [ Google Scholar ]
- Paolini, A.; Kollmannsberger, S.; Rank, E. Additive Manufacturing in Construction: A Review on Processes, Applications, and Digital Planning Methods. Addit. Manuf. 2019 , 30 , 100894. [ Google Scholar ] [ CrossRef ]
- Volpe, S.; Sangiorgio, V.; Fiorito, F.; Varum, H. Overview of 3D Construction Printing and Future Perspectives: A Review of Technology, Companies and Research Progression. Archit. Sci. Rev. 2022 , 67 , 1–22. [ Google Scholar ] [ CrossRef ]
- Placzek, G.; Schwerdtner, P. Concrete Additive Manufacturing in Construction: Integration Based on Component-Related Fabrication Strategies. Buildings 2023 , 13 , 1769. [ Google Scholar ] [ CrossRef ]
- Pacillo, G.A.; Ranocchiai, G.; Loccarini, F.; Fagone, M. Additive Manufacturing in Construction: A Review on Technologies, Processes, Materials, and Their Applications of 3D and 4D Printing. Mater. Des. Process. Commun. 2021 , 3 , e253. [ Google Scholar ] [ CrossRef ]
- Scheel, P.; Wrobel, R.; Rheingans, B.; Mayer, T.; Leinenbach, C.; Mazza, E.; Hosseini, E. Advancing Efficiency and Reliability in Thermal Analysis of Laser Powder-Bed Fusion. Int. J. Mech. Sci. 2023 , 260 , 108583. [ Google Scholar ] [ CrossRef ]
- Yap, C.Y.; Chua, C.K.; Dong, Z.L.; Liu, Z.H.; Zhang, D.Q.; Loh, L.E.; Sing, S.L. Review of Selective Laser Melting: Materials and Applications. Appl. Phys. Rev. 2015 , 2 , 041101. [ Google Scholar ] [ CrossRef ]
- Song, B.; Zhao, X.; Li, S.; Han, C.; Wei, Q.; Wen, S.; Liu, J.; Shi, Y. Differences in Microstructure and Properties between Selective Laser Melting and Traditional Manufacturing for Fabrication of Metal Parts: A Review. Front. Mech. Eng. 2015 , 10 , 111–125. [ Google Scholar ] [ CrossRef ]
- Du, X.; Chen, J.; She, Y.; Liu, Y.; Yang, Y.; Yang, J.; Dong, S. Effect of Process Parameter Optimization on Morphology and Mechanical Properties of Ti 6 Al 4 V Alloy Produced by Selective Laser Melting. Prog. Nat. Sci. Mater. Int. 2023 , 33 , 911–917. [ Google Scholar ] [ CrossRef ]
- Fette, M.; Sander, P.; Wulfsberg, J.; Zierk, H.; Herrmann, A.; Stoess, N. Optimized and Cost-Efficient Compression Molds Manufactured by Selective Laser Melting for the Production of Thermoset Fiber Reinforced Plastic Aircraft Components. Procedia CIRP 2015 , 35 , 25–30. [ Google Scholar ] [ CrossRef ]
- Liu, B.; Bai, P.; Li, Y. Post Treatment Process and Selective Laser Sintering Mechanism of Polymer-Coated Mo Powder. Open Mater. Sci. J. 2011 , 5 , 194–198. [ Google Scholar ] [ CrossRef ]
- Andreacola, F.R.; Capasso, I.; Pilotti, L.; Brando, G. Influence of 3D-Printing Parameters on the Mechanical Properties of 17-4PH Stainless Steel Produced through Selective Laser Melting. Frat. Intregrità Strutt. 2021 , 58 , 282–295. [ Google Scholar ] [ CrossRef ]
- Andreacola, F.R.; Capasso, I.; Langella, A.; Brando, G. 3D-Printed Metals: Process Parameters Effects on Mechanical Properties of 17-4PH Stainless Steel. Heliyon 2023 , 9 , e17698. [ Google Scholar ] [ CrossRef ]
- Gokuldoss, P.K.; Kolla, S.; Eckert, J. Additive Manufacturing Processes: Selective Laser Melting, Electron Beam Melting and Binder Jetting-Selection Guidelines. Materials 2017 , 10 , 672. [ Google Scholar ] [ CrossRef ]
- Murr, L.E.; Gaytan, S.M.; Ramirez, D.A.; Martinez, E.; Hernandez, J.; Amato, K.N.; Shindo, P.W.; Medina, F.R.; Wicker, R.B. Metal Fabrication by Additive Manufacturing Using Laser and Electron Beam Melting Technologies. J. Mater. Sci. Technol. 2012 , 28 , 167–177. [ Google Scholar ] [ CrossRef ]
- Huang, N.; Cook, O.J.; Argüelles, A.P.; Beese, A.M. Review of Process–Structure–Property Relationships in Metals Fabricated Using Binder Jet Additive Manufacturing. Metallogr. Microstruct. Anal. 2023 , 12 , 883–905. [ Google Scholar ] [ CrossRef ]
- Tischel, F.; Reineke, L.; Alrashdan, J.; Ploshikhin, V. Experimental Investigation and Modeling of Densification during Sintering of Binder Jetted Ti–6Al–4V. Powder Technol. 2024 , 444 , 119958. [ Google Scholar ] [ CrossRef ]
- Aramian, A.; Razavi, S.M.J.; Sadeghian, Z.; Berto, F. A Review of Additive Manufacturing of Cermets. Addit. Manuf. 2020 , 33 , 101130. [ Google Scholar ] [ CrossRef ]
- Mostafaei, A.; Elliott, A.M.; Barnes, J.E.; Li, F.; Tan, W.; Cramer, C.L.; Nandwana, P.; Chmielus, M. Binder Jet 3D Printing-Process Parameters, Materials, Properties, Modeling, and Challenges. Prog. Mater. Sci. 2021 , 119 , 100707. [ Google Scholar ] [ CrossRef ]
- Blunk, H.; Seibel, A. Design Guidelines for Metal Binder Jetting. Prog. Addit. Manuf. 2024 , 9 , 725–732. [ Google Scholar ] [ CrossRef ]
- Dwivedi, S.; Dixit, A.R.; Das, A.K.; Nag, A. A Novel Additive Texturing of Stainless Steel 316L Through Binder Jetting Additive Manufacturing. Int. J. Precis. Eng. Manuf. Green Technol. 2023 , 10 , 1605–1613. [ Google Scholar ] [ CrossRef ]
- Mueller, B.; Kochan, D. Laminated Object Manufacturing for Rapid Tooling and Patternmaking in Foundry Industry. Comput. Ind. 1999 , 39 , 47–53. [ Google Scholar ] [ CrossRef ]
- Kan, C.; Zhao, L.; Cao, Y.; Ma, C.; Peng, Y.; Tian, Z. Microstructure Evolution and Strengthening Behavior of Maraging Steel Fabricated by Wire Arc Additive Manufacturing at Different Heat Treatment Processes. Mater. Sci. Eng. A 2024 , 909 , 146804. [ Google Scholar ] [ CrossRef ]
- Srivastava, M.; Rathee, S.; Tiwari, A.; Dongre, M. Wire Arc Additive Manufacturing of Metals: A Review on Processes, Materials and Their Behaviour. Mater. Chem. Phys. 2023 , 294 , 126988. [ Google Scholar ] [ CrossRef ]
- Ahmed, N. Direct Metal Fabrication in Rapid Prototyping: A Review. J. Manuf. Process. 2019 , 42 , 167–191. [ Google Scholar ] [ CrossRef ]
- Alami, A.H.; Ghani Olabi, A.; Alashkar, A.; Alasad, S.; Aljaghoub, H.; Rezk, H.; Abdelkareem, M.A. Additive Manufacturing in the Aerospace and Automotive Industries: Recent Trends and Role in Achieving Sustainable Development Goals. Ain Shams Eng. J. 2023 , 14 , 102516. [ Google Scholar ] [ CrossRef ]
- Arrizubieta, J.I.; Martínez, S.; Lamikiz, A.; Ukar, E.; Arntz, K.; Klocke, F. Instantaneous Powder Flux Regulation System for Laser Metal Deposition. J. Manuf. Process. 2017 , 29 , 242–251. [ Google Scholar ] [ CrossRef ]
- Pirch, N.; Linnenbrink, S.; Gasser, A.; Schleifenbaum, H. Laser-Aided Directed Energy Deposition of Metal Powder along Edges. Int. J. Heat Mass Transf. 2019 , 143 , 118464. [ Google Scholar ] [ CrossRef ]
- Yilmaz, O.; Ugla, A.A. Shaped Metal Deposition Technique in Additive Manufacturing: A Review. Proc. Inst. Mech. Eng. Part B J. Eng. Manuf. 2016 , 230 , 1781–1798. [ Google Scholar ] [ CrossRef ]
- Agelet de Saracibar, C.; Lundbäck, A.; Chiumenti, M.; Cervera, M. Shaped Metal Deposition Processes. In Encyclopedia of Thermal Stresses ; Springer: Dordrecht, The Netherlands, 2014; pp. 4346–4355. [ Google Scholar ] [ CrossRef ]
- Cooke, S.; Ahmadi, K.; Willerth, S.; Herring, R. Metal Additive Manufacturing: Technology, Metallurgy and Modelling. J. Manuf. Process. 2020 , 57 , 978–1003. [ Google Scholar ] [ CrossRef ]
- Zhang, D.; Sun, S.; Qiu, D.; Gibson, M.A.; Dargusch, M.S.; Brandt, M.; Qian, M.; Easton, M. Metal Alloys for Fusion-Based Additive Manufacturing. Adv. Eng. Mater. 2018 , 20 , 1700952. [ Google Scholar ] [ CrossRef ]
- Li, Y.; Liang, X.; Yu, Y.; Wang, D.; Lin, F. Review on Additive Manufacturing of Single-Crystal Nickel-Based Superalloys. Chin. J. Mech. Eng. Addit. Manuf. Front. 2022 , 1 , 100019. [ Google Scholar ] [ CrossRef ]
- Mostafaei, A.; Ghiaasiaan, R.; Ho, I.T.; Strayer, S.; Chang, K.C.; Shamsaei, N.; Shao, S.; Paul, S.; Yeh, A.C.; Tin, S.; et al. Additive Manufacturing of Nickel-Based Superalloys: A State-of-the-Art Review on Process-Structure-Defect-Property Relationship. Prog. Mater. Sci. 2023 , 136 , 101108. [ Google Scholar ] [ CrossRef ]
- Mazzucato, F.; Forni, D.; Valente, A.; Cadoni, E. Laser Metal Deposition of Inconel 718 Alloy and As-Built Mechanical Properties Compared to Casting. Materials 2021 , 14 , 437. [ Google Scholar ] [ CrossRef ]
- Dixit, S.; Liu, S. Laser Additive Manufacturing of High-Strength Aluminum Alloys: Challenges and Strategies. J. Manuf. Mater. Process. 2022 , 6 , 156. [ Google Scholar ] [ CrossRef ]
- Rometsch, P.A.; Zhu, Y.; Wu, X.; Huang, A. Review of High-Strength Aluminium Alloys for Additive Manufacturing by Laser Powder Bed Fusion. Mater. Des. 2022 , 219 , 110779. [ Google Scholar ] [ CrossRef ]
- Farber, E.; Zhu, J.N.; Popovich, A.; Popovich, V. A Review of NiTi Shape Memory Alloy as a Smart Material Produced by Additive Manufacturing. Mater. Today Proc. 2019 , 30 , 761–767. [ Google Scholar ] [ CrossRef ]
- Felice, I.O.; Shen, J.; Barragan, A.F.C.; Moura, I.A.B.; Li, B.; Wang, B.; Khodaverdi, H.; Mohri, M.; Schell, N.; Ghafoori, E.; et al. Wire and Arc Additive Manufacturing of Fe-Based Shape Memory Alloys: Microstructure, Mechanical and Functional Behavior. Mater. Des. 2023 , 231 , 112004. [ Google Scholar ] [ CrossRef ]
- Wei, S.; Zhang, J.; Zhang, L.; Zhang, Y.; Song, B.; Wang, X.; Fan, J.; Liu, Q.; Shi, Y. Laser Powder Bed Fusion Additive Manufacturing of NiTi Shape Memory Alloys: A Review. Int. J. Extrem. Manuf. 2023 , 5 , 032001. [ Google Scholar ] [ CrossRef ]
- Ostovari Moghaddam, A.; Shaburova, N.A.; Samodurova, M.N.; Abdollahzadeh, A.; Trofimov, E.A. Additive Manufacturing of High Entropy Alloys: A Practical Review. J. Mater. Sci. Technol. 2021 , 77 , 131–162. [ Google Scholar ] [ CrossRef ]
- Ron, T.; Shirizly, A.; Aghion, E. Additive Manufacturing Technologies of High Entropy Alloys (HEA): Review and Prospects. Materials 2023 , 16 , 2454. [ Google Scholar ] [ CrossRef ]
- Cao, L.; Li, J.; Hu, J.; Liu, H.; Wu, Y.; Zhou, Q. Optimization of Surface Roughness and Dimensional Accuracy in LPBF Additive Manufacturing. Opt. Laser Technol. 2021 , 142 , 107246. [ Google Scholar ] [ CrossRef ]
- Nandhakumar, R.; Venkatesan, K. A Process Parameters Review on Selective Laser Melting-Based Additive Manufacturing of Single and Multi-Material: Microstructure, Physical Properties, Tribological, and Surface Roughness ; Elsevier Ltd.: Amsterdam, The Netherlands, 2023; Volume 35, ISBN 5465151392629. [ Google Scholar ]
- Xia, C.; Pan, Z.; Polden, J.; Li, H.; Xu, Y.; Chen, S. Modelling and Prediction of Surface Roughness in Wire Arc Additive Manufacturing Using Machine Learning. J. Intell. Manuf. 2022 , 33 , 1467–1482. [ Google Scholar ] [ CrossRef ]
- Obilanade, D.; Dordlofva, C.; Törlind, P. Surface Roughness Considerations in Design for Additive Manufacturing—A Literature Review. Proc. Des. Soc. 2021 , 1 , 2841–2850. [ Google Scholar ] [ CrossRef ]
- Zai, L.; Zhang, C.; Wang, Y.; Guo, W.; Wellmann, D.; Tong, X.; Tian, Y. Laser Powder Bed Fusion of Precipitation-Hardened Martensitic Stainless Steels: A Review. Metals 2020 , 10 , 255. [ Google Scholar ] [ CrossRef ]
- Sefene, E.M. State-of-the-Art of Selective Laser Melting Process: A Comprehensive Review. J. Manuf. Syst. 2022 , 63 , 250–274. [ Google Scholar ] [ CrossRef ]
- Gardner, L.; Kyvelou, P.; Herbert, G.; Buchanan, C. Testing and Initial Verification of the World’s First Metal 3D Printed Bridge. J. Constr. Steel Res. 2020 , 172 , 106233. [ Google Scholar ] [ CrossRef ]
- Anant Pidge, P.; Kumar, H. Additive Manufacturing: A Review on 3 D Printing of Metals and Study of Residual Stress, Buckling Load Capacity of Strut Members. Mater. Today Proc. 2020 , 21 , 1689–1694. [ Google Scholar ] [ CrossRef ]
- Tan, J.H.; Wong, W.L.E.; Dalgarno, K.W. An Overview of Powder Granulometry on Feedstock and Part Performance in the Selective Laser Melting Process. Addit. Manuf. 2017 , 18 , 228–255. [ Google Scholar ] [ CrossRef ]
- Larimian, T.; Kannan, M.; Grzesiak, D.; AlMangour, B.; Borkar, T. Effect of Energy Density and Scanning Strategy on Densification, Microstructure and Mechanical Properties of 316L Stainless Steel Processed via Selective Laser Melting. Mater. Sci. Eng. A 2020 , 770 , 138455. [ Google Scholar ] [ CrossRef ]
- Chen, J.; Wang, X.; Pan, Y. Influence of Laser Power and Scan Speed on the Microstructure and Properties of GH4169 Alloy Prepared by Selective Laser Melting. IOP Conf. Series Mater. Sci. Eng. 2019 , 688 , 033064. [ Google Scholar ] [ CrossRef ]
- Bremen, S.; Meiners, W.; Diatlov, A. Selective Laser Melting: A Manufacturing Technology for the Future? Laser Tech J 2012 , 9 , 33–38. [ Google Scholar ] [ CrossRef ]
- Mercelis, P.; Kruth, J.P. Residual Stresses in Selective Laser Sintering and Selective Laser Melting. Rapid Prototyp. J 2006 , 12 , 254–265. [ Google Scholar ] [ CrossRef ]
- Zhang, W.; Tong, M.; Harrison, N.M. Scanning Strategies Effect on Temperature, Residual Stress and Deformation by Multi-Laser Beam Powder Bed Fusion Manufacturing. Addit. Manuf. 2020 , 36 , 101507. [ Google Scholar ] [ CrossRef ]
- Miao, X.; Liu, X.; Lu, P.; Han, J.; Duan, W.; Wu, M. Influence of Scanning Strategy on the Performances of GO-Reinforced Ti 6 Al 4 V Nanocomposites Manufactured by SLM. Metals 2020 , 10 , 1379. [ Google Scholar ] [ CrossRef ]
- Haferkamp, L.; Haudenschild, L.; Spierings, A.; Wegener, K.; Riener, K.; Ziegelmeier, S.; Leichtfried, G.J. The Influence of Particle Shape, Powder Flowability, and Powder Layer Density on Part Density in Laser Powder Bed Fusion. Metals 2021 , 11 , 418. [ Google Scholar ] [ CrossRef ]
- Spierings, A.B.; Herres, N.; Levy, G. Influence of the Particle Size Distribution on Surface Quality and Mechanical Properties in Additive Manufactured Stainless Steel Parts. Rapid Prototyp. J. 2010 , 17 , 195–202. [ Google Scholar ] [ CrossRef ]
- Huck-Jones, D.; Langley, C. Beyond Particle Size: Exploring the Influence of Particle Shape on Metal Powder Performance. Met. Addit. Manuf. 2017 , 3 , 99–103. [ Google Scholar ]
- Eddine, S.; Letenneur, M.; Alex, C.; Brailovski, V. Influence of Particle Morphology and Size Distribution on the Powder Flowability and Laser Powder Bed Fusion Manufacturability of Ti-6Al-4V Alloy. Addit. Manuf. 2020 , 31 , 100929. [ Google Scholar ] [ CrossRef ]
- Irrinki, H.; Dexter, M.; Barmore, B.; Enneti, R.; Pasebani, S.; Badwe, S.; Stitzel, J.; Malhotra, R.; Atre, S.V. Effects of Powder Attributes and Laser Powder Bed Fusion (L-PBF) Process Conditions on the Densification and Mechanical Properties of 17-4 PH Stainless Steel. JOM J. Miner. Met. Mater. Soc. 2016 , 68 , 860–868. [ Google Scholar ] [ CrossRef ]
- Rashid, R.; Masood, S.H.; Ruan, D.; Palanisamy, S.; Rahman Rashid, R.A.; Brandt, M. Effect of Scan Strategy on Density and Metallurgical Properties of 17-4PH Parts Printed by Selective Laser Melting (SLM). J. Mater. Process. Technol. 2017 , 249 , 502–511. [ Google Scholar ] [ CrossRef ]
- Nguyen, Q.B.; Luu, D.N.; Nai, S.M.L.; Zhu, Z.; Chen, Z.; Wei, J. The Role of Powder Layer Thickness on the Quality of SLM Printed Parts. Arch. Civ. Mech. Eng. 2018 , 18 , 948–955. [ Google Scholar ] [ CrossRef ]
- Haghdadi, N.; Laleh, M.; Moyle, M.; Primig, S. Additive Manufacturing of Steels: A Review of Achievements and Challenges. J. Mater. Sci. 2021 , 56 , 64–107. [ Google Scholar ] [ CrossRef ]
- Simonelli, M.; Tse, Y.Y.; Tuck, C. Effect of the Build Orientation on the Mechanical Properties and Fracture Modes of SLM Ti–6Al–4V. Mater. Sci. Eng. A 2014 , 616 , 1–11. [ Google Scholar ] [ CrossRef ]
- Guan, K.; Wang, Z.; Gao, M.; Li, X.; Zeng, X. Effects of Processing Parameters on Tensile Properties of Selective Laser Melted 304 Stainless Steel. Mater. Des. 2013 , 50 , 581–586. [ Google Scholar ] [ CrossRef ]
- How Does Part Orientation Affect a 3D Print? Practical Design Tips for Additive Manufacturing. 2024. Available online: https://www.hubs.com/knowledge-base/how-does-part-orientation-affect-3d-print/ (accessed on 7 June 2024).
