NOTIFICATIONS
Measuring the speed of sound.
- + Create new collection
In this investigation, students measure distance and time in order to calculate the speed of a sound wave.
The investigation supports the science capability ‘Gather and interpret data’. It also provides a real-world context in which to practise mathematical skills.
By the end of this investigation, students should be able to:
- calculate the speed of sound
- explain why we see a lightning bolt before we hear the thunder.
Equipment required includes:
- sound-making device (wooden clapper)
- device with timing software app
- tape measure
- an outdoor space of at least 150 metres with a building at one end.
Download the Word file (see link below).

Related content
This article is part of an article series :
- Sound – understanding standing waves
- Sound – visualising sound waves
- Sound – resonance
- Sound – wave interference
- Sound – beats, the Doppler effect and sonic booms
with the accompanying investigation:
- Investigating sound wave resonance
Learn more about sound with these articles:
- Hearing sound
- Sound on the move
- Measuring sound
Useful links
Download the app software from https://phyphox.org/ :
- Android app
See our newsletters here .
Would you like to take a short survey?
This survey will open in a new tab and you can fill it out after your visit to the site.

Advertisement
How to Measure Sound Travel in the Air
- Share Content on Facebook
- Share Content on LinkedIn
- Share Content on Flipboard
- Share Content on Reddit
- Share Content via Email
Key Takeaways
- Sound can travel through air, water and solids but not through a vacuum, as it requires a medium to propagate.
- The speed of sound in air is approximately 1,130 feet (344 meters) per second at room temperature, though this speed can vary with changes in temperature and humidity.
- You can perform a simple experiment involving two blocks of wood, a stopwatch and a tape measure to measure the speed of sound in air by calculating the time it takes for the sound to travel a known distance.
Sound can travel through most materials -- the most commonly known being air (gas), water (liquid) and steel (solid). However, it does not travel at all in a vacuum, because the sound waves need some kind of medium in which to travel. In addition, some materials absorb, rather than reflect or pass, sound waves. This is the basis of soundproofing [source: Kurtus ].
The average speed of sound through air is about 1130 feet per second (344 meters per second) at room temperature. However, changes in temperature and humidity will affect this speed [source: Kurtus ].
Here is a simple way to measure the speed at which sound travels through air. You'll need the following items:
- Two blocks of wood, or other items that make a loud, sharp sound when struck together
- A stopwatch
- A friend to help with the experiment
- A tape measure
Instructions:
- Find a large empty area, such as a field or large court.
- Choose two spots on opposite ends of the area where each person will stand.
- Measure the distance between the two spots using a tape measure. Alternatively, you can count off measured steps between the two spots.
- Have your friend take the blocks and stand at one spot, holding them up high.
- Take the stopwatch and stand at the other spot. Make sure you have a clear view of the blocks.
- Signal your friend to bang the two blocks together hard.
- Start the stopwatch as soon as you see the blocks hit each other.
- Press stop as soon as you hear the sound from the blocks.
- Calculate the speed of the sound by dividing the distance between you and your friend by the elapsed time. To get a more accurate measurement, repeat the above steps a few times and then take an average of the results [source: Green Planet Solar Energy ]. //]]]]> ]]>
Frequently Asked Questions
How does the humidity level affect the speed of sound in air, can the speed of sound vary at different altitudes.
Please copy/paste the following text to properly cite this HowStuffWorks.com article:
Choose an Account to Log In

Notifications
Science project, sound resonance: how to calculate speed of sound.
Sounds, whether from talking, music, an earthquake, a fire or a sonic boom, travel in waves. Wave travel, called propagation , has a different velocity through each material, like water or air.
Frequency is the number of times an event occurs per unit of time. For sound, the frequency is measured in Hertz , abbreviated Hz , which means is period cycle, from the top of one wave to another, per second. Humans can normally hear anywhere from 20Hz to 20000Hz, while dogs can hear up to 60,000 Hz, and some bats can hear sound as low as 1 Hz. Sound is perceived in pitch ; higher frequencies sound like higher pitches (like a whistle) and lower frequencies have lower pitches (like a tuba).
A tuning fork is an object that resonates , or vibrates, at a specific frequency and pitch. The sound of a tuning fork depends on the length of the prongs and can be used to tune musical instruments.
Calculate the speed of sound in air.