- Yadollahi, A.; Shamsaei, N.; Thompson, S.M.; Elwany, A.; Bian, L. Effects of Building Orientation and Heat Treatment on Fatigue Behavior of Selective Laser Melted 17-4 PH Stainless Steel. Int. J. Fatigue 2017 , 94 , 218–235. [ Google Scholar ] [ CrossRef ]
- Hitzler, L.; Janousch, C.; Schanz, J.; Merkel, M.; Heine, B.; Mack, F.; Hall, W.; Öchsner, A. Direction and Location Dependency of Selective Laser Melted AlSi10Mg Specimens. J. Mater. Process. Technol. 2017 , 243 , 48–61. [ Google Scholar ] [ CrossRef ]
- Wang, C.G.; Zhu, J.X.; Wang, G.W.; Qin, Y.; Sun, M.Y.; Yang, J.L.; Shen, X.F.; Huang, S.K. Effect of Building Orientation and Heat Treatment on the Anisotropic Tensile Properties of AlSi 10 Mg Fabricated by Selective Laser Melting. J. Alloys Compd. 2022 , 895 , 162665. [ Google Scholar ] [ CrossRef ]
- Sufiiarov, V.S.; Popovich, A.A.; Borisov, E.V.; Polozov, I.A.; Masaylo, D.V.; Orlov, A.V. The Effect of Layer Thickness at Selective Laser Melting. Procedia Eng. 2017 , 174 , 126–134. [ Google Scholar ] [ CrossRef ]
- Wan, H.Y.; Zhou, Z.J.; Li, C.P.; Chen, G.F.; Zhang, G.P. Effect of Scanning Strategy on Mechanical Properties of Selective Laser Melted Inconel 718. Mater. Sci. Eng. A 2019 , 753 , 42–48. [ Google Scholar ] [ CrossRef ]
- Giganto, S.; Zapico, P.; Castro-Sastre, M.Á.; Martínez-Pellitero, S.; Leo, P.; Perulli, P. Influence of the Scanning Strategy Parameters upon the Quality of the SLM Parts. Procedia Manuf. 2019 , 41 , 698–705. [ Google Scholar ] [ CrossRef ]
- Forni, D.; Mazzucato, F.; Valente, A.; Cadoni, E. High Strain-Rate Behaviour of as-Cast and as-Build Inconel 718 Alloys at Elevated Temperatures. Mech. Mater. 2021 , 159 , 103859. [ Google Scholar ] [ CrossRef ]
- Brando, G.; Andreacola, F.R.; Capasso, I.; Forni, D.; Cadoni, E. Strain-Rate Response of 3D Printed 17-4PH Stainless Steel Manufactured via Selective Laser Melting. Constr. Build. Mater. 2023 , 409 , 133971. [ Google Scholar ] [ CrossRef ]
- Michla, J.R.J.; Nagarajan, R.; Krishnasamy, S.; Siengchin, S.; Ismail, S.O.; Prabhu, T.R. Conventional and Additively Manufactured Stainless Steels: A Review. Trans. Indian Inst. Met. 2021 , 74 , 1261–1278. [ Google Scholar ] [ CrossRef ]
- Zhu, H.H.; Lu, L.; Fuh, J.Y.H. Study on Shrinkage Behaviour of Direct Laser Sintering Metallic Powder. Proc. Inst. Mech. Eng. B J. Eng. Manuf. 2006 , 220 , 183–190. [ Google Scholar ] [ CrossRef ]
- Ramos, D.; Belblidia, F.; Sienz, J. New Scanning Strategy to Reduce Warpage in Additive Manufacturing. Addit. Manuf. 2019 , 28 , 554–564. [ Google Scholar ] [ CrossRef ]
- Enneti, R.K.; Morgan, R.; Atre, S.V. Effect of Process Parameters on the Selective Laser Melting (SLM) of Tungsten. Int. J. Refract. Met. Hard. Mater. 2018 , 71 , 315–319. [ Google Scholar ] [ CrossRef ]
- Klocke, F.; Wagner, C. Coalescence Behaviour of Two Metallic Particles as Base Mechanism of Selective Laser Sintering. CIRP Ann. 2003 , 52 , 117–180. [ Google Scholar ] [ CrossRef ]
- Simchi, A.; Pohl, H. Effects of Laser Sintering Processing Parameters on the Microstructure and Densification of Iron Powder. Mater. Sci. Eng. A 2003 , 359 , 119–128. [ Google Scholar ] [ CrossRef ]
- Attaran, M. The Rise of 3-D Printing: The Advantages of Additive Manufacturing over Traditional Manufacturing. Bus. Horiz. 2017 , 60 , 677–688. [ Google Scholar ] [ CrossRef ]
- Ali, M.H.; Issayev, G.; Shehab, E.; Sarfraz, S. A Critical Review of 3D Printing and Digital Manufacturing in Construction Engineering. Rapid Prototyp. J. 2022 , 28 , 1312–1324. [ Google Scholar ] [ CrossRef ]
- Hossain, M.A.; Zhumabekova, A.; Paul, S.C.; Kim, J.R. A Review of 3D Printing in Construction and Its Impact on the Labor Market. Sustainability 2020 , 12 , 8492. [ Google Scholar ] [ CrossRef ]
- Buchanan, C.; Gardner, L. Metal 3D Printing in Construction: A Review of Methods, Research, Applications, Opportunities and Challenges. Eng. Struct. 2019 , 180 , 332–348. [ Google Scholar ] [ CrossRef ]
- Riegger, F.; Wenzler, D.L.; Zaeh, M.F. Stud and Wire Arc Additive Manufacturing—Development of a Combined Process for the High-Productivity Additive Manufacturing of Large-Scale Lattice Structures. J. Adv. Join. Process. 2024 , 9 , 100189. [ Google Scholar ] [ CrossRef ]
- Ren, S.; Galjaard, S. ; Arup Topology Optimisation for Steel Structural Design with Additive Manufacturing. Modelling Behaviour ; Springer: Cham, Swizterland, 2015. [ Google Scholar ] [ CrossRef ]
- Galjaard, S.; Hofman, S.; Ren, S. Optimizing Structural Building Elements in Metal by Using Additive Manufacturing. Proc. Int. Assoc. Shell Spat. Struct. 2015 , 2 , 1–12. [ Google Scholar ]
- Galjaard, S.; Hofman, S.; Ren, S. New Opportunities to Optimize Structural Designs in Metal by Using Additive Manufacturing. In Advances in Architectural Geometry 2014 ; Springer: Cham, Swizterland, 2015. [ Google Scholar ] [ CrossRef ]
- Gardner, L. Metal Additive Manufacturing in Structural Engineering—Review, Advances, Opportunities and Outlook. Structures 2023 , 47 , 2178–2193. [ Google Scholar ] [ CrossRef ]
- MX3D Bridge. 2024. Available online: https://mx3d.com/industries/mx3d-bridge/ (accessed on 5 July 2024).
- Connector for Takenaka. Available online: https://mx3d.com/projects/takenaka-connector/ (accessed on 5 July 2024).
- Mechtcherine, V.; Grafe, J.; Nerella, V.N.; Spaniol, E.; Hertel, M.; Füssel, U. 3D-Printed Steel Reinforcement for Digital Concrete Construction—Manufacture, Mechanical Properties and Bond Behaviour. Constr. Build. Mater. 2018 , 179 , 125–137. [ Google Scholar ] [ CrossRef ]
- Müller, J.; Grabowski, M.; Müller, C.; Hensel, J.; Unglaub, J.; Thiele, K.; Kloft, H.; Dilger, K. Design and Parameter Identification of Wire and Arc Additively Manufactured (WAAM) Steel Bars for Use in Construction. Metals 2019 , 9 , 725. [ Google Scholar ] [ CrossRef ]
- Silvestru, V.A.; Ariza, I.; Taras, A. Structural Behaviour of Point-by-Point Wire Arc Additively Manufactured Steel Bars under Compressive Loading. J. Constr. Steel Res. 2023 , 207 , 107982. [ Google Scholar ] [ CrossRef ]
- Silvestru, V.A.; Ariza, I.; Vienne, J.; Michel, L.; Aguilar Sanchez, A.M.; Angst, U.; Rust, R.; Gramazio, F.; Kohler, M.; Taras, A. Performance under Tensile Loading of Point-by-Point Wire and Arc Additively Manufactured Steel Bars for Structural Components. Mater. Des. 2021 , 205 , 109740. [ Google Scholar ] [ CrossRef ]
- Aboulkhair, N.T.; Simonelli, M.; Parry, L.; Ashcroft, I.; Tuck, C.; Hague, R. 3D Printing of Aluminium Alloys: Additive Manufacturing of Aluminium Alloys Using Selective Laser Melting. Prog. Mater. Sci. 2019 , 106 , 100578. [ Google Scholar ] [ CrossRef ]
- Seabra, M.; Azevedo, J.; Araújo, A.; Reis, L.; Pinto, E.; Alves, N.; Santos, R.; Pedro Mortágua, J. Selective Laser Melting (SLM) and Topology Optimization for Lighter Aerospace Componentes. Procedia Struct. Integr. 2016 , 1 , 289–296. [ Google Scholar ] [ CrossRef ]
- Dimitrov, D.; Uheida, E.; Oosthuizen, G.; Blaine, D.; Laubscher, R.; Sterzing, A.; Blau, P.; Gerber, W.; Damm, O.F.R.A. Manufacturing of High Added Value Titanium Components. A South African Perspective. IOP Conf. Ser. Mater. Sci. Eng. 2018 , 430 , 012009. [ Google Scholar ] [ CrossRef ]
- Saadlaoui, Y.; Milan, J.L.; Rossi, J.M.; Chabrand, P. Topology Optimization and Additive Manufacturing: Comparison of Conception Methods Using Industrial Codes. J. Manuf. Syst. 2017 , 43 , 178–186. [ Google Scholar ] [ CrossRef ]
- Baptista, R.J.S.; Pragana, J.P.M.; Bragança, I.M.F.; Silva, C.M.A.; Alves, L.M.; Martins, P.A.F. Joining Aluminium Profiles to Composite Sheets by Additive Manufacturing and Forming. J. Mater. Process. Technol. 2020 , 279 , 116587. [ Google Scholar ] [ CrossRef ]
- Guo, X.; Kyvelou, P.; Ye, J.; Teh, L.H.; Gardner, L. Experimental Study of DED-Arc Additively Manufactured Steel Double-Lap Shear Bolted Connections. Eng. Struct. 2023 , 281 , 115736. [ Google Scholar ] [ CrossRef ]
- Meng, X.; Zhi, J.; Xu, F.; Gardner, L. Novel Hybrid Sleeve Connections between 3D Printed and Conventional Tubular Steel Elements. Eng. Struct. 2024 , 302 , 117269. [ Google Scholar ] [ CrossRef ]
- Guo, X.; Kyvelou, P.; Ye, J.; Gardner, L. Experimental Investigation of Wire Arc Additively Manufactured Steel T-Stub Connections. J. Constr. Steel Res. 2023 , 211 , 108106. [ Google Scholar ] [ CrossRef ]
- Feucht, T.; Lange, J. 3-D-Printing with Steel: Additive Manufacturing of Connection Elements and Beam Reinforcements. ce/papers 2019 , 3 , 343–348. [ Google Scholar ] [ CrossRef ]
- Lange, J.; Feucht, T.; Erven, M. 3D Printing with Steel: Additive Manufacturing for Connections and Structures. Steel Constr. 2020 , 13 , 144–153. [ Google Scholar ] [ CrossRef ]
- Walton, D.; Moztarzadeh, H. Design and Development of an Additive Manufactured Component by Topology Optimisation. Procedia CIRP 2017 , 60 , 205–210. [ Google Scholar ] [ CrossRef ]
- Hällgren, S.; Pejryd, L.; Ekengren, J. (Re)Design for Additive Manufacturing. Procedia CIRP 2016 , 50 , 246–251. [ Google Scholar ] [ CrossRef ]
- Plocher, J.; Panesar, A. Review on Design and Structural Optimisation in Additive Manufacturing: Towards next-Generation Lightweight Structures. Mater. Des. 2019 , 183 , 108164. [ Google Scholar ] [ CrossRef ]
- Gebisa, A.W.; Lemu, H.G. A Case Study on Topology Optimized Design for Additive Manufacturing. IOP Conf. Ser. Mater. Sci. Eng. 2017 , 276 , 012026. [ Google Scholar ] [ CrossRef ]
- Tyflopoulos, E.; Flem, D.T.; Steinert, M.; Olsen, A. State of the Art of Generative Design and Topology Optimization and Potential Research Needs. In Proceedings of the NordDesign 2018, Linköping, Sweden, 14–17 August 2018. [ Google Scholar ]
- Christensen, P.; Klarbring, A. An Introduction to Structural Optimization ; Springer: Dordrecht, The Netherlands, 2008; Volume 153. [ Google Scholar ]
- Rozvany, G.I.N. A Critical Review of Established Methods of Structural Topology Optimization. Struct. Multidiscip. Optim. 2009 , 37 , 217–237. [ Google Scholar ] [ CrossRef ]
- Bendsøe, M.P.; Sigmund, O. Material Interpolation Schemes in Topology Optimization. Arch. Appl. Mech. 1999 , 69 , 635–654. [ Google Scholar ] [ CrossRef ]
- Allaire, G.; Jouve, F.; Toader, A.M. Structural Optimization Using Sensitivity Analysis and a Level-Set Method. J. Comput. Phys. 2004 , 194 , 363–393. [ Google Scholar ] [ CrossRef ]
- Wang, M.Y.; Wang, X.; Guo, D. A Level Set Method for Structural Topology Optimization. Comput. Methods Appl. Mech. Eng. 2003 , 192 , 227–246. [ Google Scholar ] [ CrossRef ]
- Maconachie, T.; Leary, M.; Lozanovski, B.; Zhang, X.; Qian, M.; Faruque, O.; Brandt, M. SLM Lattice Structures: Properties, Performance, Applications and Challenges. Mater. Des. 2019 , 183 , 108137. [ Google Scholar ] [ CrossRef ]
- Leary, M.; Mazur, M.; Williams, H.; Yang, E.; Alghamdi, A.; Lozanovski, B.; Zhang, X.; Shidid, D.; Farahbod-Sternahl, L.; Witt, G.; et al. Inconel 625 Lattice Structures Manufactured by Selective Laser Melting (SLM): Mechanical Properties, Deformation and Failure Modes. Mater. Des. 2018 , 157 , 179–199. [ Google Scholar ] [ CrossRef ]
- Panesar, A.; Abdi, M.; Hickman, D.; Ashcroft, I. Strategies for Functionally Graded Lattice Structures Derived Using Topology Optimisation for Additive Manufacturing. Addit. Manuf. 2018 , 19 , 81–94. [ Google Scholar ] [ CrossRef ]
- Liu, R.; Wang, Z.; Sparks, T.; Liou, F.; Newkirk, J. Aerospace Applications of Laser Additive Manufacturing. In Laser Additive Manufacturing: Materials, Design, Technologies, and Applications ; Woodhead Publishing: Sawston, UK, 2017; pp. 351–371. ISBN 9780081004333. [ Google Scholar ]
- Gao, J.; Folkes, J.; Yilmaz, O.; Gindy, N. Investigation of a 3D Non-Contact Measurement Based Blade Repair Integration System. Aircr. Eng. Aerosp. Technol. 2005 , 77 , 34–41. [ Google Scholar ] [ CrossRef ]
- Leino, M.; Pekkarinen, J.; Soukka, R. The Role of Laser Additive Manufacturing Methods of Metals in Repair, Refurbishment and Remanufacturing—Enabling Circular Economy. Phys. Procedia 2016 , 83 , 752–760. [ Google Scholar ] [ CrossRef ]
3D Printing Technique | Advantages | Disadvantages |
---|---|---|
Solid Based | Powder Based | |||
---|---|---|---|---|
Technology | WAAM | SLM-DMLS | EBM | BJ |
Material extrusion + welding | Melting | Melting | Binding | |
Electric arc | Laser beam | Electron beam | Bonding agent | |
Metal wires | Metal powders | Metal powders | Metal powders | |
| Titanium Steel Nickel Aluminium (or any weldable metal) | Stainless steel Aluminium alloys Titanium alloys Nickel alloys | Stainless steel Titanium alloys Nickel alloys Cobalt chrome | Stainless steel Bronze |
No | Yes | Yes | No | |
Unlimited build volume | From 100 × 100 × 100 mm (small sizes) to 800 × 500 × 400 mm (large sizes) | 350 × 350 × 450 mm | Up to 800 × 500 × 400 mm | |
1 mm | 0.1 mm | 0.1 mm | 0.2 mm | |
50–250 µm | 10–50 µm | 15–75 µm | variable | |
min 1–2 mm | 30–50 µm | 30–50 µm | 100 µm | |
Aerospace, energy sector, research and development, cladding and repair components | Medical and dental industry, aerospace and automotive sectors | Realistic models, coloured components, casting models with complex shapes |
The statements, opinions and data contained in all publications are solely those of the individual author(s) and contributor(s) and not of MDPI and/or the editor(s). MDPI and/or the editor(s) disclaim responsibility for any injury to people or property resulting from any ideas, methods, instructions or products referred to in the content. |
Share and Cite
Capasso, I.; Andreacola, F.R.; Brando, G. Additive Manufacturing of Metal Materials for Construction Engineering: An Overview on Technologies and Applications. Metals 2024 , 14 , 1033. https://doi.org/10.3390/met14091033
Capasso I, Andreacola FR, Brando G. Additive Manufacturing of Metal Materials for Construction Engineering: An Overview on Technologies and Applications. Metals . 2024; 14(9):1033. https://doi.org/10.3390/met14091033
Capasso, Ilaria, Francesca Romana Andreacola, and Giuseppe Brando. 2024. "Additive Manufacturing of Metal Materials for Construction Engineering: An Overview on Technologies and Applications" Metals 14, no. 9: 1033. https://doi.org/10.3390/met14091033
Article Metrics
Article access statistics, further information, mdpi initiatives, follow mdpi.
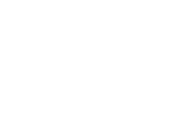
Subscribe to receive issue release notifications and newsletters from MDPI journals
- Biomedical Engineering
- Chemical & Biomolecular Engineering
- Civil and Environmental Engineering
- Computer & Data Sciences
- Electrical, Computer and Systems Engineering
- Macromolecular Science & Engineering
- Materials Science & Engineering
- Mechanical & Aerospace Engineering
- Intranet Login
Search form
Case school of engineering.
- Global Opportunities
- Outside The Classroom
Advanced Materials
- Big Data and Digital Solutions
- Energy and Sustainability
- High-Performance Manufacturing
- Human Health
- Micro-/Nano-electronics and Sensors
- Robotics and Motion Control
- Smart Systems and Cities
- Take Your Idea to Market
- Faculty Achievements
- Peer-Reviewed Publications
- Research News
Recent Grants
$155k | dod office of naval research | | "", $110k | american chemical society | mark de guire | "chemical expansivity in ceramic oxygen transport materials", $100k | nsf | gary wnek | "planning grant: engineering research center for advanced films & packaging", recent publications, through-thickness inhomogeneity of sensitization on environmentally assisted cracking in aa5083-h128 alloy, 3d interpenetrated graphene foam/58s bioactive glass scaffolds for electrical-stimulation-assisted differentiation of rabbit mesenchymal stem cells to enhance bone regeneration, facile synthesis of nanostructural high-performance cu-pb electrocatalysts for co2 reduction, gecko-inspired adhesive.