- Tuning fork with known frequency
- Large, tall cylindrical glass container
- Rubber mallet
Procedure
- Fill the glass container halfway up with water.
- Strike the tuning fork with the rubber mallet. Why should you use a mallet to strike the tuning for rather than a hard surface like a table?
- Hold the tuning fork over the opening of the glass container as pictured. Record your observations. How does holding the fork over the container change the sound you hear? Why does this happen?
- While keeping the tuning fork over the opening of the container, slowly pour more water into the cylinder. If you cannot do it yourself, ask a friend or family member to help you. Listen for the point when there is a change in pitch (frequency).
- Remove some water and experiment with adding it again until you can identify the height of the water at which the frequency jumps. Why does the frequency jump?
- Calculate the velocity of sound at this height using the following equation:
v = 4f (H + 0.4D)
is the velocity of sound |
is the frequency of the tuning fork in Hz |
is the height distance between the top of the water and the lip of the cylinder |
is the diameter of the water container in meters |
The speed of sound in air is 343 m/s (nearly a mile in 5 seconds!) at 20°C. Compare your experimental value to the true value. If it is warmer, the speed will be faster.
The change in sound in the water column will occur when the graduated cylinder produces the same resonance as the tuning fork. Simply put, this means that one period of the wave goes to the bottom of the cylinder and back in the same amount of time it takes for the tuning fork to vibrate one time.
The fundamental frequency is the frequency of one period of a wavelength. The second harmonic, also called the 1 st overtone, is an integer (whole number) multiple of the fundamental frequency. Many people will not hear the difference between harmonics, but they are commonly used in music, and examples of harmonics can even be found in the human voice.
Here are a few examples of frequencies applied to musical notes:
|
|
|
| Harmonic height (m) |
C | 256 | 1.34 | 0.33 | 0.17 |
C# | 278 | 1.23 | 0.31 | 0.16 |
D | 294 | 1.17 | 0.29 | 0.15 |
E | 311 | 1.10 | 028 | 0.14 |
E | 330 | 1.04 | 0.26 | 0.13 |
F | 349 | 0.98 | 0.24 | 0.12 |
F# | 370 | 0.93 | 0.23 | 0.12 |
G | 392 | 0.88 | 0.22 | 0.11 |
A | 415 | 0.83 | 0.20 | 0.11 |
A | 440 | 0.78 | 0.19 | 0.10 |
B | 494 | 0.69 | 0.18 | 0.09 |
Wavelength is the length in meters of one period of the wave, from peak to peak. It is often denoted by the Greek symbol lambda λ and can be calculated by the equation
v = λ f
is the velocity in m/s |
is wavelength in meters |
is frequency in Hz, or s |
Related learning resources
Add to collection, create new collection, new collection, new collection>, sign up to start collecting.
Bookmark this to easily find it later. Then send your curated collection to your children, or put together your own custom lesson plan.

- Feb 2, 2023
Seven experiments to measure the speed of sound in the air
Updated: Feb 15
Calculating the speed of sound becomes a simple and engaging task with the use of a smartphone, transforming this commonly abstract concept into a tangible learning experience. For students, this exercise is particularly gratifying as it demystifies the complexities of sound waves, allowing them to explore its various physical properties through a device that's a familiar staple in their everyday lives. In this article, we introduce seven experiments, each utilizing a smartphone and the FizziQ app , to determine the speed of sound, making science interactive and accessible.
Sound waves and their propagation - Methods for measuring the speed of sound - Measurement by time of flight - Measurement by wavelength - Measurement by resonance frequency - Conclusion
Sound waves and their propagation
A sound wave is a mechanical vibration that propagates through a medium, such as air or a liquid. The speed of sound is the speed at which this wave propagates in this m edium, it depends on the temperature, the pressure and the density of the medium through which it propagates. In air, if we assimilate it to a perfect diatomic gas, we can calculate the speed of sound by the equation: c = sqrt(γ*RT/Ma), c, the speed of sound, γ, the ratio of heat capacities at constant pressure and volume. γ= 7/5 for air, R, the ideal gas constant, T, the absolute temperature of the medium, Ma, the molar mass of air: Ma = 29g/mol. Using the previous formula we can calculate the theoretical speed of sound at the usual conditions of temperature and pressure: c = 343 m/s for a temperature of 20 degrees, or approximately 767 miles per hour. In water, sound travels more than 4 times faster than in air, i.e. at about 1,482 meters per second, and in some metals like soft iron, it travels significantly faster at close to 6,000 m/s (13,333 miles per hour).
How to measure the speed of sound with a smartphone?
There are many different ways to measure the speed of sound using a smartphone or a tablet. These methods fall into three broad categories, which, interestingly, use different physical characteristics of sound waves :
Estimating the time of flight (ToF )
Measuring the sound frequency using an Helmholtz resonator
Measuring the wavelength at a given frequency
These are the methods that have been used by generations of scientists to determine the speed of sound:
➡️ Marin Mersenne, the first, evaluated in 1635 the speed of sound in air at 448 m/s by the propagation time method. Value further refined by the scientists Viviani and Borelli in 1656 with a value of 344 m/s.
➡️ Isaac Newton took a different approach through an analytical method by determining it from the resonant frequencies of sound waves in a U-tube and details his method in the first edition ofIt begins (1687).
➡️ Over the centuries, as estimations were more accurate, one uncertainty remained: could humans go faster than the speed of sound? This question will be resolved in 1947 when American aviator Chuck Yeager reached Mach 1 aboard the X-1 aircraft . Once again, the human had crossed an impassable barrier.
Now, the iconic measurement of the speed of sound is readily accessible to anyone. You can delve into these experiments using one or more smartphones, with no need for specialized equipment. Embark on a journey of discovery right at your fingertips, grab your cellphones and let's dive into this exciting venture!
Measuring the Time of Flight (ToF)
Like any speed calculation, the objective here is to determine the time it takes for a sound wave to travel a certain distance . The speed of sound being high, the measurement of time requires a specific equipment: an acoustic stopwatch.
An acoustic stopwatch measures the time difference between two sounds which sound level exceeds a certain threshold. This device cannot be found on a lab bench but many smartphone applications exist that offer this functionality. In FizziQ, you will find the acoustic stopwatch in the Tools menu. You can also build your own acoustic stopwatch using triggers .
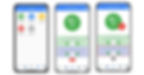
The traditional protocol for measuring the speed of sound with an acoustic stopwatch is as follows: two smartphones are separated by a certain distance (at least 5 meters), and an operator is placed near each telephone. The operators clap their hands one after the other. The first clap starts both stopwatch and the seconds stops them. Students then check that the time difference dt between the two stopwatches is dt = 2*d/c, where d is the distance between the smartphones, c the speed of sound. This experiment allows an accuracy between 5 and 10%, and can be improved by performing several measurements. An opportunity to do a bit of statistics as well !
The protocol works well, but younger students find it often difficult to understand the offset formula calculation which is not very intuitive. We prefer a variation of this protocol developed by Aline Chaillou of the La main à la pâte Foundation.
In this second protocol, we start by synchronizing the chronometers by putting them side by side and trigger the sound chronometers by clapping our hands. Then, we move one of the two smartphone by a certain distance d without making noise. An operator located near this second laptop then stops the two stopwatches by clapping his hands. The calculation of the shift is then very intuitive for the students because they have immediately put in relation the difference in distance which creates the phase shift with the displacement of one of the two smartphones.
The time difference dt is equal to: dt = d/c.
This second protocol also makes it possible to introduce the notion of clock synchronization. It is the same concept of synchronization that was used in the famous Hafele-Keating experiment in 1971 to prove relativity. Be mindful to calibrate the trigger level of the sound stopwatch so that it does not trigger when you move one of the two smartphones.
Watch our vidéo :
Measuring the speed of sound with Helmholtz resonators
The second method of calculating the speed of sound is based on the principle of acoustic resonance, which is a phenomenon in which an acoustic system amplifies sound waves whose frequency corresponds to one of its own frequencies of vibration. The resonance frequencies of certain cavities like a cylinders or a bottles are easy to determine by calculus. This frequency depends on the speed of sound and the shape of the object. By measuring the resonance frequency we can infer the speed of sound.
A very simple first protocol consists of blowing on the edge of a graduated test tube. This emits a sound for which we can measure the fundamental frequency using FizziQ. For a closed tube, the fundamental resonance frequency is: f₀ = c(4*L+1.6*D), where L is the length of the tube, D is the diameter of the tube.
To make more precise measurements, we can measure the frequency for different heights of water in the test piece, and by doing a linear regression of the results, we can accurately determine the speed of sound to less than one percent.