Gecko-inspired adhesive holds firm in extreme temperatures
Knotty polymers
Unraveling the secrets of knotty polymers
Materials Branch
Explore the materials branch
The range of elements available for device production has exploded in the past 20 years, enabling entirely new classes of electronic and mechanical products, biomedical implants, tissues, coatings and more.
We’re leaders in the world of advanced materials, with three of our school’s seven departments moving this field forward: Materials, Macromolecular Science and Engineering and Chemical and Biomolecular Engineering. And with our home in Northeast Ohio—a region brimming with material and chemical industry leaders—we collaborate on moving the latest materials technologies quickly from research to use.
Centers and institutes that conduct research in Advanced Materials
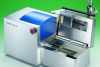
Center for Layered Polymeric Systems (CLiPS)
An NSF Science and Technology Center advancing the nation's science and technology agenda through the development of new materials and material systems
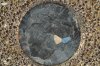
Center for the Evaluation of Implant Performance
Advances the science of joint replacement durability and performance through improvements in implant materials and design.
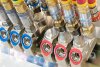
Advanced Manufacturing and Mechanical Reliability Center
Develops and provides mechanical testing for materials using an array of equipment.
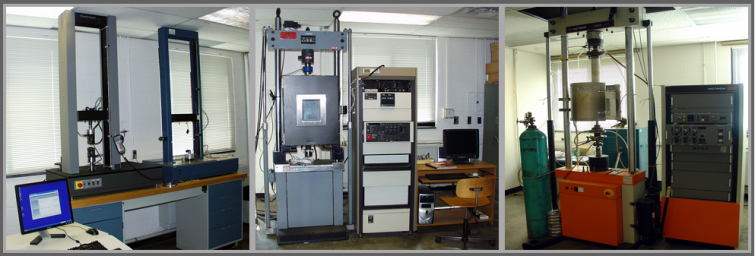
Swagelok Center for Surface Analysis of Materials
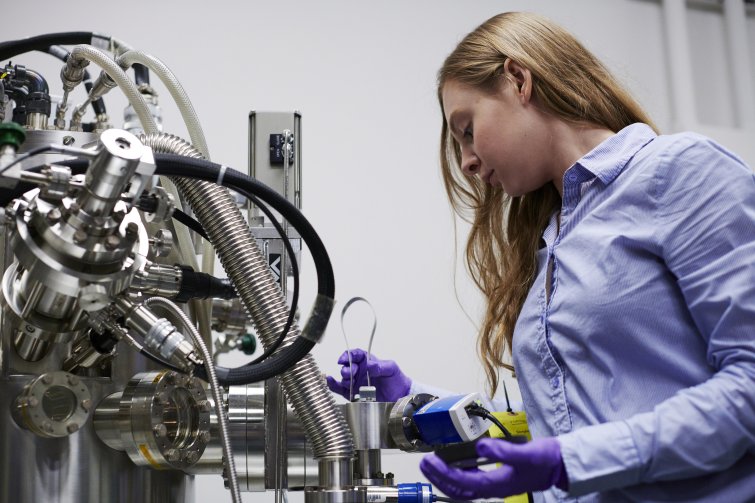
Provides expert staff and specialized analytical equipment to solve materials characterization challenges encountered in education, research and industry.
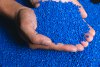
Center for Advanced Polymer Processing
Develops new advanced and functional multiphase complex materials and optimizes the performance of existing ones by integrating the most advanced experimental and computational capabilities.
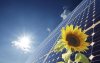
SDLE Research Center
Studies solar photovoltaic and other outdoor exposed technologies using degradation science, data science and analytics.
Faculty who conduct research in Advanced Materials
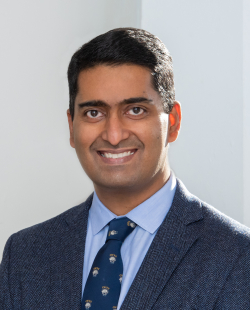
Rohan Akolkar
Develops new electrochemical processes for applications including nano-material fabrication, energy storage, electrometallurgy and sensors
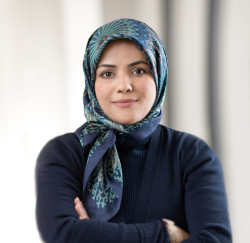
Hoda Amani Hamedani
Develops multifunctional materials and flexible nanostructured platforms for electrochemical and biomedical devices, localized drug delivery, neural interfacing, and electrochemical sensing; studies nanomaterials evolution/interactions in controlled (liquid) environments using in-situ characterization techniques
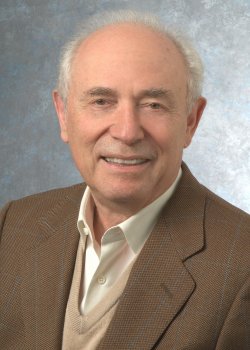
Develops processing-structure-property relationships in polymeric systems; creates micro- and nano-layered films; and produces biomimetic hierarchical structures of soft materials
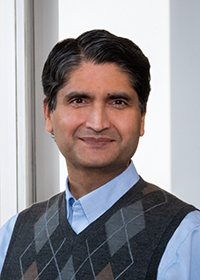
Harihara Baskaran
Understands and solves problems in biology and medicine using transport principles
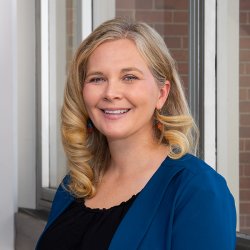
Laura Bruckman
Develops predictive lifetime models for materials degradation related to stress conditions and induced degradation mechanisms evaluated by quantitative spectroscopic characterization of materials
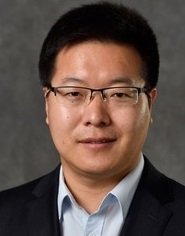
Changyong (Chase) Cao
Study the mechanics, designs, and manufacturing of smart multifunctional materials, soft robotics, soft electronics, and self-powered sensing systems.
Laboratory for Soft Machines & Electronics (SME Lab) www.CaoGroup.org
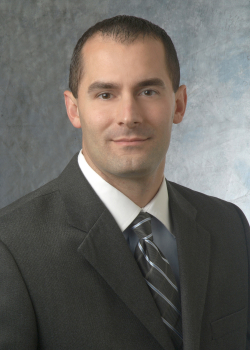
Jeff Capadona
Engineers biomaterials-based strategies to improve the performance and longevity of central nervous system implanted devices
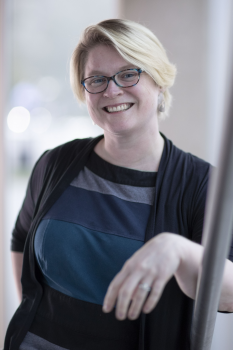
Jennifer Carter
deformation mechanisms of metals and metal-matrix composites; fatigue, fracture, and creep; failure analysis; electron microscopy; 3D microscopy; novel methodologies for multi-scale material characterization; data science and analytics; open science
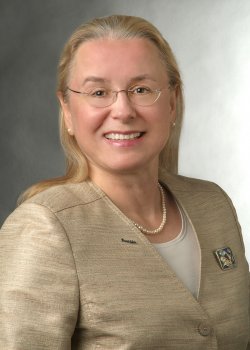
Sunniva Collins
Engineers metallic surfaces for improved performance; selects materials and designs manufacturing processes for innovative outcomes
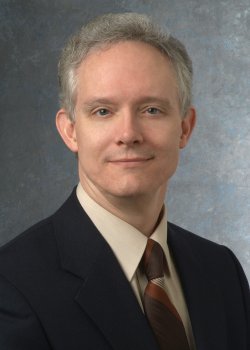
Mark De Guire
Analyzes performance of ceramics in energy applications, including fuel cells and oxygen transport membranes
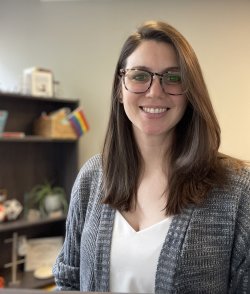
Christine Duval
Develops separation materials and processes to benefit nuclear medicine, environmental protection, and nuclear waste recycling and remediation.
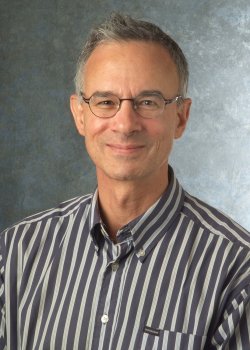
Steven Eppell
Designs, synthesizes and tests orthopedic biomaterials using biomimetic strategies emphasizing nanoscale structures and self-assembly
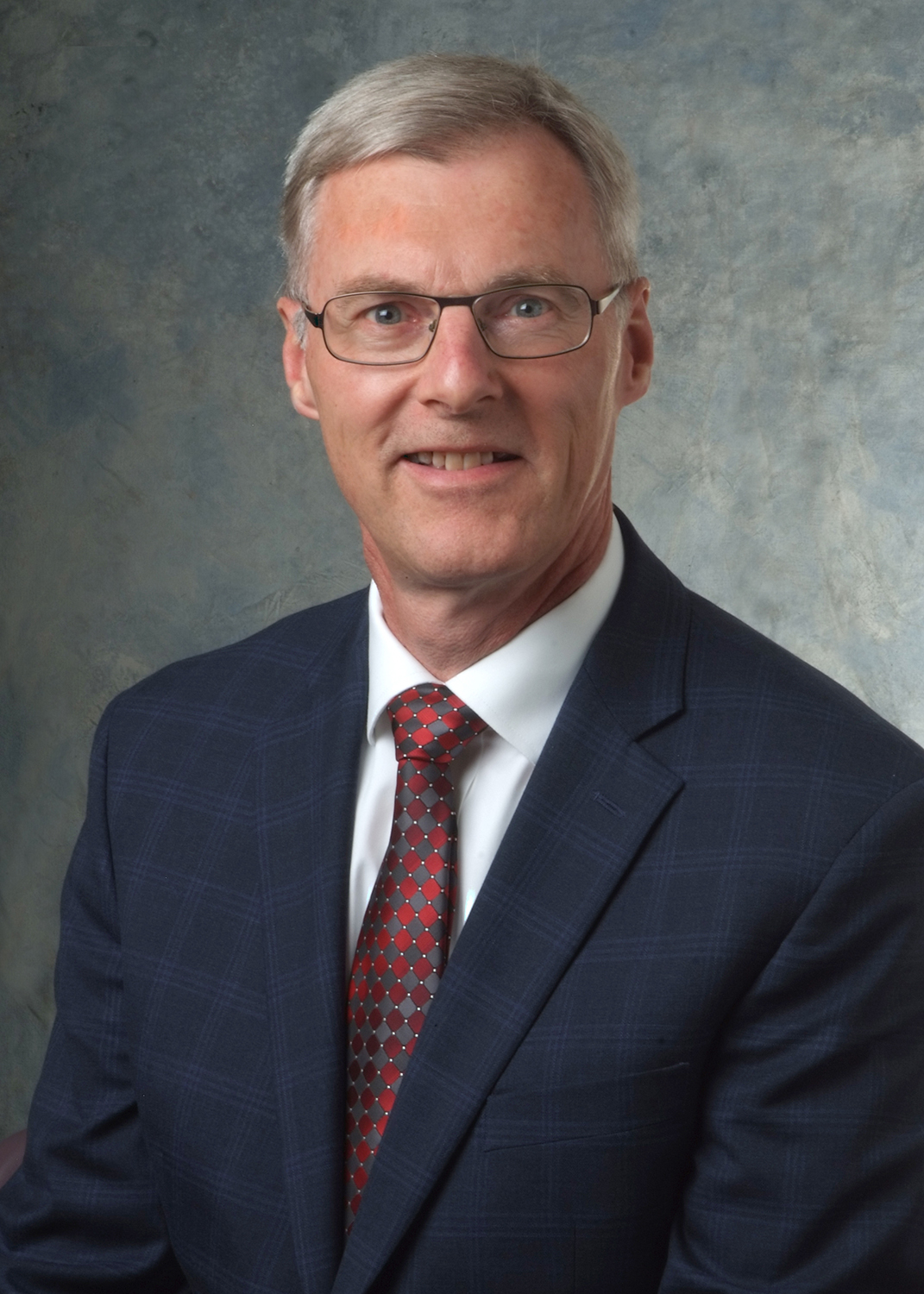
Frank Ernst
Studies and engineers microstructures, interfaces and surfaces of metallic materials by novel methods of processing and microcharacterization
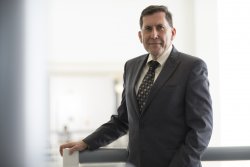
Donald Feke
Develops novel polymeric materials and ultrasonic-based separation processes for nano- and microscale multi-phase systems
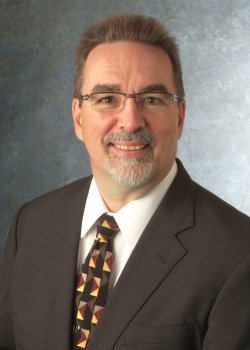

Roger French
Applies data science and analytics to energy and materials science research problems
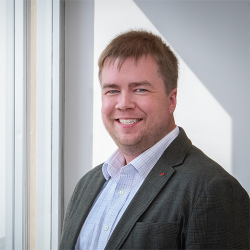
Michael Hore
Combines experiments, theory and simulation to study fundamental problems in the polymer physics of grafted and non-linear polymers
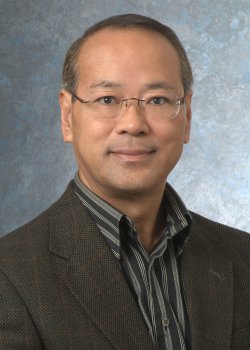
Hatsuo Ishida
Develops advanced benzoxazine resins, thermosetting resins based on natural renewable materials and green flame retarding systems
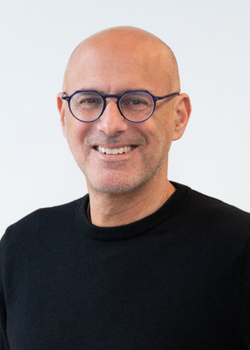
Daniel Lacks
Develops first-principles molecular-scale theories of chemical processes and materials properties
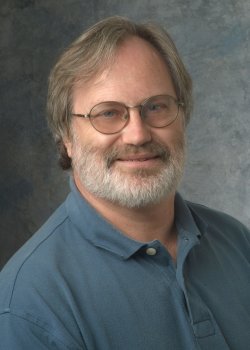
Peter Lagerlof
Develops a unified theory for plastic deformation via slip and deformation twinning
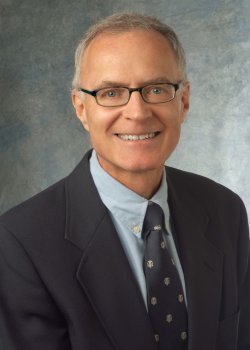
John Lewandowski
Researches material reliability for biomedical and structural applications, advanced materials manufacturing and processing/microstructure/property relationships. Hybrid Autonomous Manufacturing.
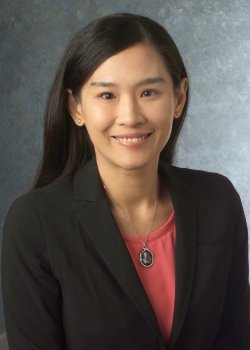
Ya-Ting Liao
Creates computational models of combustion, fire and fire behavior and develops fire-resistant structures
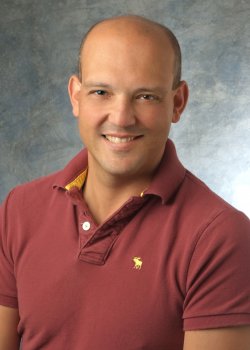
Develops multi-scale, complex polymer-based materials, using both experimental and computational-based tools
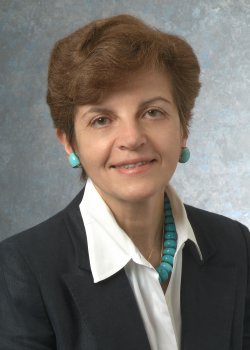
Ica Manas-Zloczower
Engineering new materials and technologies for industrial applications
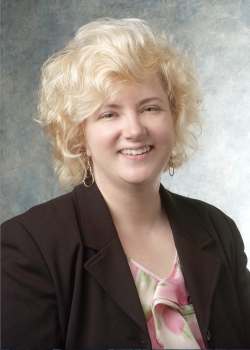
Heidi Martin
Develops diamond electrodes for electrochemical and neural device applications
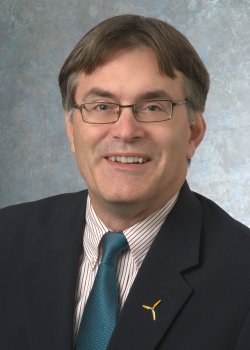
David Matthiesen
Develops process engineering solutions for the manufacturing of new magnetic materials
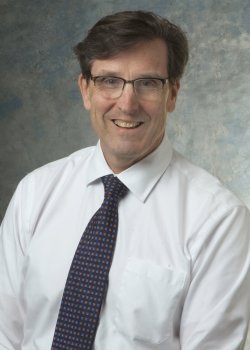
Jim McGuffin-Cawley
Develops new methodologies for material processing, characterizes processing-property relationships, studies high-temperature diffusion and solid-state reactions, fosters industry-university relationships
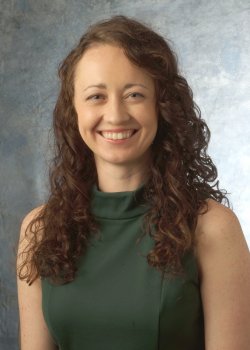
Julie Renner
Develops biomolecular platforms to control solid-liquid interfaces and enable a new generation of advanced technologies
Publications
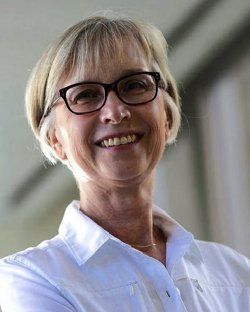
Clare Rimnac
Advances the long-term performance of implants and structural bone allografts through material analysis and characterization
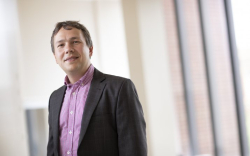
Valentin Rodionov
Investigates catalysis with soft materials: catalytic surfactants and polymers; complex macromolecular architectures for bio-inspired catalysis; and ligand-mediated nanocatalysis
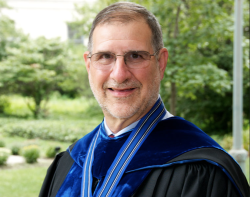
Robert Savinell
Develops high-performance electrochemical energy conversion and storage technologies through fundamental and applied studies of interfacial and transport processes
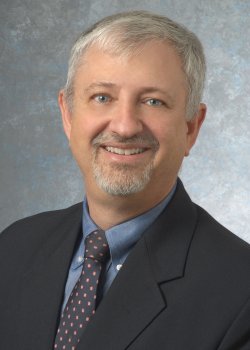
David Schiraldi
Develops bio-based, flame-retarded plastics, polymer aerogels and packaging materials; and studies properties of polyesters
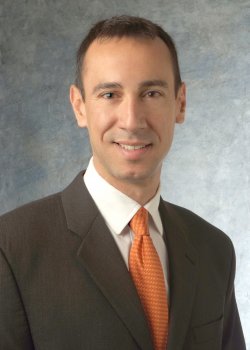
Alp Sehirlioglu
Develops new materials through exploitation of interfaces to control functionality and exploration of multi-functionality for energy-related applications
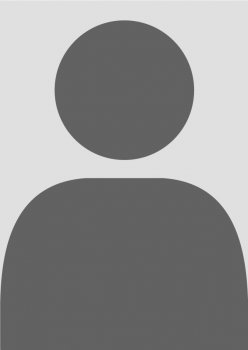
Tina Vrabec
Develops novel waveforms and electrodes for downregulation of the nervous system for clinical applications including peripheral, autonomic and pain
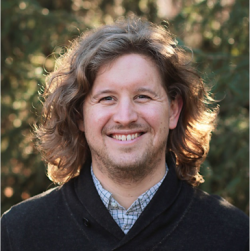
Robert Warburton
Develops computational models of interfacial chemical reactions relevant to applications in catalysis and energy storage
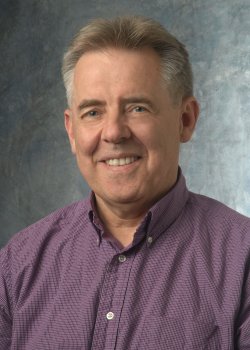
Gerhard Welsch
Develops new processing methods and designs for energy storage and optimized materials
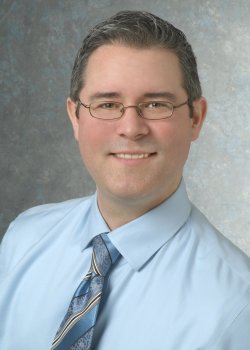
Matthew Willard
Investigates phase transformations and materials processing, especially their impact on structure and properties of materials
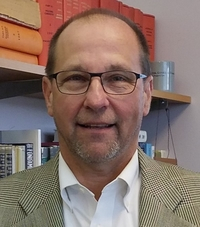
Develops advanced polymers for packaging, biomaterial applications and more.
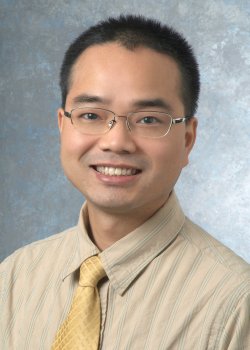
Multiscale multiphysics processes in geosystems and infrastructure, interdisciplinary innovations in intelligent infrastructure technologies towards resilience and sustainability.
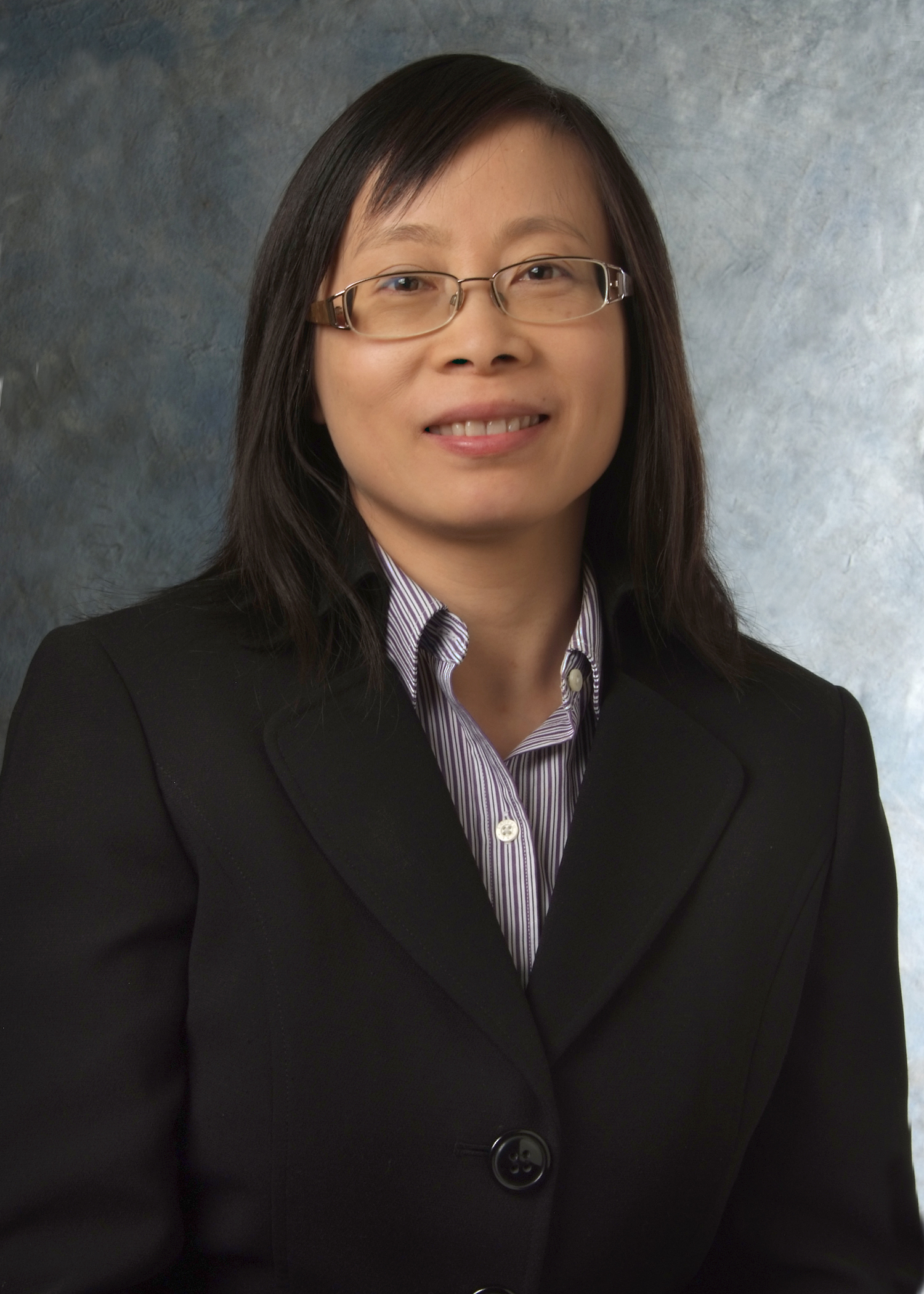
Huichun (Judy) Zhang
Examines fate and transformation of environmental contaminants in natural and engineered environments and develops advanced water/wastewater treatment technologies
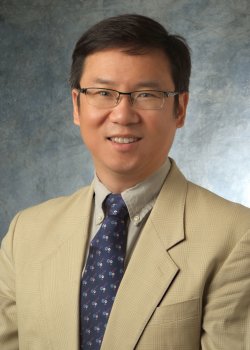
Develops high-energy and high-performance polymers based on close relationships among structure, property and processing
- Skip to Content
- Bulletin Home
2024-25 General Bulletin

Department of Materials Science and Engineering
White Building (7204) Phone: 216.368.4230 Department Chair: Frank Ernst [email protected]
Materials science and engineering is a discipline that extends from understanding the microscopic structure and properties of materials to designing materials in engineering systems and evaluating their performance. Achievements in materials engineering underpin the revolutionary advances in technology that define the modern standard of living. Materials scientists and engineers understand how the properties of materials relate to their microscopic structure and composition and engineer the synthesis and microstructure of materials to advance their performance in conventional and innovative technical applications.
The Department of Materials Science and Engineering of the Case School of Engineering offers programs leading to the degrees of Bachelor of Science in Engineering, Master of Science, and Doctor of Philosophy. The technological challenges that materials engineers face demand knowledge across a broad spectrum of materials. The Department conducts academic and research activities with metals, ceramics, semiconductors, polymers, and composites. Timely research and education respond to the demands for new materials and improved materials performance in existing applications, often transcending the traditional materials categories.
While a discipline of engineering, the field brings basic science to bear on the technological challenges related to the performance of industrial products and their manufacture. Materials science draws on chemistry in its concern for bonding, synthesis, and composition of engineering materials and their chemical interactions with the environment. Physics provides a basis for understanding the atomistic and electronic structure of materials and how they determine mechanical, thermal, optical, magnetic, and electrical properties. Mathematics, computation, and data science provide quantitative physical theories and modeling of the atomistic and electronic structure and provide advances in methods for microstructural analysis, materials design, and manufacturing processes.
The Department of Materials Science and Engineering engages faculty, students, postdoctoral researchers, engineers, and staff in developing and understanding relationships between processing, structure, properties, and the performance of materials in engineering applications. The Department provides a research-intensive environment that encourages collaboration and underpins modern education of undergraduate and graduate students as well as professionals in the field. This environment provides a strong foundation for advancing the frontiers of materials research, developing important technical innovations, and preparing engineers and scientists for challenging leadership careers.
Research Areas
Deformation and fracture.
Stress–strain relations during elastic and anelastic deformation. Plastic deformation mechanisms controlled by dislocation activity, twinning, or transformation-induced shear mechanisms, as well as by creep and viscous flow mechanisms under uniaxial, biaxial, and triaxial stress states, in particular in plane-strain and/or plane-stress conditions. Relationships between structure (atomistic structure and microstructure) and mechanical behavior of crystalline and glassy materials, including metals, intermetallics, semiconductors, ceramics, and composites. State-of-the-art facilities are available for testing mechanical properties over a range of strain rates, test temperatures, stress states, and size scales under monotonic and cyclic loading and under stress–corrosion conditions.
Materials Processing
Phase-transformation- and thermo-mechanical processing of alloys, including solution-, precipitation-, recovery-and-recrystallization- and stress-relief heat-treatments, also for intentional generation of residual-stresses. Deformation processing of materials. Surface engineering, crystal growth, sputter-, vapor- and laser-ablation synthesis of films. Melting and casting of metal alloys into sand/ceramic molds, injection into metallic molds, and by rapid solidification to form crystalline or (metallic-) glass ribbons. Ceramic- and metal powder synthesis. Consolidation processing by cold-pressing and sintering, electric-field-assisted compaction, or hot-pressing. Composite materials by forming of layered materials, electroplated metals, diffusion-bonding, brazing, and welding. Electrochemical- and thermo-chemical conversion processing, e.g. oxide-film growth by anodizing or thermochemical conversion. Synthesis of micro-to-nano-porous metal/oxide structures, e.g. for battery and capacitor electrodes or for catalyst support.
Environmental Effects
Durability and lifetime extension of structural, energy-conversion-, and energy-storage materials, including materials for solar energy conversion. Corrosion, oxidation, stress-corrosion, low- and high-cycle fatigue, adhesion, decohesion, friction, and wear. Surface modification and coatings, adhesion, bonding, and dis-bonding of dissimilar materials, reliability of electronics, photonics, and sensors.
Surfaces and Interfaces
Material surfaces in vacuum, ambient-, and chemical environments, grain- and phase boundaries, hetero-interfaces (interfaces between different metals, ceramics, carbon/graphite, polymers, and combinations thereof).
Electronic, Magnetic, and Optical Materials
Materials for energy conversion technologies, such as photovoltaics, organic and inorganic light-emitting diodes and displays, fuel cells, electrolytic capacitors, solid-state Li-ion batteries, and building-envelope materials. Processing, properties, and characterization of magnetic, ferroelectric, and piezoelectric materials.
Microcharacterization of Materials
Facilities for high-resolution imaging, spatially resolved chemical analysis and spectrometry, and diffractometry. Conventional, analytical, and high-resolution transmission electron microscopy, scanning electron microscopy, focused ion beam techniques, scanning probe microscopy, light-optical microscopy, optical and electron spectroscopies, surface analysis, and X-ray diffractometry.
Materials Data Science
Rapid qualification of alloys, data science applications in polymers and coatings. Distributed computing, informatics, statistical analytics, exploratory data analysis, statistical modeling, and prediction. Hadoop, cloud computing, and computationally intensive research are supported through the operation of a scalable high-performance computing (HPC) system.
Frank Ernst, Dr. rer. nat. habil. (University of Göttingen) Leonard Case Jr. Professor of Engineering https://goo.gl/OWsF9K Microstructure and microcharacterization, alloy surface engineering, defects in crystalline materials, interface- and stress-related phenomena.
Laura S. Bruckman, PhD (University of South Carolina) Associate Professor Materials data science, lifetime and degradation science, study protocol development, spatiotemporal data integration
Jennifer W. Carter, PhD (The Ohio State University) Associate Professor Processing–structure–property relationships of crystalline and amorphous materials. Multi-scale material characterization methods for correlating local microstructural features with mechanical and environmental responses.
Roger H. French, PhD (Massachusetts Institute of Technology) Kyocera Professor Optical properties and electronic structure of polymers, ceramics, optical and biomolecular materials. These determine the vdW interactions which drive wetting of interfaces and mesoscale assembly biomolecular and inorganic systems including CNTs, proteins and DNA. Energy research focused on lifetime and degradation science. Including developing CRADLE, a Hadoop/Hbase/Spark-based distributed computing environment, for data science and analytics of complex systems such as photovoltaics and outdoor exposed materials. This allows multi-factor real-world performance to be integrated with lab-based datasets to identify mechanisms and pathways activated over lifetime using statistical and machine learning.
Hyeji Im, PhD (Korea Advanced Institute of Science and Technology) Assistant Professor Development and manufacturing of structural materials, extreme environment materials, metal additive manufacturing, sustainable alloy design and processing, materials characterization and metallurgical phenomena, and nano-microstructure control.
Neamul Khansur (UNSW Sydney, Australia) Assistant Professor Processing-structure-properties relationships in multi-functional materials. Powder aerosol deposition for fabricating protective and functional ceramic coatings. In situ X-ray and neutron diffraction characterization methods for non-linear dielectric ceramics
John J. Lewandowski, PhD (Carnegie Mellon University) Arthur P. Armington Professor of Engineering Mechanical behavior of materials. Fracture and fatigue. Micromechanisms of deformation and fracture. Composite materials. Bulk metallic glasses and composites. Refractory metals. Toughening of brittle materials. High-pressure deformation and fracture studies. Hydrostatic extrusion.
James D. McGuffin-Cawley, PhD (Case Western Reserve University) Arthur S. Holden Professor of Engineering Powder processing of ceramics. Manufacturing and materials. Additive manufacturing and rapid prototyping. Aggregation phenomena. Defects, diffusion, and solid state reactions. Materials for optical devices.
Alp Sehirlioglu, PhD (University of Illinois at Urbana Champaign) Associate Professor Energy conversion materials, including piezoelectrics and thermoelectrics. Bulk and film electro-ceramics. Epitaxial oxide films.
Gerhard E. Welsch, PhD (Case Western Reserve University) Professor High-temperature materials. Materials for capacitive energy storage. Metals, metal sponges, oxides. Mechanical and electrical properties. Synthesis.
Matthew A. Willard, PhD (Carnegie Mellon University) Associate Professor Magnetic materials: properties, microstructure evolution, phase formation, and processing conditions. Rapid solidification processing. Soft magnetic materials. Permanent magnet materials. Magnetic shape memory alloys, magnetocaloric effects, magnetic nanoparticles, and multiferroics.
Research Faculty
Erika Barcelos, PhD (Case Western Reserve University) Research Assistant Professor
Janet L. Gbur, PhD (Case Western Reserve University) Research Assistant Professor Fatigue and fracture of medical materials, mechanical behavior of superelastic Nitinol; development of microscale medical devices for rehabilitation; and flexible circuit fabrication using aerosol jet printing.
Hoda Amani Hamedani, PhD (Georgia Institute of Technology) Research Assistant Professor Nanomaterials synthesis and characterization for electrochemical energy harvesting, conversion (solar cells, fuel cells). Nanostructured platforms for biomedical applications including flexible bioelectronics and implantable microdevices, localized drug delivery, neural interfacing, sensing and in vivo power generation.
Pawan Tripathi, PhD (Indian Institute of Technology) Research Assistant Professor Materials Data Science, Advanced Manufacturing, Synchrotron Data Analysis, Image Processing, Deep Learning, Artificial Intelligence, Uncertainty Quantification, Atomistic Simulation
Jeffrey Yarus, PhD (University of South Carolina) Research Professor Applications of data science and statistics in materials science, materials engineering, and geology.
Secondary Faculty
Clemens Burda, PhD Professor Chemistry
Sunniva Collins, PhD Associate Professor Mechanical Engineering
Liming Dai, PhD Kent Hale Smith Professor Macromolecular Science and Engineering
Walter Lambrecht, PhD Professor Physics
Clare Rimnac, PhD Professor Mechanical Engineering
Mohan Sankaran, PhD Goodrich Professor of Engineering Innovation Chemical Engineering
Russell Wang, DDS Associate Professor Dentistry
Xiong (Bill) Yu, PhD, PE Professor Civil Engineering
Adjunct Faculty
Jennifer Braid, PhD (Colorado School of Mines) Adjunct Professor Developing data science and computer vision techniques for PV module and system research
Arnon Chait, PhD (The Ohio State University) Adjunct Professor NASA Lewis Research Center
Mark DeGuire, PhD (Massachusetts Institute of Technology) Adjunct Associate Professor
George Fisher, PhD Adjunct Professor Ion Vacuum Technologies Corporation
N.J. Henry Holroyd, PhD (Newcastle University) Adjunct Professor Luxfer Gas Cylinders
Jeffrey J. Hoyt, PhD (University of California, Berkeley) Adjunct Professor McMaster University
Jennie S. Hwang, PhD (Case Western Reserve University) Adjunct Professor H-Technologies Group
Peter Lagerlof, PhD (Case Western Reserve University) Adjunct Associate Professor
Ina Martin, PhD (Colorado State University) Adjunct Assistant Professor Case Western Reserve University
Farrel Martin, PhD Adjunct Professor United States Naval Research Laboratory
David Matthiesen, PhD (Massachusetts Institute of Technology) Adjunct Associate Professor
Terence Mitchell, PhD (University of Cambridge) Adjunct Professor Los Alamos National Laboratory
Erik Mueller, PhD (University of Florida) Adjunct Assistant Professor
Badri Narayanan, PhD (The Ohio State University) Adjunct Assistant Professor Lincoln Electric
Joe H. Payer, PhD Adjunct Professor University of Akron
Timothy Peshek, PhD (Case Western Reserve University) Adjunct Assistant Professor NASA Glenn Research Center
Rudolph Podgornik, PhD (University of Ljubljana) Adjunct Professor University of Ljubljana
Gary Ruff, PhD (Case Western Reserve University) Adjunct Professor Ruff Associates
Ali Sayir, PhD (Case Western Reserve University) Adjunct Professor Air Force Office of Scientific Research
Mohsen Seifi, PhD (Case Western Reserve University) Adjunct Assistant Professor ASTM International
Emeritus Faculty
William A. "Bud" Baeslack III, PhD (Rensselaer Polytechnic Institute) Professor Emeritus Welding, joining of materials, and titanium and aluminum metallurgy
Mark De Guire, PhD (Massachusetts Institute of Technology) Associate Professor Emeritus Synthesis and properties of ceramics in bulk and thin-film form, including fuel cell materials, gas sensors, coatings for biomedical applications, photovoltaics, and ferrites. Testing and microstructural characterization of materials for alternative energy applications. High-temperature phase equilibria. Defect chemistry.
Arthur H. Heuer Professor Emeritus
Peter Lagerlof, PhD (Case Western Reserve University) Associate Professor Emeritus Mechanical properties of ceramics and metals. Low-temperature deformation twinning. Light-induced plasticity of semiconductors. Methodology of transmission electron microscopy and diffractometry.
David Matthiesen, PhD (Massachusetts Institute of Technology) Associate Professor Emeritus Nitride-based ferromagnetic materials. Applied atomistic simulation of materials. Materials for use in wind turbines. Wind resource measurements onshore and offshore. Materials interactions with ice. Bulk crystal growth processing. Process engineering in manufacturing. Heat, mass, and momentum transport.