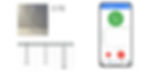
If you are a Bordeaux lover and have an empty bottle, you can use a bottle from this region whose volumetric characteristics are immutable. Ulysse Delabre in this video details the calculations for measuring the resonance frequency when blowing into the bottle.
What if the bottle is unopened? It is still possible to carry out the experiment and, paradoxically, in an even simpler way: by uncorking it! When the cork is removed, a "pop" is heard which is due to the resonance of the air in the part between the liquid and the top of the bottle. If we measure the frequency of pop with the frequency meter, we can use the previous formula of the resonant frequency of a tube to deduce the speed of sound.
A last protocol which always surprises students uses the fact that if several frequencies are emitted simultaneously in a cavity, the harmonics of the resonant frequency of the cavity will be amplified compared to the other emitted frequencies. If we measure the spectrum of a white noise emitted in this cavity, the harmonic frequencies of the resonant frequency are highlighted compared to the others. It is recalled that white noise is a random succession of sound emitted in all frequencies. White noise sounds can be found in FizziQ's sound library.
So let's take a tube open at both ends, such as a paper towel roll or a vacuum cleaner hose. At one end of the tube, we will emit a white noise that can be generated with the FizziQ sound library or by using the sound of a video emitting white or pink noise. At the other end of the tube, we measure the frequency spectrum. Measuring the white noise spectrum through a tube will show peaks for the fundamental frequency and its harmonics. We deduce the resonance frequency then the speed of sound by the formula of the resonance frequency of an open tube : f₀ = c(2*L+1.6*D)
Better results are often obtained with pink noise, which is similar to white noise, but with a reduced loudness for high-pitched sounds. The use of pink noise makes it possible to reinforce the intensity of the fundamental resonant frequency compared to its higher harmonics. Examples of of pink noise can be found on internet.
Finally, one can make different measurements with different sizes of the tube, and deduce c by measuring the slope on the graph.
Measuring the speed of sound with waves interferences
This third type of protocol is based on measuring the wavelength of a pure sound of known frequency. We deduce the speed by the relation: c = l.f, with l the wavelength and f the frequency.
This method is the one usually used in the school labs. It uses a sound source and two microphones placed at a certain distance from this source and connected to an oscilloscope with a dual input. By moving the two microphones relative to each other, the operator finds the distance for which the two waves are in phase, which is the wavelength.
With smartphones, this protocol is not possible because they do not have dual sound inputs... However with a little imagination we can find other ways!
The first protocol that we propose consists in using two smartphones that emit the same pure sound, for example at a frequency of 680 hertz. By placing the smartphones at a certain distance, we will calculate the places along the two smartphones axis where waves add up and places where they cance.
With FizziQ one can use the sound at 680 hertz from the sound library. Two smartphones are placed about 3 meters from each other. A third smartphone is used to measure the sound intensity (oscillogram instrument on FizziQ) along the axis of the two smartphones. The interference of the two waves creates zones of very high intensities, the antinodes, and other very weak ones, the nodes. The distance between the nodes (about 50 cm) is equal to the wavelength of the sound wave for the frequency 680 hertz. By measuring the difference between the nodes (or the bellies), we calculate the speed of sound.
This experience also opens an interesting discussion on how active noise reduction headphones work by carrying out a small activity: https://www.fizziq.org/en/team/noise-cancellation
The experiment can also be carried out with only two mobile phones. One of the two smartphones then serves as a transmitter, and also as a tool for measuring the sound volume. A second mobile that emits a pure sound of the same frequency is approached to the first, and the distance between the knot and the belly is noted by measuring the sound volume on the first smartphone, identified by the variations in intensity. To carry out this experiment with FizziQ, we prefer to use the sound intensity measured with the Oscilloscope instrument and which is more precise than the sound volume in decibels.
Finally, if you only have a smartphone, it is also possible to carry out this experiment by placing a reflective surface in place of the second smartphone from the previous experiment. The precision is further reduced but the calculation is nevertheless possible!
These different experiments make it possible to calculate the speed of sound with an accuracy of about 10%.
To conclude
We have identified a number of different ways to estimate the speed of sound. These experiments can be classified into three categories that relate to different physical properties of sound waves. All of these experiments can be done with FizziQ, or with other mobile or tablet apps, depending on your preference. The smartphone is one of the best tools available for measuring the speed of sound, offering multiple ways to approach the same problem, and easily accessible to students. Happy experimenting!