Pirouz Pirouz Professor Emeritus
- Applied Data Science, Graduate Certificate
- Applied Data Science, Minor
- Materials Science and Engineering, BSE
- Materials Science and Engineering, Minor
- Materials Science and Engineering, MS
- Materials Science and Engineering, PhD
Dual Degrees
- Programs Toward Graduate or Professional Degrees
Related Majors in Other Departments
- Data Science and Analytics, BS (administered by the Department of Computer and Data Sciences )
Advanced Manufacturing and Mechanical Reliability Center (AMMRC)
White Building 115, 211, 216, 222, 300, 338
Deformation Processing Laboratory: White Building 115 Nitonol Commercialization Accelerator: White Building 300, 338 Mechanical Testing Laboratories: White Building 211, 216, 222
Contact: John Lewandowski 216-368-4234 [email protected]
The AMMRC (Advanced Manufacturing and Mechanical Reliability Center) permits the determination of mechanical behavior of materials over loading rates ranging from static to impact, with the capability of testing under a variety of stress states under either monotonic or cyclic conditions. A variety of furnaces and environmental chambers are available to enable testing at temperatures ranging from -196 °C to 1800 °C. The facility is operated under the direction of a faculty member and under the guidance of a full-time engineer. The facility contains one of the few laboratories in the world for high-pressure deformation and processing, enabling experimentation under a variety of stress states and temperatures. This state-of-the-art facility includes the following equipment:
- High-Pressure Deformation Apparatus: Th is unit enables tension or compression testing to be conducted under conditions of high hydrostatic pressure and consists of a pressure vessel and diagnostics for measurement of load and displacement on deforming specimens, as well as instantaneous pressure in the vessel. Pressures up to 1.0 GPa loads up to 10 kN, and displacements of up to 25 mm are possible. This oil-based apparatus can be operated at temperatures up to 300 °C.
- Hydrostatic-Extrusion Apparatus: Hydrostatic extrusion (e.g. pressure-to-air, pressure-to-pressure) can be conducted at temperatures up to 300 °C on manually operated equipment interfaced with a computer data acquisition package. Pressures up to 2.0 GPa are possible, with reduction ratios up to 6 to 1, while various diagnostics provide real time monitoring of extrusion pressure and ram displacement.
- Advanced Forging-Simulation Rig: A multi-actuator MTS machine based on 1.5 MN, four post frame, enables sub-scale forging simulations over industrially relevant strain rates. A 490 kN forging actuator is powered by five nitrogen accumulators enabling loading rates up to 3.0 m/s on large specimens. A 980 kN indexing actuator provides precise deformation sequences for either single, or multiple, deformation sequences. Dat a acquisition at rates sufficient for analysis is available. Testing with heated dies is possible.
- Advanced Metal-Forming Rig: A four-post frame with separate control of punch actuator speed and blank hold down pressure enables determination of forming limit diagrams. Dynamic control of blank hold down pressure is possible, with maximum punch actuator speeds of 30.0 cm/s. A variety of die sets are available.
- Servo-hydraulic Machines: Four MTS Model 810 computer-controlled machines with load capacities of 13 kN, 90 kN, 220 kN, and 220 kN, permit tension, compression, and fatigue studies to be conducted under load-, strain-, or stroke control. Fatigue crack growth may be monitored via a DC potential drop technique as well as via KRAK gauges applied to the specimen surfaces. Fatigue studies may be conducted at frequencies up to 30 Hz. In addition, an Instron Model 1331 90 kN Servo-hydraulic machine is available for both quasi-state and cyclic testing.
- Universal Testing Machines: Three INSTRON screw-driven machines, including two INSTRON Model 1125 units permit tension, compression, and torsion testing.
- Electromechanical Testing Machine: A computer-controlled INSTRON Model 1361 can be operated under load-, strain-, or stroke control. Stroke rates as slow as 0.3 nm/s are possible.
- Fatigue Testing Machines: Three Sonntag fatigue machines and two R. R. Moore rotating-bending fatigue machines are available for producing fatigue-life (S–N) data. The Sonntag machines may be operated at frequencies up to 60 Hz.
- Creep Testing Machines: Three constant load frames with temperature capabilities up to 800 °C permit creep testing, while recently modified creep frames permit thermal cycling experiments as well as slow cyclic creep experiments.
- Impact Testing Machines: Two Charpy impact machines with capacities ranging from 20 ft-lbs to 240 ft-lbs are available. Accessories include a Dynatup instrumentation package interfaced with an IBM PC, which enables recording of load vs. time traces on bend specimens as well as on tension specimens tested under impact conditions.
- Instrumented Microhardness Tester: A Nikon Model QM High-Temperature Microhardness Tester permits indentation studies on specimens tested at temperatures ranging from -196 °C to 1 200 °C under vacuum and inert gas atmospheres. This unit is complemented by a Zwick Model 3212 Microhardness Tester as well as a variety of Rockwell Hardness and Brinell Hardness Testing Machines.
Swagelok Center for Surface Analysis of Materials
Glennan Building 101
Contact: Jennifer Carter, 216-368-4214, [email protected] Jeffrey Pigott, 216-368-6012, [email protected] Website: https://engineering.case.edu/centers/scsam/
SCSAM, the Swagelok Center for Surface Analysis of Materials, is a multi-user facility providing cutting-edge major instrumentation for microcharacterization of materials. SCSAM is administered by the CSE (Case School of Engineering) and is central to much of the research carried out by CSE's seven departments. The facility is also extensively used by the CAS (College of Arts and Sciences) Departments of Physics, Chemistry, Biology, and Earth, Environmental, and Planetary Sciences, as well as many departments within the School of Medicine and the School of Dental Medicine. Typically, more than 200 users, mostly academic, utilize the facility per year.
SCSAM's instruments encompass a wide and complementary range of characterization techniques, which provide a comprehensive resource for high-resolution imaging, diffractometry, and spatially-resolved compositional analysis.
Current capabilities for high-resolution imaging include: an AFM (atomic force microscope) which can optionally be operated with an imaging nanoindenter scan head or a stand-alone automated nanoindenter; a Keyence optical microscope providing the next-generation of optical microscopy with a large depth-of-field and advanced measurement capabilities for inspection and failure analysis.; two scanning electron microscopes, one equipped for FIB (focused ion beam) micromachining, and both equipped with XEDS (X-ray energy-dispersive spectrometry), TSEM (transmission scanning electron microscopy), and EBSD (electron backscatter diffraction) detectors.
For XRD (X-ray diffractometry), SCSAM provides two diffractometers with 1D and 2D detectors to allow for phase identification, phase fraction determination, crystal structure refinements, as well as stress and strain measurements of crystalline solids.
SCSAM's surface analysis suite of instruments includes an instrument for ToF-SIMS (time-of-flight secondary-ion mass spectrometry), a SAM (scanning Auger microprobe) for spatially resolved AES (Auger electron spectroscopy), and an instrument for XPS (X-ray photoelectron spectroscopy, also known as ESCA, electron spectrometry for chemical analysis), that accomplishes high spatial resolution by operating with a focused X-ray beam.
SCSAM’s instruments are housed in a centralized area allowing users convenient access to state-of-the-art tools for their research.
Magnetometry Laboratory
Contact: Matthew Willard 216-368-5070
The Magnetometry Laboratory has facilities used to investigate the magnetic properties of materials. This laboratory has the following instruments:
Lake Shore Cryotronics Model 7410 Vibrating Sample Magnetometer This instrument serves for measurement of hysteresis loops (at constant temperature) and thermomagnetic measurements (at constant magnetic field). The maximum applied field at room temperature (without furnace in place) is 3.1 T. For high temperature measurements, the maximum applied field is 2.5 T over the temperature range from room temperature to 1000 °C.
Home-Built Magnetostriction Measurement System This system has been designed and built to measure the shape change of magnetic materials under applied magnetic fields. Better than 1 ppm sensitivity is possible by this strain gauge technique. An applied field of ≈0.2 T is used to saturate samples.
SDLE Research Center
White Building 538
Contact: Roger French
216-368-3655
The SDLE Research Center was established in 2011 as a Wright Project Center with funding from Ohio Third Frontier. Initially it was dedicated to advancing the fields of lifetime and degradation science using data science. The research center activities have expanded to include research focused on the durability and degradation of environmentally exposed, long-lived materials and technologies such as photovoltaics (PV), coatings, energy efficient lighting, and building envelope applications, as well as broad-based collaborations in materials data science in reliability and degradation, carbon capture and storage, geothermal energy applications.
Today the SDLE Research Center is advancing the development of Materials Data Science for use in Materials Reliability, Materials Science and broader application areas.
The SDLE Research Center, also includes the SDLE Core Facility, which is a CWRU User Facility, which provides both reliability and Materials Data Science tools and capabilities available to the CWRU community, other academic researchers and industrial and national laboratory researchers.
A data science approach is needed to handle large-scale data on materials, components, systems, modules, commercial power plants, and the grid. These approaches involve data ingestion into nonrelational data warehouses and data-driven modeling with a foundation in the underlying physics and chemistry of degradation and lifetime performance. Assembling FAIR (Findable, Accessible, Interoperable, Reusable) data and other data, developing and sharing codes and tools, and reporting research results along a materials value chain is a key component of the Center. The SDLE Research Center facilitates complex data-driven modeling, including geostatistical, geospatiotemporal modeling, graph network modeling, and degradation network models. The data analytics platform (CRADLE), an integrated distributed and high performance computing cluster, was developed in the center to facilitate large data storage and analysis with ease of access to team members enabling fleets of high performance computing jobs for improved data analytics.
The SDLE Center has developed a method to enable large-scale distributed analysis of commercial fleet scale photovoltaic (PV) power plants for both performance loss rate (PLR) determination and power forecasting. This study includes a set of timeseries datasets for 100,000 PV plant inverters and determines the data quality of these plants in relation to prediction of PLR. Additionally, a multi-year benchmarking and review of the impact of data quality and filtering, power prediction algorithms, and PLR determination methods has defined the challenges in PLR determination. The data quality, data gaps, and filtering of timeseries data of commercial fleets of PV plants restrict which algorithms analyses can use and can bias results and reduce their accuracy. Data quality and data gaps can be improved with spatio-temporal graph neural network (st-GNN) models of PV power plant data including satellite weather data and autoencoders for data imputation of missing data. FAIR data principles are used to make FAIR data and models in order to improve transferability of data and models.
The SDLE Center has a focus on materials data science in relation to long-lived materials. This work determines the degradation mechanisms in material systems, which can be mitigated to optimize lifetime performance of materials, components, and devices. Understanding these key degradation mechanisms in relation to the stress and stress level is fundamental to lifetime and degradation science (L&DS). By encompassing the knowledge from the experimental insights of the degradation of materials, the lifetime of materials can be predicted under multiple different stress conditions. Thus far traditional materials reliability has been flawed with costly failures in applications such as polyamide backsheet failure in photovoltaic (PV) modules. The Center has developed an epidemiological approach to understanding materials degradation which provides more scientific value by giving information on the standard deviation within a population. Additionally, by combining standard and modified accelerated exposures with real-world exposures, degradation can be more accurately predicted on a variety of different grades of materials or component structure. Then data-driven or network modeling provides insights into the impact of stress conditions on degradation and performance. Real-world degradation gives the information on the complex and synergistic nature of materials degradation compared to single or even combinational accelerated stressors. The unique environment that a material exists in the real world or in-use conditions is varied due to specific microstressors as well as the impact of climate change on climate zones.
Geostatistical geospatiotemporal modeling is an active area of research within SDLE which is a quantitative method for mapping phenomena that are inherently tied to geographic and/or temporal space. The method provides for estimating at unsampled locations and for simulating multiple equally probable realizations to assess the space of uncertainty in the subsurface, surface, or near surface environment. Applications include environmental, mineral resources, geothermal, hydrology, agriculture, climate, forestry, soil, air, and more.
The SDLE Research Center’s Core Facility has capabilities and equipment including:
Outdoor solar exposures: SunFarm with 14 dual-axis solar trackers with multi-sun concentrators, and power degradation monitoring
Solar simulators for 1-1000X solar exposures
Multi-factor environmental test chambers with temperature, humidity, freeze/thaw, and cycling
A full suite of optical, interfacial, thermo-mechanical, and electrical evaluation tools for materials, components, and systems
CRADLE: 800 Terabytes of nonrelational data warehouses based on Cloudera’s distribution of Apache’s Hadoop, Hbase, and Spark
High Performance Compute Cluster for data science and analytics
The Center for Materials Data Science for Reliability and Degradation (MDS-Rely)
The Center for Materials Data Science for Reliability and Degradation (MDS-Rely) is a National Science Foundation (NSF) Industry-University Cooperative Research Center (IUCRC) which CWRU leads in partnership with the University of Pittsburgh. Center Members from both industry and government join the Center as Members and directly fund center research. MDS-Rely seeks to apply data science-informed research to better understand the reliability and lifetime of essential materials, while creating code packages, models, and other materials-agnostic deliverables that are jointly owned by Member organizations .
Through a series of competencies, research thrusts, and materials value chains, MDS-Rely focuses on transferable research outputs while training a data-enabled workforce of students and graduates that enjoy strong, collaborative relationships with our Member organizations. Through a diverse research portfolio, MDS-Rely provides insight into the following areas:
1. Competencies: Standards & Reliability Protocols; Materials Data Science; Reliability, Performance, & Degradation Solutions 2. Research Thrusts: Weathering & Performance; Subtractive & Additive Manufacturing; Energy Technologies. 3. Materials Value Chains: Polymers, Elastomers, & Coatings; Metals & Alloys; Semiconductors & Optoelectronics; and an evolving focus on circular economy, sustainability, & climate.
MDS-Rely works in close partnership with the SDLE Research Center. Interested parties should reach out to Jonathan Steirer, Managing Director, at [email protected] or Roger French, Center Director, at [email protected]
Applied Data Science (DSCI)
Materials science and engineering (emse).
DSCI 330. Cognition and Computation. 3 Units.
An introduction to (1) theories of the relationship between cognition and computation; (2) computational models of human cognition (e.g. models of decision-making or concept creation); and (3) computational tools for the study of human cognition. All three dimensions involve data science: theories are tested against archives of brain imaging data; models are derived from and tested against datasets of e.g., financial decisions (markets), legal rulings and findings (juries, judges, courts), legislative actions, and healthcare decisions; computational tools aggregate data and operate upon it analytically, for search, recognition, tagging, machine learning, statistical description, and hypothesis testing. Offered as COGS 330 , COGS 430 , DSCI 330 and DSCI 430 .
DSCI 332. Spatial Statistics for Near Surface, Surface, and Subsurface Modeling. 3 Units.
This course is on spatial modeling of near surface, surface, and subsurface data, also known as geostatistical modeling. Spatial modeling has its origins in predictive modeling of minerals in subsurface formations, from which many examples are used in this class. Students will learn the basics of spatial models in order to understand how they are built from various data types and how their uncertainties are assessed and risk reduced. Students will be expected to learn the rudimentary navigation of R Studio, execute pre-written publically available R code (provided), and make simple modifications. Graduate students will be expected to learn the above and develop a 10 week modeling project focused on the use of spatial modeling methods with R using data relevant to their specific discipline or interest. These projects will include preparing datasets to be executed in R code scripts. Resulting scripts will be placed in a git repository for use by other students as open source resources along with documentation demonstrating the reproducible spatial modeling science and analyses for these problems. Geostatistical (spatial) mapping is applicable across many disciplines. Examples of graduate projects from previous classes include subsurface modeling (geology), earthquake mapping (geophysics/civil engineering), soil stability modeling (civil engineering), aquifer characterization (hydrology), and pollution/contaminant mapping (environmental studies/medicine). Offered as DSCI 332 and DSCI 432 .
DSCI 351. Exploratory Data Science. 3 Units.
In this course, we will learn data science and analysis approaches to identify statistically significance relationships and better model and predict the behavior of these systems. We will assemble and explore real-world datasets, perform clustering and pair plot analyses to investigate correlations, and logistic regression will be employed to develop associated predictive models. Results will be interpreted, visualized and discussed. We will introduce basic elements of statistical analysis using R Project open source software for exploratory data analysis and model development. R is an open-source software project with broad abilities to access machine-readable open-data resources, data cleaning and munging functions, and a rich selection of statistical packages, used for data analytics, model development and prediction. This will include an introduction to R data types, reading and writing data, looping, plotting and regular expressions, so that one can start performing variable transformations for linear fitting and developing structural equation models, while exploring for statistically significant relationships. The M section of DSCI 351 is for students focusing on Materials Data Science. Offered as DSCI 351 , DSCI 351M and DSCI 451 . Prereq: ( ENGR 130 or ENGR 131 or CSDS 132 or ECSE 132 or DSCI 134) and ( STAT 312R or STAT 201R or SYBB 310 or PQHS 431 ).
DSCI 351M. Exploratory Data Science. 3 Units.
DSCI 352. Applied Data Science Research. 3 Units.
This is a project based data science research class, in which project teams identify a research project under the guidance of a domain expert professor. The research is structured as a data analysis project including the 6 steps of developing a reproducible data science project, including 1: Define the ADS question, 2: Identify, locate, and/or generate the data 3: Exploratory data analysis 4: Statistical modeling and prediction 5: Synthesizing the results in the domain context 6: Creation of reproducible research, Including code, datasets, documentation and reports. During the course special topic lectures will include Ethics, Privacy, Openness, Security, Ethics. Value. The M section of DSCI 352 is for students focusing on Materials Data Science. Offered as DSCI 352 , DSCI 352M and DSCI 452 . Prereq: ( CSDS 133 or DSCI 134 or ENGR 130 or ENGR 131 or CSDS 132 or ECSE 132 ) and ( STAT 312R or STAT 201R or SYBB 310 or PQHS 431 or OPRE 207 ) and ( DSCI 351 or ( SYBB 311A and SYBB 311B and SYBB 311C and SYBB 311D) or SYBB 321 or MKMR 201 ).
DSCI 352M. Applied Data Science Research. 3 Units.
DSCI 353. Data Science: Statistical Learning, Modeling and Prediction. 3 Units.
In this course, we will use an open data science tool chain to develop reproducible data analyses useful for inference, modeling and prediction of the behavior of complex systems. In addition to the standard data cleaning, assembly and exploratory data analysis steps essential to all data analyses, we will identify statistically significant relationships from datasets derived from population samples, and infer the reliability of these findings. We will use regression methods to model a number of both real-world and lab-based systems producing predictive models applicable in comparable populations. We will assemble and explore real-world datasets, use pair-wise plots to explore correlations, perform clustering, self-similarity, and logistic regression develop both fixed-effect and mixed-effect predictive models. We will introduce machine-learning approaches for classification and tree-based methods. Results will be interpreted, visualized and discussed. We will introduce the basic elements of data science and analytics using R Project open source software. R is an open-source software project with broad abilities to access machine-readable open-data resources, data cleaning and assembly functions, and a rich selection of statistical packages, used for data analytics, model development, prediction, inference and clustering. With this background, it becomes possible to start performing variable transformations for linear regression fitting and developing structural equation models, fixed-effects and mixed-effects models along with other statistical learning techniques, while exploring for statistically significant relationships. The class will be structured to have a balance of theory and practice. We'll split class into Foundation and Practicum a) Foundation: lectures, presentations, discussion b) Practicum: coding, demonstrations and hands-on data science work. The M section of DSCI 353 is for students focusing on Materials Data Science. Offered as DSCI 353 , DSCI 353M and DSCI 453 .
DSCI 353M. Data Science: Statistical Learning, Modeling and Prediction. 3 Units.
DSCI 354. Data Visualization and Analytics. 3 Units.
Data Visualization and Analytics students will learn data visualization and analytics techniques focused on different types of data such as time-series, spectral, or image data science problems. This class will focus on increasing analysis of complex data sets through visualization by enhancing exploratory data analysis and data cleaning. This class will focus on creating effective data visualizations to communicate data analytics results to different audiences. Different datasets will be provided to develop different types of visualizations and analytics. Types of data visualizations include in interactive plots (e.g., bar graphs change over time), applications that allow users to adjust the visualizations based on their decisions (e.g., shiny applications), interactive maps, 3-D plots of data, etc. Discussing how an audience understands information and brings in data as well as the ethics of making data visualizations will be discussed. The class will also include ways to increase modeling and analysis with effective visualizations for credible, data-driven decision making. This will include a git repository for other students to use these codes as open source resources and the preparation of reproducible data science analyses for different types of problems. Offered as DSCI 354 , DSCI 354M , and DSCI 454 . Prereq: ( DSCI 351 or DSCI 351M ) and ( DSCI 353 or DSCI 353M ).
DSCI 354M. Data Visualization and Analytics. 3 Units.
DSCI 430. Cognition and Computation. 3 Units.
DSCI 432. Spatial Statistics for Near Surface, Surface, and Subsurface Modeling. 3 Units.
DSCI 451. Exploratory Data Science. 3 Units.
In this course, we will learn data science and analysis approaches to identify statistically significance relationships and better model and predict the behavior of these systems. We will assemble and explore real-world datasets, perform clustering and pair plot analyses to investigate correlations, and logistic regression will be employed to develop associated predictive models. Results will be interpreted, visualized and discussed. We will introduce basic elements of statistical analysis using R Project open source software for exploratory data analysis and model development. R is an open-source software project with broad abilities to access machine-readable open-data resources, data cleaning and munging functions, and a rich selection of statistical packages, used for data analytics, model development and prediction. This will include an introduction to R data types, reading and writing data, looping, plotting and regular expressions, so that one can start performing variable transformations for linear fitting and developing structural equation models, while exploring for statistically significant relationships. The M section of DSCI 351 is for students focusing on Materials Data Science. Offered as DSCI 351 , DSCI 351M and DSCI 451 .
DSCI 452. Applied Data Science Research. 3 Units.
This is a project based data science research class, in which project teams identify a research project under the guidance of a domain expert professor. The research is structured as a data analysis project including the 6 steps of developing a reproducible data science project, including 1: Define the ADS question, 2: Identify, locate, and/or generate the data 3: Exploratory data analysis 4: Statistical modeling and prediction 5: Synthesizing the results in the domain context 6: Creation of reproducible research, Including code, datasets, documentation and reports. During the course special topic lectures will include Ethics, Privacy, Openness, Security, Ethics. Value. The M section of DSCI 352 is for students focusing on Materials Data Science. Offered as DSCI 352 , DSCI 352M and DSCI 452 .
DSCI 453. Data Science: Statistical Learning, Modeling and Prediction. 3 Units.
DSCI 454. Data Visualization and Analytics. 3 Units.
Data Visualization and Analytics students will learn data visualization and analytics techniques focused on different types of data such as time-series, spectral, or image data science problems. This class will focus on increasing analysis of complex data sets through visualization by enhancing exploratory data analysis and data cleaning. This class will focus on creating effective data visualizations to communicate data analytics results to different audiences. Different datasets will be provided to develop different types of visualizations and analytics. Types of data visualizations include in interactive plots (e.g., bar graphs change over time), applications that allow users to adjust the visualizations based on their decisions (e.g., shiny applications), interactive maps, 3-D plots of data, etc. Discussing how an audience understands information and brings in data as well as the ethics of making data visualizations will be discussed. The class will also include ways to increase modeling and analysis with effective visualizations for credible, data-driven decision making. This will include a git repository for other students to use these codes as open source resources and the preparation of reproducible data science analyses for different types of problems. Offered as DSCI 354 , DSCI 354M , and DSCI 454 . Prereq: DSCI 451 and DSCI 453 .
EMSE 102. Materials for Current and Future Technologies. 1 Unit.
Open to all students discussing the importance of materials on current and future technologies. The course will be a series of seminars by the faculty at the Department of Materials Science and Engineering covering important topics such as materials processing, use of materials in a variety of technologically important areas; e.g., construction, energy related technologies, biomedical applications and space applications.
EMSE 110. Transitioning Ideas to Reality I - Materials in Service of Industry and Society. 1 Unit.
In order for ideas to impact the lives of individuals and society they must be moved from "blue sky" to that which is manufacturable. Therein lies true creativity - design under constraint. Greater Cleveland is fortunate to have a diverse set of industries that serve medical, aerospace, electric, and advanced-materials technologies. This course involves trips to an array of work sites of leading companies to witness first-hand the processes and products, and to interact directly with practitioners. Occasional in-class speakers with demonstrations will be used when it is not logistically reasonable to visit off-site.
EMSE 120. Transitioning Ideas to Reality II - Manufacturing Laboratory. 2 Units.
This course complements EMSE 110 . In that class students witness a diverse array of processing on-site in industry. In this class students work in teams and as individuals within processing laboratories working with an array of "real materials" to explore the potential of casting, machining, and deformation processes to produce real parts and/or components. An introduction to CAD as a means of communication is provided. The bulk of the term is spent in labs doing hands-on work. Planned work is carried out to demonstrate techniques and potential. Students have the opportunity to work independently or in teams to produce articles as varied as jewelry, electronics, transportation vehicles, or novel components or devices of the students' choosing.
EMSE 125. First Year Research in Materials Science and Engineering. 1 Unit.
First year students conduct independent research in the area of material science and engineering, working closely with graduate student(s) and/or postdoctoral fellow(s), and supervised by an EMSE faculty member. An average of 5-6 hr/wk in the laboratory, periodic updates, and an end of semester report is required. Prereq: Limited to first year undergraduate students..
EMSE 220. Materials Laboratory I. 2 Units.
Experiments designed to introduce processing, microstructure and property relationships of metal alloys and ceramics. Solidification of a binary alloy and metallography by optical and scanning electron microscopy. Synthesis of ceramics powders, thermal analysis using thermogravimetric analysis and differential thermal analysis, powder consolidation. Kinetics of high-temperature sintering, grain growth, and metal oxidation. Statistical analysis of experimental results. Recommended preparation or recommended co-requisite: EMSE 276 . This course satisfies the GER Disciplinary Communication requirement only in combination with EMSE 320 . Counts as a Disciplinary Communication course.
EMSE 228. Mathematical and Computational Methods for Materials Science and Engineering. 3 Units.
The course combines fundamental topics of material science and engineering with underlying mathematical methods and coding for computation. Focusing on the mathematics of vectors and using Mathematica as computational framework, the course teaches how to solve problems drawn from crystallography, diffraction, imaging of materials, and image processing. Students will develop a fundamental understanding of the basis for solving these problems including understanding the constituent equations, solution methods, and analysis and presentation of results. Prereq: ( ENGR 130 or ENGR 131 or CSDS 132 or ECSE 132 ) and ( ENGR 145 or CHEM 106 or PHYS 221 ).
EMSE 276. Materials Properties: Composition and Structure. 3 Units.
Relation of crystal structure, microstructure, and chemical composition to the selected properties of materials. The role materials processing has in controlling structure so as to obtain desired properties, using examples from metals, semiconductors, ceramics, and composites. Demonstration of properties determined by type and strength of interatomic bonding; presence of crystallographic defects; and microstructural control. Use of diagrams, frameworks, and mathematical relationships to evaluate and predict properties. A systematic review of characterization methods for assessing the state of different classes of materials will be provided throughout the course. Prereq: MATH 121 and ( CHEM 106 or PHYS 221 or ENGR 145 ). Prereq or Coreq: PHYS 122 or PHYS 124 .
EMSE 308. Welding Metallurgy. 3 Units.
Introduction to arc welding and metallurgy of welding. The course provides a broad overview of different industrial applications requiring welding, the variables controlling critical property requirements of the weld and a survey of the different types of arc welding processes. The course details the fundamental concepts that govern the different aspects of arc welding including the welding arc, weld pool solidification, precipitate formation and solid state phase transformations. Offered as EMSE 308 and EMSE 408 . Coreq: EMSE 327 .
EMSE 319. Processing and Manufacturing of Materials. 3 Units.
Introduction to processing technologies by which materials are manufactured into engineering components. Discussion of how processing methods are dependent on desired composition, structure, microstructure, and defects, and how processing affects material performance. Emphasis will be placed on processes and treatments to achieve or improve chemical, mechanical, physical performance and/or aesthetics, including: casting, welding, forging, cold-forming, powder processing of metals and ceramics, and polymer and composite processing. Coverage of statistics and computational tools relevant to materials manufacturing. Prereq: EMSE 276 .
EMSE 320. Materials Laboratory II. 1 Unit.
Introduce the design of microstructural characterization approaches to elucidate the structure and/or microstructure. It will include demonstrations of appropriate techniques for different material classes. The characterization is the enabler of the materials science and engineering triad: processing-structure-properties-performance. Students will reflect on how professional standards, statistical analysis, and instrument limitations require that engineers and scientists conduct failure analysis and interpret conclusions. Recommended preparation: EMSE 276 . Recommended corequisite: EMSE 372 . This course satisfies the GER Disciplinary Communication requirement only in combination with EMSE 220 . Counts as a Disciplinary Communication course.
EMSE 325. Undergraduate Research in Materials Science and Engineering. 1 - 3 Units.
Undergraduate laboratory research in materials science and engineering. Students will undertake an independent research project alongside graduate student(s) and/or postdoctoral fellow(s), and will be supervised by an EMSE faculty member. Written and oral reports will be given on a regular basis, and an end of semester report is required. The course can be repeated up to four (4) times for a total of six (6) credit hours. Prereq: Sophomore or Junior standing and consent of instructor.
EMSE 327. Thermodynamic Stability and Rate Processes. 3 Units.
An introduction to thermodynamics of materials as applied to metals, ceramics, polymers and optical/radiant heat transfer for photovoltaics. The laws of thermodynamics are introduced and the general approaches used in the thermodynamic method are presented. Systems studied span phase stability and oxidation in metals and oxides; nitride ceramics and semiconductors; polymerization, crystallization and block copolymer domain formation; and the thermodynamics of systems such as for solar power collection and conversion. Recommended preparation: EMSE 228 and ENGR 225 or equivalent. Prereq: EMSE 276 or EMSE 201.
EMSE 328. Mesoscale Structural Control of Functional Materials. 3 Units.
The course focuses on mesoscale structure of materials and their interrelated effects on properties, mostly in electrical in nature. The mesoscale science covers the structures varying from electronic- to micro-structure. In each scale, fundamental science will be complimented by examples of applications and how the structure is exploited both to modify and enable function. The student will develop an understanding of how the structure across multiple scales are interrelated and how to tailor them for desired outcomes. Offered as: EMSE 328 and EMSE 428 . Prereq: ( MATH 223 or MATH 227 ) and ( EMSE 276 or EMSE 201).
EMSE 330. Materials Laboratory III. 2 Units.
Experiments designed to characterize and evaluate different microstructures produced by variations in processing. Hardenability of steels, TTT and CT diagrams, precipitation hardening of alloys and fracture of brittle materials. Statistical analysis of experimental results. Recommended preparation: EMSE 276 . Recommended preparation or co-requisites: EMSE 327 .
EMSE 335. Strategic Metals and Materials for the 21st Century. 3 Units.
This course seeks to create an understanding of the role of mineral-based materials in the modern economy focusing on how such knowledge can and should be used in making strategic choices in an engineering context. The history of the role of materials in emerging technologies from a historical perspective will be briefly explored. The current literature will be used to demonstrate the connectedness of materials availability and the development and sustainability of engineering advances with examples of applications exploiting structural, electronic, optical, magnetic, and energy conversion properties. Processing will be comprehensively reviewed from source through refinement through processing including property development through application of an illustrative set of engineering materials representing commodities, less common metals, and minor metals. The concept of strategic recycling, including design for recycling and waste stream management will be considered. Offered as EMSE 335 and EMSE 435 . Prereq: Senior standing or graduate student.
EMSE 343. Processing of Electronic Materials. 3 Units.
The class will focus on the processing of materials for electronic applications. Necessary background into the fundamentals and applications will be given at the beginning to provide the basis for choices made during processing. MOSFET will be used as the target application. However, the processing steps covered are related to many other semiconductor based applications. The class will include both planar and bulk processing. Offered as: EMSE 343 and EMSE 443 . Prereq: ( PHYS 122 or PHYS 124 ) and EMSE 276 .
EMSE 345. Engineered Materials for Biomedical Applications. 3 Units.
A survey of synthetic biomedical materials from the perspective of materials science and engineering, focusing on how processing/synthesis, structure, and properties determine materials performance under the engineering demands imposed by physiological environments. Comparisons and contrasts between engineered metals, ceramics, and polymers, versus the biological materials they are called on to replace; consequences for materials and device design. Biomedical materials in applications such as orthopedic implants, dental restorations, wound healing, ophthalmic materials, and biomedical microelectromechanical systems (bioMEMS). Additive manufacturing of biomedical materials. Prereq: ENGR 200 and ENGR 145 .
EMSE 349. Role of Materials in Energy and Sustainability. 3 Units.
This course has two parts: engineered materials as consumers of resources (raw materials, energy); and as key contributors to energy efficiency and sustainable energy technologies. Topics covered include: Energy usage in the U.S. and the world. Availability of raw materials, including strategic materials; factors affecting global reserves and annual world production. Resource demand of materials production, fabrication, and recycling. Design strategies, and how the inclusion of environmental impacts in design criteria can affect design outcomes and material selection. Roles of engineered materials in energy technologies: photovoltaics, solar thermal, fuel cells, wind, batteries, capacitors. Materials in energy-efficient lighting. Energy return on energy invested. Semester projects will allow students to explore related topics (e.g. geothermal; biomass; energy-efficient manufacturing and transportation). Offered as EMSE 349 and EMSE 449 . Prereq: (ENGR 225 or EMAE 251 or EMAC 351 ) and ENGR 145 and ( PHYS 122 or PHYS 124 ) or Requisites Not Met permission.
EMSE 368. Thesis/Article Writing for Scientists and Engineers. 3 Units.
For writing a publication, such as a thesis or a journal article, in a field of science or engineering, students need a diverse set of skills in addition to mastering the scientific content. Generally, scientific writing requires proficiency in document organization, professional presentation of numerical and graphical data, literature retrieval and management, text processing, version control, graphical illustration, the English language, elements of style, etc. For scientists and engineers, specifically, writing a publication requires additional knowledge about e.g. conventions of numerical precision, error limits, mathematical typesetting, proper use of units, proper digital processing of micrographs, etc. Having to acquire these essential skills at the beginning of writing a thesis or a journal article may compromise the outcome by distracting from the most important task of composing the best possible scientific content. This course properly prepares students for scientific writing with a comprehensive spectrum of knowledge, skills, and tools enabling them to fully focus on the scientific content of their thesis or publication when the time has come to start writing. Similar to artistic drawing, where the ability to "see" is as (or more!) important as skills of the hand, the ability of proper scientific writing is intimately linked to the ability of critically reviewing scientific texts. Therefore, students will practice both authoring and critical reviewing of scientific texts. To sharpen students' skills of reviewing, examples of good and less good scientific writing will be taken from published literature in science and engineering and analyzed in the context of knowledge acquired in the course. At the end of the course, students will have set up skills and a highly functional work environment to start writing their role thesis or article with full focus on the scientific content. Students of all disciplines of science and engineering will benefit from the course material. Offered as EMSE 368 and EMSE 468 .
EMSE 372. Structural Materials by Design. 4 Units.
Materials selection and design of mechanical and structural elements with respect to static failure, elastic stability, residual stresses, stress concentrations, impact, fatigue, creep, and environmental conditions. Mechanical behavior of engineering materials (metals, polymers, ceramics, composites). Influence of ultrastructural and microstructural aspects of materials on mechanical properties. Mechanical test methods covered. Models of deformation behavior of isotropic and anisotropic materials. Methods to analyze static and fatigue fracture properties. Rational approaches to materials selection for new and existing designs of structures. Failure analysis methods and examples, and the professional ethical responsibility of the engineer. Four mandatory laboratories, with reports. Offered as EMAE 372 and EMSE 372 . Prereq: ENGR 200 .
EMSE 379. Design for Lifetime Performance. 3 Units.
The roles of processing and properties of a material on its performance, cost, maintenance, degradation and end-of-life treatment. Corrosion and oxidation, hydrogen and transformation-induced degradation mechanisms. Defects from prior processing. New defects generated during use/operation, e.g. generated from radiation, stress, heat etc. Accumulation and growth of defects during use/operation; crack nucleation, propagation, failure. Impact, abrasion, wear, corrosion/oxidation/reduction, stress-corrosion, fatigue, fretting, creep. Evaluation of degradation: Non-destructive versus destructive methods, estimation of remaining lifetime. Mitigation of degradation mechanisms. Statistical tools for assessing a material's lifetime performance. Capstone design project. Prereq: EMSE 372 . Coreq: EMSE 319 .
EMSE 396. Special Project or Thesis. 1 - 18 Units.
Special research projects or undergraduate thesis in selected material areas.
EMSE 398. Senior Project in Materials I. 1 Unit.
Independent Research project. Projects selected from those suggested by faculty; usually entail original research. The EMSE 398 and 399 sequence form an approved SAGES capstone. Counts as a SAGES Senior Capstone course.
EMSE 399. Senior Project in Materials II. 2 Units.
Independent Research project. Projects selected from those suggested by faculty; usually entail original research. Requirements include periodic reporting of progress, plus a final oral presentation and written report. Counts as a SAGES Senior Capstone course. Prereq: EMSE 398 .
EMSE 400T. Graduate Teaching I. 0 Unit.
To provide teaching experience for all Ph.D.-bound graduate students. This will include preparing exams/quizzes, homework, leading recitation sessions, tutoring, providing laboratory assistance, and developing teaching aids that include both web-based and classroom materials. Graduate students will meet with supervising faculty member throughout the semester. Grading is pass/fail. Students must receive three passing grades and up to two assignments may be taken concurrently. Recommended preparation: Ph.D. student in Materials Science and Engineering.
EMSE 408. Welding Metallurgy. 3 Units.
Introduction to arc welding and metallurgy of welding. The course provides a broad overview of different industrial applications requiring welding, the variables controlling critical property requirements of the weld and a survey of the different types of arc welding processes. The course details the fundamental concepts that govern the different aspects of arc welding including the welding arc, weld pool solidification, precipitate formation and solid state phase transformations. Offered as EMSE 308 and EMSE 408 .
EMSE 409. Deformation Processing. 3 Units.
Flow stress as a function of material and processing parameters; yielding criteria; stress states in elastic-plastic deformation; forming methods: forging, rolling, extrusion, drawing, stretch forming, composite forming.
EMSE 413. Fundamentals of Materials Engineering and Science. 3 Units.
Provides a background in materials for graduate students with undergraduate majors in other branches of engineering and science: reviews basic bonding relations, structure, and defects in crystals. Lattice dynamics; thermodynamic relations in multi-component systems; microstructural control in metals and ceramics; mechanical and chemical properties of materials as affected by structure; control of properties by techniques involving structure property relations; basic electrical, magnetic and optical properties.
EMSE 414. Electrical, Magnetic, Optical, and Thermal Properties of Materials. 3 Units.
Reviews quantum mechanics as applied to materials, energy bands, and density of states; Electrical properties of metals, semiconductors, insulators, and superconductors; Optical properties of materials, including: metallic luster, color, and optoelectronics; Magnetic properties of materials, including: Types of magnetic behavior, theory, and applications; Thermal properties of materials, including: heat capacity, thermal expansion, and thermal conductivity. Prereq: Graduate Standing in Materials Science and Engineering or Requisites Not Met permission.
EMSE 417. Properties of Materials in Extreme Environments. 3 Units.
Fundamentals of degradation pathways of materials under extreme conditions; thermodynamic stability of microstructures, deformation mechanisms, and failure mechanisms. Extreme conditions that will typically be addressed include: elevated temperatures, high-strain rates (ballistic), environmental effects, nuclear radiation, and small scales. Examples will be drawn from recent events as appropriate.
EMSE 421. Fracture of Materials. 3 Units.
Micromechanisms of deformation and fracture of engineering materials. Brittle fracture and ductile fracture mechanisms in relation to microstructure. Strength, toughness, and test techniques. Review of predictive models. Recommended preparation: ENGR 200 and EMSE 427 ; or consent.
EMSE 422. Failure Analysis. 3 Units.
Methods and procedures for determining the basic causes of failures in structures and components. Recognition of fractures and excessive deformations in terms of their nature and origin. Development and full characterization of fractures. Review of essential mechanical behavior concepts and fracture mechanics concepts applied to failure analyses in inorganic, organic, and composite systems. Legal, ethical, and professional aspects of failures from service. Prereq: EMSE 372 or EMAE 372 or Requisites Not Met permission.
EMSE 427. Defects in Solids. 3 Units.