Recent Posts
Seven Experiments on Gravity with your Smartphone
Measuring Pi with Smartphone Sensors: A Fun and Educational Challenge
5 science projects in biomechanics with a smartphone
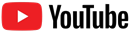
- TPC and eLearning
- What's NEW at TPC?
- Read Watch Interact
- Practice Review Test
- Teacher-Tools
- Request a Demo
- Get A Quote
- Subscription Selection
- Seat Calculator
- Ad Free Account
- Edit Profile Settings
- Metric Conversions Questions
- Metric System Questions
- Metric Estimation Questions
- Significant Digits Questions
- Proportional Reasoning
- Acceleration
- Distance-Displacement
- Dots and Graphs
- Graph That Motion
- Match That Graph
- Name That Motion
- Motion Diagrams
- Pos'n Time Graphs Numerical
- Pos'n Time Graphs Conceptual
- Up And Down - Questions
- Balanced vs. Unbalanced Forces
- Change of State
- Force and Motion
- Mass and Weight
- Match That Free-Body Diagram
- Net Force (and Acceleration) Ranking Tasks
- Newton's Second Law
- Normal Force Card Sort
- Recognizing Forces
- Air Resistance and Skydiving
- Solve It! with Newton's Second Law
- Which One Doesn't Belong?
- Component Addition Questions
- Head-to-Tail Vector Addition
- Projectile Mathematics
- Trajectory - Angle Launched Projectiles
- Trajectory - Horizontally Launched Projectiles
- Vector Addition
- Vector Direction
- Which One Doesn't Belong? Projectile Motion
- Forces in 2-Dimensions
- Being Impulsive About Momentum
- Explosions - Law Breakers
- Hit and Stick Collisions - Law Breakers
- Case Studies: Impulse and Force
- Impulse-Momentum Change Table
- Keeping Track of Momentum - Hit and Stick
- Keeping Track of Momentum - Hit and Bounce
- What's Up (and Down) with KE and PE?
- Energy Conservation Questions
- Energy Dissipation Questions
- Energy Ranking Tasks
- LOL Charts (a.k.a., Energy Bar Charts)
- Match That Bar Chart
- Words and Charts Questions
- Name That Energy
- Stepping Up with PE and KE Questions
- Case Studies - Circular Motion
- Circular Logic
- Forces and Free-Body Diagrams in Circular Motion
- Gravitational Field Strength
- Universal Gravitation
- Angular Position and Displacement
- Linear and Angular Velocity
- Angular Acceleration
- Rotational Inertia
- Balanced vs. Unbalanced Torques
- Getting a Handle on Torque
- Torque-ing About Rotation
- Properties of Matter
- Fluid Pressure
- Buoyant Force
- Sinking, Floating, and Hanging
- Pascal's Principle
- Flow Velocity
- Bernoulli's Principle
- Balloon Interactions
- Charge and Charging
- Charge Interactions
- Charging by Induction
- Conductors and Insulators
- Coulombs Law
- Electric Field
- Electric Field Intensity
- Polarization
- Case Studies: Electric Power
- Know Your Potential
- Light Bulb Anatomy
- I = ∆V/R Equations as a Guide to Thinking
- Parallel Circuits - ∆V = I•R Calculations
- Resistance Ranking Tasks
- Series Circuits - ∆V = I•R Calculations
- Series vs. Parallel Circuits
- Equivalent Resistance
- Period and Frequency of a Pendulum
- Pendulum Motion: Velocity and Force
- Energy of a Pendulum
- Period and Frequency of a Mass on a Spring
- Horizontal Springs: Velocity and Force
- Vertical Springs: Velocity and Force
- Energy of a Mass on a Spring
- Decibel Scale
- Frequency and Period
- Closed-End Air Columns
- Name That Harmonic: Strings
- Rocking the Boat
- Wave Basics
- Matching Pairs: Wave Characteristics
- Wave Interference
- Waves - Case Studies
- Color Addition and Subtraction
- Color Filters
- If This, Then That: Color Subtraction
- Light Intensity
- Color Pigments
- Converging Lenses
- Curved Mirror Images
- Law of Reflection
- Refraction and Lenses
- Total Internal Reflection
- Who Can See Who?