Defects in solids control many properties of interest to the materials scientist or engineer. This course focuses on point, line, and interfacial defects in crystals and their interactions, including calculations of defect energies and interaction forces. Crystallographic defects presented include point defects (e.g., vacancies, interstitials, substitutional and interstitial impurities), line defects (e.g., dislocations), and planar defects (e.g., grain boundaries). The consequence of point defects on diffusion as well as on optical and electronic properties is discussed. Dislocation motion and dislocation dissociation are treated, and the influence of dislocation dynamics on yield phenomena, work hardening, and other mechanical properties are discussed. The role of grain boundaries and inter-phase boundaries in determining the physical properties of the material are presented. Experimental techniques for characterizing defects are integrated throughout the course. Recommended preparation: MATH 223 (or equivalent) and EMSE 276 (or equivalent).
EMSE 428. Mesoscale Structural Control of Functional Materials. 3 Units.
The course focuses on mesoscale structure of materials and their interrelated effects on properties, mostly in electrical in nature. The mesoscale science covers the structures varying from electronic- to micro-structure. In each scale, fundamental science will be complimented by examples of applications and how the structure is exploited both to modify and enable function. The student will develop an understanding of how the structure across multiple scales are interrelated and how to tailor them for desired outcomes. Offered as: EMSE 328 and EMSE 428 .
EMSE 435. Strategic Metals and Materials for the 21st Century. 3 Units.
EMSE 443. Processing of Electronic Materials. 3 Units.
EMSE 449. Role of Materials in Energy and Sustainability. 3 Units.
This course has two parts: engineered materials as consumers of resources (raw materials, energy); and as key contributors to energy efficiency and sustainable energy technologies. Topics covered include: Energy usage in the U.S. and the world. Availability of raw materials, including strategic materials; factors affecting global reserves and annual world production. Resource demand of materials production, fabrication, and recycling. Design strategies, and how the inclusion of environmental impacts in design criteria can affect design outcomes and material selection. Roles of engineered materials in energy technologies: photovoltaics, solar thermal, fuel cells, wind, batteries, capacitors. Materials in energy-efficient lighting. Energy return on energy invested. Semester projects will allow students to explore related topics (e.g. geothermal; biomass; energy-efficient manufacturing and transportation). Offered as EMSE 349 and EMSE 449 . Prereq: ENGR 225 and ( ENGR 145 or EMSE 146) and ( PHYS 122 or PHYS 124 ) or requisites not met permission.
EMSE 463. Magnetism and Magnetic Materials. 3 Units.
This course covers the fundamentals of magnetism and application of modern magnetic materials especially for energy and data storage technologies. The course will focus on intrinsic and extrinsic magnetic properties, processing of magnetic materials to achieve important magnetic performance metrics, and the state-of-the-art magnetic materials used today. The topics related to intrinsic properties, include: magnetic dipole moments, magnetization, exchange coupling, magnetic anisotropy and magnetostriction. Topics related to extrinsic properties, include: magnetic hysteresis, frequency dependent magnetic response and magnetic losses. Technologically important permanent magnets (including rare earth containing alloys and magnetic oxides), soft magnets (including electrical steels, amorphous, ferrites, and nanocrystalline alloys), and thin film materials (including iron platinum) will be discussed in the context of their technological interest. Throughout the course, experimental techniques and data analysis will be discussed. The course is suitable for most graduate students and advanced undergraduates in engineering and science.
EMSE 468. Thesis/Article Writing for Scientists and Engineers. 3 Units.
EMSE 499. Materials Science and Engineering Colloquium. 0 Unit.
Invited speakers deliver lectures on topics of active research in materials science. Speakers include researchers at universities, government laboratories, and industry. Course is offered only for 0 credits. Attendance is required.
EMSE 500T. Graduate Teaching II. 0 Unit.
To provide teaching experience for all Ph.D.-bound graduate students. This will include preparing exams/quizzes/homework, leading recitation sessions, tutoring, providing laboratory assistance, and developing teaching aids that include both web-based and classroom materials. Graduate students will meet with supervising faculty member throughout the semester. Grading is pass/fail. Students must receive three passing grades and up to two assignments may be taken concurrently. Recommended preparation: Ph.D. student in Materials Science and Engineering.
EMSE 503. Structure of Materials. 3 Units.
The structure of materials and physical properties are explored in terms of atomic bonding and the resulting crystallography. The course will cover basic crystal chemistry, basic crystallography (crystal symmetries, point groups, translation symmetries, space lattices, and crystal classes), basic characterization techniques and basic physical properties related to a materials structure.
EMSE 504. Thermodynamics of Solids. 3 Units.
Review of the first, second, and third laws of thermodynamics and their consequences. Stability criteria, simultaneous chemical reactions, binary and multi-component solutions, phase diagrams, surfaces, adsorption phenomena.
EMSE 505. Phase Transformations, Kinetics, and Microstructure. 3 Units.
Phase diagrams are used in materials science and engineering to understand the interrelationships of composition, microstructure, and processing conditions. The microstructure and phases constitution of metallic and nonmetallic systems alike are determined by the thermodynamic driving forces and reaction pathways. In this course, solution thermodynamics, the energetics of surfaces and interfaces, and both diffusional and diffusionless phase transformations are reviewed. The development of the laws of diffusion and its application for both melts and solids are covered. Phase equilibria and microstructure in multicomponent systems will also be discussed.
EMSE 515. Analytical Methods in Materials Science. 3 Units.
Microcharacterization techniques of materials science and engineering: SPM (scanning probe microscopy), SEM (scanning electron microscopy), FIB (focused ion beam) techniques, SIMS (secondary ion mass spectrometry), EPMA (electron probe microanalysis), XPS (X-ray photoelectron spectrometry), and AES (Auger electron spectrometry), ESCA (electron spectrometry for chemical analysis). The course includes theory, application examples, and laboratory demonstrations.
EMSE 599. Critical Review of Materials Science and Engineering Colloquium. 1 - 2 Units.
Invited speakers deliver lectures on topics of active research in materials science. Speakers include researchers at universities, government laboratories, and industry. Each course offering is for 1 or 2 credits but the course can be taken multiple times totaling up to a maximum of six credits. Attendance is required. Graded coursework is in the form of a term paper per credit. The topic for the term paper(s) should be chosen from seminar topics. The term paper will be graded by the advisor of the graduate student.
EMSE 600T. Graduate Teaching III. 0 Unit.
To provide teaching experience for all Ph.D.-bound graduate students. This will include preparing exam/quizzes/homework, leading recitation sessions, tutoring, providing laboratory assistance, and developing teaching aids that include both web-based and classroom materials. Graduate students will meet with supervising faculty member throughout the semester. Grading is pass/fail. Students must receive three passing grades and up to two assignments may be taken concurrently. Recommended preparation: Ph.D. student in Materials Science and Engineering.
EMSE 601. Independent Study. 1 - 18 Units.
EMSE 634. Special Topics of Materials Science. 1 - 3 Units.
This course introduces graduate students to specific topics of material science, tailored to individual interests of the students. For example, students with interest in specific techniques for microcharacterization of materials may be educated in the physical background of these techniques by studying literature under the guidance of the instructor, presenting and discussing the learned material with the instructor and other students, and being trained in practical experimentation in laboratory sessions demonstrating these techniques on instruments of SCSAM, the Swagelok Center for Surface Analysis of Materials.
EMSE 649. Special Projects. 1 - 18 Units.
EMSE 651. Thesis M.S.. 1 - 18 Units.
Required for Master's degree. A research problem in metallurgy, ceramics, electronic materials, biomaterials or archeological and art historical materials, culminating in the writing of a thesis.
EMSE 695. Project M.S.. 1 - 9 Units.
Research course taken by Plan B M.S. students. Prereq: Enrolled in the EMSE Plan B MS Program.
EMSE 701. Dissertation Ph.D.. 1 - 9 Units.
Required for Ph.D. degree. A research problem in metallurgy, ceramics, electronic materials, biomaterials or archeological and art historical materials, culminating in the writing of a thesis. Prereq: Predoctoral research consent or advanced to Ph.D. candidacy milestone.
Print Options
Print this page.
The PDF will include all information unique to this page.
Mechanical Behavior of Multi-fracturing Under Confining Pressure: Effects of Borehole Diameter and Rock Type
- Original Paper
- Published: 11 September 2024
Cite this article
- Dongdong Ma 1 ,
- Yu Wu ORCID: orcid.org/0000-0002-6217-2874 1 , 2 ,
- Haozhe Geng 1 ,
- Xiao Ma 3 ,
- Yiqun Zhang 2 ,
- Hai Pu 1 , 2 &
- Lingyu Li 4
The tensile strength of rock materials, a crucial factor for the stability of underground engineering, is much lower than its compressive strength. Moreover, the tensile strength of rock under confining pressure differs significantly from that of unconfined conditions, presenting a compelling avenue for investigation. In this work, the tensile strength of rock under confining pressure was tested using the multi-fracturing method. The effects of borehole diameter (i.e., 6, 8, 10, and 12 mm for granite) of hydraulic fracturing and rock type (i.e., granite, sandstone, and coal) on tensile strength were investigated, the evolution of tensile strength with confining pressure was elaborated, and its application in the failure criterion was elucidated. The results demonstrated that the breakdown pressure of hydraulic fracturing decreased with the borehole diameter at low confining pressure (i.e., σ 3 = 2, 5 MPa), while the variation was minimal for high confining pressure (i.e., σ 3 = 20, 30 MPa). Tensile strength exhibited a bulging phenomenon in response to changes in confining pressure, increasing to a bulging point and then gradually decreasing, with more pronounced changes observed in sandstone and coal. The pre-failure stress state σ 1 > σ 2 > σ 3 at low confining pressure gradually converted toward σ 1 > σ 2 = σ 3 at high confining pressure, contributing to the evolution of tensile strength into a bulging phenomenon. Furthermore, the tensile stress regime predicted by the Hoek–Brown criterion coincided with experimental data, with minor deviations for sandstone and granite. The researches facilitate a further understanding of the tensile properties in underground engineering.
Tensile strength of rock first in creased and then decreased with confining pressure.
Changes in stress state with confining pressure contributed to tensile strength in a 38 bulging phenomenon.
The Hoek Brown criterion was applicable for the determination of tensile strength 40 under confined pressure.
This is a preview of subscription content, log in via an institution to check access.
Access this article
Subscribe and save.
- Get 10 units per month
- Download Article/Chapter or eBook
- 1 Unit = 1 Article or 1 Chapter
- Cancel anytime
Price includes VAT (Russian Federation)
Instant access to the full article PDF.
Rent this article via DeepDyve
Institutional subscriptions
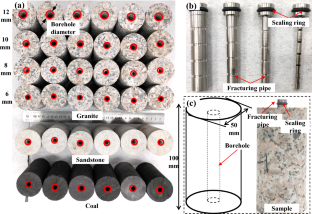
Data availability
The raw/processed data required to reproduce the above findings cannot be shared at this time as the data also forms part of an ongoing study.
Akazawa T (1943) New test method for evaluating internal stress due to compression of concrete: the splitting tension test. J Jpn Soc Civ Eng 29:777–787
Google Scholar
Aliha MRM, Ebneabbasi P, Karimi H, Nikbakht E (2021) A novel test device for the direct measurement of tensile strength of rock using ring shape sample. Int J Rock Mech Min Sci 139:104649
Article Google Scholar
Andrews BJ, Cumberpatch ZA, Shipton ZK, Lord R (2020) Collapse processes in abandoned pillar and stall coal mines: implications for shallow mine geothermal energy. Geothermics 88:101904
Berrone S, Busetto M, Vicini F (2023) Virtual element simulation of two-phase flow of immiscible fluids in discrete fracture networks. J Comput Phys 473:111735
Bobich JK (2005) Experimental analysis of the extension to shear fracture transition in Berea sandstone. Texas A&M University, college station (MS thesis)
Cai M (2010) Practical estimates of tensile strength and Hoek–Brown strength parameter mi of brittle rocks. Rock Mech Rock Eng 43:167–184
Carneiro F. (1943) A new method to determine the tensile strength of concrete. In: proceedings of the the 5th meeting of the Brazilian association for technical rules, vol 3, p 126–129
Cheng M, Fu X, Chen Z, Liu T, Zhang M, Kang J (2023) A new approach to evaluate abandoned mine methane resources based on the zoning of the mining-disturbed strata. Energy 274:127307
Article CAS Google Scholar
Cui P, Ge Y, Li S, Li Z, Xu X, Zhou GGD, Chen H, Wang H, Lei Y, Zhou L, Yi S, Wu C, Guo J, Wang Q, Lan H, Ding M, Ren J, Zeng L, Jiang Y, Wang Y (2022) Scientific challenges in disaster risk reduction for the Sichuan–Tibet railway. Eng Geol 309:106837
Davoodi S, Al-Shargabi M, Wood DA, Rukavishnikov VS (2023) A comprehensive review of beneficial applications of viscoelastic surfactants in wellbore hydraulic fracturing fluids. Fuel 338:127228
Fairhurst C (1961) Laboratory measurement of some physical properties of rock. 4 th symposium on rock mechanics. University Park Penn, p 105–118
Fu C, Liu N (2019) Waterless fluids in hydraulic fracturing-a review. J Nat Gas Sci Eng 67:214–224
Fuenkajorn K, Klanphumeesri S (2010) Direct tension tests of intact rocks using compression-to-tension load converter. Res Dev J 21(2):51–57
Ghazvinian A, Fathi A, Moradian Z (2008) Failure behavior of marlstone under triaxial compression. Int J Rock Mech Min Sci 5:807–814
Goldstein RV, Vaishelbaum VM (1978) Material scale length as a measure of fracture toughness in fracture mechanics of plastic materials. Int J Fract 14(2):185–201
Goto R, Sakaguchi K, Parisio F, Ke Y, Pramudyo E, Watanabe N (2022) Wellbore stability in high-temperature granite under true triaxial stress. Geothermics 100:102334
Guo F, Morgenstern NR, Scott JD (1993) Interpretation of hydraulic fracturing pressure: a comparison of eight methods used to identify shut-in pressure. Int J Rock Mech Min Sci Geomech Abstr 30(6):627–631
Guo T, Liu B, Qu Z, Gong D, Xin L (2017) Study on initiation mechanisms of hydraulic fracture guided by vertical multi-radial boreholes. Rock Mech Rock Eng 50:1767–1785
Guo T, Tang S, Liu S, Liu X, Zhang W, Qu G (2020) Numerical simulation of hydraulic fracturing of hot dry rock under thermal stress. Eng Fract Mech 240:107350
Guo W, Guo Y, Yang H, Wang L, Liu B, Yang C (2022) Tensile mechanical properties and AE characteristics of shale in triaxial Brazilian splitting tests. J Petrol Sci Eng 219:111080
Heider Y (2021) A review on phase-field modeling of hydraulic fracturing. Eng Fract Mech 253:107881
Hoek E (1964) Fracture of anisotropic rock. J S Afr Inst Min Metall 64(10):501–518
Hou P, Xue Y, Gao F, Dou F, Su S, Cai C, Zhu C (2022) Effect of liquid nitrogen cooling on mechanical characteristics and fracture morphology of layer coal under Brazilian splitting test. Int J Rock Mech Min Sci 151:105026
Huang D, Liu Y, Cen D, Zeng B, Wu Z, Yang Y (2022) Effect of confining pressure on deformation and strength of granite in confined direct tension tests. Bull Eng Geol Env 81:110
Huang D, Liu Y, Cen D, Peng J, Zeng B (2023) Mechanical responses of granite under confined direct tensile testing: fracture, strength, energy conversion and mechanism. Rock Mech Rock Eng 56:143–166
Hubbert MK, Willis DG (1957) Mechanics of hydraulic fracturing. Pet. Trans. AIME 210:153–168
Ignacio P, Andrea M, Manuel AG, Mauro M, Miguel HP, Xian E, Jordi D, Leandro RA (2023) Size effects on the tensile strength and fracture toughness of granitic rock in different tests. J Rock Mech Geotech Eng 15:2179–2192
ISRM (1978) Suggested methods for determining tensile strength of rock materials. Int J Rock Mech Min Sci Geomech Abstr 15:99–103
Kumari WGP, Ranjith PG, Perera MSA, Li X, Li LH, Chen BK, Avanthi Isaka BL, De Silva VRS (2018) Hydraulic fracturing under high temperature and pressure conditions with micro CT applications: geothermal energy from hot dry rocks. Fuel 230:138–154
Lan HX, Chen JH, Macciotta R (2019) Universal confined tensile strength of intact rock. Sci Rep 9:6170. https://doi.org/10.1038/s41598-019-42698-6
Landry CJ, Prodanović M, Karpyn Z, Eichhubl P (2024) Estimation of fracture permeability from aperture distributions for rough and partially cemented fractures. Transp Porous Media 151:689–717
Li Y, Ghassemi A (2021) Rock failure envelope and behavior using the confined Brazilian test. J Geophys Res Solid Earth 126:e2021JB022471
Li G, Sheng M, Tian S, Huang Z, Li Y, Yuan X (2012) Multistage hydraulic jet acid fracturing technique for horizontal wells. Pet Explor Develop 39(1):107–112
Li G, Wang K, Tang C, Qian X (2020) An NMM-based fluid-solid coupling model for simulating rock hydraulic fracturing process. Eng Fract Mech 235:107193
Li N, Wang C, Zhang S, Yu J, Kang J, Wang Y, Dai Y (2021) Recent advances in waterless fracturing technology for the petroleum industry: an overview. J Nat Gas Sci Eng 92:103999
Liao S, Hu J, Zhang Y (2022) Numerical evaluation of refracturing fracture deflection behavior under non-uniform pore pressure using XFEM. J Pet Sci Eng 219:111074
Lin C, Jia X, Deng S, Mao J, Chen X, He J, Li X (2024) The roles of micro pores and minerals in shale during hydraulic fracturing. Rock Mech Rock Eng. https://doi.org/10.1007/s00603-024-04063-6
Liu Z, Zhou H, Zhang W, Xie S, Shao J (2019) A new experimental method for tensile property study of quartz sandstone under confining pressure. Int J Rock Mech Min Sci 123:104091
Liu Y, Da H, Cen D, Zhong Z, Gong F, Wu Z, Yang Y (2021) Tensile strength and fracture surface morphology of granite under confined direct tension test. Rock Mech Rock Eng 54:4755–4769
Ma D, Cheng C, Ding C, Song J, Hu D, Zhou H (2021) Comparisons of fracturing mechanism of tight sandstone using liquid CO 2 and water. J National Gas Sci Eng 94:1875–5100
Ma D, Wu Y, Yin J, Lu J, Hu D, Zhou H (2023a) Effect of initial pore pressure on the hydraulic fracturing of tight sandstone: an experimental study. Geomech Geophys Geo Energ Geo-Resour 9:15
Ma D, Wu Y, Ma X, Hu D, Zhou H, Li D (2023b) A preliminary experimental and numerical study on the applicability of liquid CO 2 fracturing in sparse sandstone. Rock Mech Rock Eng 56:7315–7332
Ma D, Wu Y, Hu X, Li D, Geng H, Hao Y (2023c) DEM simulation of injection-induced micro-cracks behaviors in the heterogeneous glutenite by fluid–solid coupling. Comp Part Mech. https://doi.org/10.1007/s40571-023-00662-2
Ma D, Wu Y, Yin D, Ge Y, Hu D, Zhou H, Geng H (2024) Investigation on cracking propagation patterns in hydraulic fracturing under different vug distributions: experimental and numerical case. Eng Fract Mech. https://doi.org/10.1016/j.engfracmech.2024.109863
McDermott CI, Edlmann K, Haszeldine RS (2013) Predicting hydraulic tensile fracture spacing in strata-bound systems. Int J Rock Mech Min Sci 63:39–49
Meier T, Rybacki E, Reinicke A, Dresen G (2013) Influence of borehole diameter on the formation of borehole breakouts in black shale. Int J Rock Mech Min Sci 62:74–85
Mojid MR, Negash BM, Abdulelah H, Jufar SR, Adewumi BK (2021) A state-of-the-art review on waterless gas shale fracturing technologies. J Petrol Sci Eng 196:108048
Newman JC (1969) Stress analysis of simply and multiply connected regions containing cracks by the method of boundary collocation. Master dissertation, Virginia Polytechnic Institute
Obert L, Windes S, Duvall W (1946) Standardized tests for determining the physical properties of mine rock. U.S.B.M.R.I. 3891
Pariseau W (2007) Fitting failure criteria to laboratory strength tests. Int J Rock Mech Min Sci 4:637–646
Park D (2023) Influence of the Hoek–Brown failure criterion with tensile strength cut-off on the roof stability in deep rock tunnels. Tunn Undergr Space Technol 136:105016
Paul B (1961) A modification of the Coulomb–Mohr theory of fracture. J Appl Mech 28:259–268. https://doi.org/10.1115/1.3641665
Ren Y, Vavrycuk V, Wu S, Gao Y (2021) Accurate moment tensor inversion of acoustic emissions and its application to Brazilian splitting test. Int J Rock Mech Min Sci 141:104707
Rummel F (1987) Fracture mechanics approach to hydraulic fracturing stress measurements. In: Atkinson BK (ed) Fracture Mechanics of Rock. Academic Press, Cambridge, London, pp 217–239
Chapter Google Scholar
Sasaki T, Shao B, Elshafie M, Papadopoulou M, Yamamoto K, Soga K (2021) Simulation of axial tensile well deformation during reservoir compaction in offshore unconsolidated methane hydrate-bearing formation. Comput Geotech 129:103894
Schönhoff L, Mayinger F, Eichberger M, Lösch A, Reznikova E, Stawarczyk B (2022) Three-dimensionally printed and milled polyphenylene sulfone materials in dentistry: tensile bond strength to veneering composite resin and surface properties after different pretreatments. J Prosthet Dent 128(1):93–99
Song I, Suh M, Won KS, Haimson B (2001) A laboratory study of hydraulic fracturing breakdown pressure in tablerock sandstone. Geosci J 5(3):263–271
Song M, Li Q, Hu Q, Wu Y, Ni G, Xu Y, Zhang Y, Hu L, Shi J, Liu J, Deng Y (2022) Resistivity response of coal under hydraulic fracturing with different injection rates: a laboratory study. Int J Min Sci Technol 32:807–819
Su G, Ren H, Jiang J, Hu X (2023) Experimental study on the characteristics of rockburst occurring at the working face during tunnel excavation. Int J Rock Mech Min Sci 164:105347
Tanaka APB, Borges JPG, Matos GC, Campos MTR, Cunha BM, Souza RB, Caldeira JNM, Oliveira TAS, Marçon DR, Lima APM (2022) Fault-related fracture modeling in a pre-salt lacustrine carbonate reservoir from santos basin, offshore Brazil: predicting preferential fluid flow paths using 3D geological and flow simulation models. Mar Pet Geol 135:105392
Tang W, Zhai C, Yu X, Xu J, Sun Y, Cong Y, Zheng Y, Wang Y (2022) Dynamic Brazilian splitting experiment of bedding shale based on continuum-discrete coupled method. Int J Impact Eng 168:104289
Tang J, Song X, Lu Y (2023) An elastic-plastic kinking layer model for parallel-to-grain wood embedment behavior considering wood drilling damage. Eng Struct 280:115656
Vutukuri V, LamaSaluja RSS (1974) Handbook on Mechanical Properties of Rocks. Trans Tech Publications, Ohio
Wang K, Ma Q, Xu J, He A, Wang P, Chen R, Guo J, Li L (2022) Theoretical study of laminar plasma quenching of rail steel using the fluid-solid-thermal coupling method. Int J Therm Sci 179:107708
Wang F, Zhou M, Shen W, Huang H, He J (2023a) Fluid-solid-phase multi-field coupling modeling method for hydraulic fracture of saturated brittle porous materials. Eng Fract Mech 286:109231
Wang X, Sun Y, Li B, Zhang G, Guo W, Li S, Jiang S, Peng S, Chen H (2023b) Reservoir stimulation of marine natural gas hydrate-a review. Energy 263:126120
Wang T, Wei Y, Ou S, Jin L, Wang S, Liu J, Zhang G, Zhou Y, Lin M (2023c) Multistage cooling system for temperature reduction of the working face in deep coal mines: a technical-economic evaluation. Case Stud Thermal Eng 45:102908
Wanniarachchi WAM, Gamage RP, Perera MSA, Tharaka DR, Gao M, Eswaran P (2017) Investigation of depth and injection pressure effects on breakdown pressure and fracture permeability of shale reservoirs: an experimental study. Appl Sci 7:664
Wu N, Fu J, Zhu Z, Sun B (2020) Experimental study on the dynamic behavior of the Brazilian disc sample of rock material. Int J Rock Mech Min Sci 130:104326
Wu Y, Tao J, Wang J, Zhang Y, Peng S (2021) Experimental investigation of shale breakdown pressure under liquid nitrogen pre-conditioning before nitrogen fracturing. Int J Min Sci Technol 31:611–620
Xu W, Yu H, Zhang J, Lyu C, Wang Q, Micheal M, Wu H (2022) Phase-field method of crack branching during SC-CO 2 fracturing: a new energy release rate criterion coupling pore pressure gradient. Comput Methods Appl Mech Engrg 399:115366
Yan H, Wang W, Zhang J, Ma D, Zhou N, Wan Z (2023) Experimental study on the influence of coal-rock interface strength on crack propagation law of supercritical carbon dioxide fracturing. Gas Sci Eng 112:204943
Yang Z, Yang S, Zheng W, Tannant DD (2022) Coupled thermal-hydraulic simulations of fracturing in granite under high temperature and high pressure treatment via peridynamic. Int J Rock Mech Min Sci 160:105247
You M (2015) Strength criterion for rocks under compressive-tensile stresses and its application. J Rock Mech Geotech Eng 7:434–439
Zeng C (2018) Study on mechanical characteristics and constitutive model of red sandstone during axial unloading-tensile under confining pressure. Chongqing University
Zhai C, Xu J, Liu S, Qin L (2018) Investigation of the discharge law for drill cuttings used for coal outburst prediction based on different borehole diameters under various side stresses. Powder Technol 325:396–404
Zhang H, Zhong Y, Zhang J, Zhang Y, Kuang J, Yang B (2020) Experimental research on deterioration of mechanical properties of carbonate rocks under acidified conditions. J Pet Sci Eng 185:106612
Zhang W, Wang C, Guo T, He J, Zhang L, Chen S, Qu Z (2021) Study on the cracking mechanism of hydraulic and supercritical CO 2 fracturing in hot dry rock under thermal stress. Energy 221:119886
Zhang D, Sun Z, Fang Q (2022) Scientific problems and research proposals for Sichuan–Tibet railway tunnel construction. Undergr Space 7:419–439
Zhang X, Si G, Oh J, Zhao G (2024) Evolution of crack source mechanisms in laboratory hydraulic fracturing on harcourt granite. Rock Mech Rock Eng. https://doi.org/10.1007/s00603-024-03966-8
Zhao C, Li J, Jin Y, Zaman M, Miao Y (2021) Investigation of dynamic pore pressure in shale gas reservoir during the multi-fracturing and its influence on fault slip. J Nat Gas Sci Eng 95:104190
Zhao H, Xiong Y, Zhen H, Liu C, Li X (2022) Experimental investigation on the fracture propagation of three-stage acid fracturing of tight sandstone gas reservoir. J Pet Sci Eng 211:110143
Zhu Y, Liu C, Liu H, Kou Y, Bin S (2023) A multi-field and fluid–solid coupling method for porous media based on DEM-PNM. Comput Geotech 154:105118
Zhuang D, Yin T, Li Q, Wu Y, Tan X (2022) Effect of injection flow rate on fracture toughness during hydraulic fracturing of hot dry rock (HDR). Eng Fract Mech 260:108207
Download references
Acknowledgements
This work was supported by the Funded by the Postgraduate Research & Practice Innovation Program of Jiangsu Province (KYCX24_2706), funded by the Graduate Innovation Program of China University of Mining and Technology (2024WLKXJ203), and National Key Research and Development Program of China (Grant No. 2023YFC3804205)
Funded by the Postgraduate Research & Practice Innovation Program of Jiangsu Province, KYCX24_2706, Dongdong Ma, Funded by the Graduate Innovation Program of China University of Mining and Technology, 2024WLKXJ203, Dongdong Ma, National Key Research and Development Program of China, 2023YFC3804205, Yu Wu
Author information
Authors and affiliations.
State Key Laboratory for Geomechanics and Deep Underground Engineering, China University of Mining and Technology, Xuzhou, 221116, Jiangsu, China
Dongdong Ma, Yu Wu, Haozhe Geng & Hai Pu
School of Mechanics and Civil Engineering, China University of Mining and Technology, Xuzhou, 221116, Jiangsu, China
Yu Wu, Yiqun Zhang & Hai Pu
State Key Laboratory of Structural Health and Safety of Bridges, Wuhan, 430034, Hubei, China
State Key Laboratory of Geomechanics and Geotechnical Engineering, Institute of Rock and Soil Mechanics, Chinese Academy of Sciences, Wuhan, 430071, Hubei, China
You can also search for this author in PubMed Google Scholar
Corresponding author
Correspondence to Yu Wu .
Ethics declarations
Conflict of interest.
The authors declare that they have no known competing financial interests or personal relationships that could have appeared to influence the work reported in this paper.
Additional information
Publisher's note.
Springer Nature remains neutral with regard to jurisdictional claims in published maps and institutional affiliations.
Rights and permissions
Springer Nature or its licensor (e.g. a society or other partner) holds exclusive rights to this article under a publishing agreement with the author(s) or other rightsholder(s); author self-archiving of the accepted manuscript version of this article is solely governed by the terms of such publishing agreement and applicable law.
Reprints and permissions
About this article
Ma, D., Wu, Y., Geng, H. et al. Mechanical Behavior of Multi-fracturing Under Confining Pressure: Effects of Borehole Diameter and Rock Type. Rock Mech Rock Eng (2024). https://doi.org/10.1007/s00603-024-04145-5
Download citation
Received : 23 January 2024
Accepted : 18 August 2024
Published : 11 September 2024
DOI : https://doi.org/10.1007/s00603-024-04145-5
Share this article
Anyone you share the following link with will be able to read this content:
Sorry, a shareable link is not currently available for this article.
Provided by the Springer Nature SharedIt content-sharing initiative
- Tensile strength
- Confining pressure
- Borehole diameter
- Stress state
- Failure criterion
- Find a journal
- Publish with us
- Track your research
Physical Review B
Covering condensed matter and materials physics.
- Collections
- Editorial Team
- Accepted Paper
Tuning ferromagnetic superexchange interactions through cation disorder engineering in two-dimensional semiconductor alloys: A case study of CrMoSe 2 Br 2
Phys. rev. b, hongxu luo and sai lyu.
For two-dimensional ferromagnetic semiconductors, robust ferromagnetic couplings and high Curie temperatures are highly desirable. The alloying strategy has been proposed to be able to strengthen ferromagnetic superexchange interactions and enhance Curie temperatures. This strategy has been applied and examined in ordered alloy structures in many recent studies. However, the efficacy of the alloying strategy in the other two distinct types of alloy structures (i.e., partially ordered and random) has not been studied yet. In addition, the effects of cation disorder on the strength of ferromagnetic superexchange interactions and the Curie temperatures in two-dimensional ferromagnetic semiconductor alloys remain to be clarified. Exemplified by the CrMoSe 2 Br 2 alloy, we computationally study the ferromagnetic superexchange interactions in the ordered, partially ordered, and random alloy structures. For the CrMoSe 2 Br 2 alloy structure, a local motif is defined to be consisting of eight nearest-neighboring cations surrounding a given cation site. The large variations in the Curie temperatures for the ordered and random alloy structures are attributed to the different types of local motifs. In partially ordered alloy structures, the strength of superexchange interactions and the Curie temperatures show monotonic behaviors as functions of the degree of cation disorder. This work validates the efficacy of the alloying strategy in strengthening ferromagnetic superexchange interactions and enhancing Curie temperatures in all the three kinds of the alloy structures and demonstrates that cation disorder engineering can be a general approach to tune ferromagnetic superexchange interactions in two-dimensional semiconductor alloys.
Sign up to receive regular email alerts from Physical Review B
- Forgot your username/password?
- Create an account
Article Lookup
Paste a citation or doi, enter a citation.

IMAGES
VIDEO
COMMENTS
About the journal. Case Studies in Construction Materials provides a forum for the rapid publication of short, structured Case Studies on construction materials and related Short Communications, specialising in actual case studies involving real construction projects. Now by popular demand, CSCM has expanded the …. View full aims & scope.
Prof. Kimerling will lead a short in-class discussion on the approach for each Case Study or Project. Q&A is encouraged to clarify specific details. The Instructors and TAs will moderate online Discussion Forums: within this Forum students should post their team's tentative outline, develop concepts, discuss sources and preliminary findings.
An excellent case study is the growth of material science at Nankai University, one of China's most prestigious. Built on its strengths in chemistry and physics, Nankai formally established a ...
Introducing state-of-the art computational methods, this book combines detailed explanations with real-world case studies to give a full grounding in the design of engineering materials. This book presents a wide spectrum of key computational methods, such as CALPHAD-method, first-principles calculations, phase-field simulation and finite ...
The material can also be prone to insect attacks and has a limited lifespan, making it important to include proactive 'preservation by design' approaches as an integral part of any bamboo-based ...
design of engineering materials and other materials, being also an invaluable reference to graduates, undergraduates, researchers, and engineers who use various computa-tional tools in their study, research, and/or development of materials. Yong Du received his PhD from Central South University (CSU) of China in 1992. From
and case studies, as detailed in Table 1. Perhaps . the most critical difference between examples and case studies is the role assumed by the student: in examples, learners are . followers. of thought and their role switches to . leaders. of thought in case studies. Thus, learners must access and operate at higher
29.3 Case Study 2: Materials for Skis and Sledge Runners .....437 29.4 Case Study 3: High-Friction Rubber.....438 Contents xi. CHAPTER 30 Final Case Study: Materials and Energy ... Engineering Materials 1: An Introduction to Properties, Applications, and Design, (2012). 978--08-096665-6
Corresponding author. Ms. Mary E. Caulfield Massachusetts Institute of Technology 77 Massachusetts Avenue 12-113 Cambridge, Massachusetts, USA 02139 1-617-894-0900 [email protected]. This work is licensed under a Creative Commons Attribution-NonCommercial-NoDerivs 3.0 Unported License.
This study aims to investigate the impact of Value Engineering on material selection in the construction industry. It is believed that VE can reduce construction project budgets by 10%-25% while maintaining core quality standards, ultimately enhancing project efficiency, and reducing the risk of failure.
Case studies illustrate how to solve real, materials-related problems. Description of some basic design principles, followed by specific case studies. Topics include: five basic steps in an iterative design process. iterative and concurrent design processes. materials selection and the mechanical design process
The present study aims to study the treatment of (RCA) faction (16/25) by their encapsulation into cement slurry with a (W/C) ratio of (0.5). A complete factorial plan 2 3 was applied by considering three independent factors, namely the state of RCA (GR), the soaking time into slurry (DT) and the hardening time (RCA) (A). The selected responses ...
However, to appreciate the versatility of concrete as a structural material, attention should turn to Universities in North America, where concrete canoes are built and raced - as described by the last case study in this chapter. 9.1. Case study: The concrete canoe
MS in Materials Science and Engineering. Advance your career—and technological innovations—with a more in-depth knowledge of the properties and capabilities of metals, ceramics, semiconductors and composite materials. Now is an exciting time to be a materials engineer—almost every technology we rely on in the modern world relies on ...
ChapterPDF Available. Mechanical Properties of Engineering Materials: Relevance in Design and Manufacturing. April 2018. DOI: 10.1007/978-3-319-78488-5_1. In book: Introduction to Mechanical ...
This study takes a material engineering approach to choosing a material and compares it to the actual steps that were taken to reach a solution." Skateboard Wheels: Reserve desk - Lewis, G., Selection of Engineering Materials, Skateboard Wheels, Chapter 16, Prentice Hall, Englewood Cliffs, New Jersey, 1990. 143 - 148. This case study focuses on ...
Engineering Case Studies Online is a collection that will grow to include 250 hours of video and 50,000 pages of text resources to meet this growing need. Content is displayed alongside targeted learning objects designed to facilitate detailed understanding of the causes and impact of these failures. Materials in the collection include:
Each case study is laid out in the same way: the problem statement, setting the scene, the model, identifying function, constraints, objectives, and free variables, from which emerge the attribute limits and material indices, the selection in which the full menu of materials is reduced by screening and ranking to a short-list of viable candidates,
ABSTRACT. Most books on forensic engineering focus on civil engineering failures rather than consumer or general mechanical products. Unique both in scope and style, this treatment is built upon case studies of real accidents, broadly focused on consumer products, and dedicated to problem solving through scientific principles.
Congratulations to Anuvi Gupta, a sophomore in the Department of Materials Science and Engineering, on being selected as a Case School of Engineering at Case Western Reserve University SOURCE - STEM 2023 Summer Research Scholar. Tuesday, August 1, 2023.
Additive manufacturing, better known as 3D printing, is an innovative manufacturing technique which allows the production of parts, with complex and challenging shapes, layer by layer mainly through melting powder particles (metallic, polymeric, or composite) or extruding material in the form of wire, depending on the specific technique. Three-dimensional printing is already widely employed in ...
Explore the materials branch. The range of elements available for device production has exploded in the past 20 years, enabling entirely new classes of electronic and mechanical products, biomedical implants, tissues, coatings and more. We're leaders in the world of advanced materials, with three of our school's seven departments moving ...
White Building (7204) Phone: 216.368.4230 Department Chair: Frank Ernst [email protected]. Materials science and engineering is a discipline that extends from understanding the microscopic structure and properties of materials to designing materials in engineering systems and evaluating their performance.
Research Professor in Materials Science and Mechanical Engineering at UDLAP with more than 20 years of teaching experience in Higher Education. ... M.-R., Rosas-Fernández, J.-B., & Molina, A. (2021). The core components of education 4.0 in higher education: Three case studies in engineering education. Computers & Electrical Engineering, 93 ...
Doping is a widely explored technique to modify the properties of semiconducting materials. In the case of tungsten trioxide (WO 3), doping with elements such as SnO 2 and SrO has garnered significant attention owing to its potential impact on structural, functional, and optical characteristics. We report on structural, functional, optical, and dielectric properties of SnO 2 /WO 3 and SrO/SnO ...
The tensile strength of rock materials, a crucial factor for the stability of underground engineering, is much lower than its compressive strength. Moreover, the tensile strength of rock under confining pressure differs significantly from that of unconfined conditions, presenting a compelling avenue for investigation. In this work, the tensile strength of rock under confining pressure was ...
Real-world engineering structures will normally have welded components, which require a special consideration in the fatigue analysis process. In this chapter, global and local fatigue modeling approaches for welded structures are introduced. ... Includes many compelling case studies of materials failure in chemical processing plants, concrete ...
A Simple Case Study of Material Requirement Planning. IOSR E-Jurnal Manajemen, Vol. 9, No. 2, 2020 : 426-445 445 Journal of Mechanical and Civil Engineering, 9(5), 58-64. Husen, 2011. Project Management. ANDI. ... International Journal of Marine Engineering Innovation and Research, Vol. 8(3), Sept. 2023. 449-459 (pISSN: 2541-5972, eISSN: 2548 ...
Tuning ferromagnetic superexchange interactions through cation disorder engineering in two-dimensional semiconductor alloys: A case study of CrMoSe 2 Br 2 Phys. Rev. B Hongxu Luo and Sai Lyu Accepted 12 September 2024. ... we computationally study the ferromagnetic superexchange interactions in the ordered, partially ordered, ...
Background. The following case study illustrates how the Predicted Crash Frequency with CMF Adjustment method has been used to explicitly consider the safety impacts of opportunities during the Value Engineering (VE) process. Specifically, it focuses on the quantification of safety in the evaluation phase when safety is a project factor and crash frequency is the related performance measure.