- Formulas and Atom Counting
- Lab Equipment
- Atomic Models
- Bond Polarity
- Entropy Questions
- Cell Voltage Questions
- Heat of Formation Questions
- Reduction Potential Questions
- Oxidation States Questions
- Measuring the Quantity of Heat
- Hess's Law
- Oxidation-Reduction Questions
- Galvanic Cells Questions
- Thermal Stoichiometry
- Molecular Polarity
- Quantum Mechanics
- Balancing Chemical Equations
- Bronsted-Lowry Model of Acids and Bases
- Classification of Matter
- Collision Model of Reaction Rates
- Density Ranking Tasks
- Dissociation Reactions
- Complete Electron Configurations
- Elemental Measures
- Enthalpy Change Questions
- Equilibrium Concept
- Equilibrium Constant Expression
- Equilibrium Calculations - Questions
- Equilibrium ICE Table
- Intermolecular Forces Questions
- Ionic Bonding
- Lewis Electron Dot Structures
- Limiting Reactants
- Line Spectra Questions
- Mass Stoichiometry
- Measurement and Numbers
- Metals, Nonmetals, and Metalloids
- Metric Estimations
- Metric System
- Molarity Ranking Tasks
- Mole Conversions
- Name That Element
- Names to Formulas
- Names to Formulas 2
- Nuclear Decay
- Particles, Words, and Formulas
- Periodic Trends
- Precipitation Reactions and Net Ionic Equations
- Pressure Concepts
- Pressure-Temperature Gas Law
- Pressure-Volume Gas Law
- Chemical Reaction Types
- Significant Digits and Measurement
- States Of Matter Exercise
- Stoichiometry Law Breakers
- Stoichiometry - Math Relationships
- Subatomic Particles
- Spontaneity and Driving Forces
- Gibbs Free Energy
- Volume-Temperature Gas Law
- Acid-Base Properties
- Energy and Chemical Reactions
- Chemical and Physical Properties
- Valence Shell Electron Pair Repulsion Theory
- Writing Balanced Chemical Equations
- Mission CG1
- Mission CG10
- Mission CG2
- Mission CG3
- Mission CG4
- Mission CG5
- Mission CG6
- Mission CG7
- Mission CG8
- Mission CG9
- Mission EC1
- Mission EC10
- Mission EC11
- Mission EC12
- Mission EC2
- Mission EC3
- Mission EC4
- Mission EC5
- Mission EC6
- Mission EC7
- Mission EC8
- Mission EC9
- Mission RL1
- Mission RL2
- Mission RL3
- Mission RL4
- Mission RL5
- Mission RL6
- Mission KG7
- Mission RL8
- Mission KG9
- Mission RL10
- Mission RL11
- Mission RM1
- Mission RM2
- Mission RM3
- Mission RM4
- Mission RM5
- Mission RM6
- Mission RM8
- Mission RM10
- Mission LC1
- Mission RM11
- Mission LC2
- Mission LC3
- Mission LC4
- Mission LC5
- Mission LC6
- Mission LC8
- Mission SM1
- Mission SM2
- Mission SM3
- Mission SM4
- Mission SM5
- Mission SM6
- Mission SM8
- Mission SM10
- Mission KG10
- Mission SM11
- Mission KG2
- Mission KG3
- Mission KG4
- Mission KG5
- Mission KG6
- Mission KG8
- Mission KG11
- Mission F2D1
- Mission F2D2
- Mission F2D3
- Mission F2D4
- Mission F2D5
- Mission F2D6
- Mission KC1
- Mission KC2
- Mission KC3
- Mission KC4
- Mission KC5
- Mission KC6
- Mission KC7
- Mission KC8
- Mission AAA
- Mission SM9
- Mission LC7
- Mission LC9
- Mission NL1
- Mission NL2
- Mission NL3
- Mission NL4
- Mission NL5
- Mission NL6
- Mission NL7
- Mission NL8
- Mission NL9
- Mission NL10
- Mission NL11
- Mission NL12
- Mission MC1
- Mission MC10
- Mission MC2
- Mission MC3
- Mission MC4
- Mission MC5
- Mission MC6
- Mission MC7
- Mission MC8
- Mission MC9
- Mission RM7
- Mission RM9
- Mission RL7
- Mission RL9
- Mission SM7
- Mission SE1
- Mission SE10
- Mission SE11
- Mission SE12
- Mission SE2
- Mission SE3
- Mission SE4
- Mission SE5
- Mission SE6
- Mission SE7
- Mission SE8
- Mission SE9
- Mission VP1
- Mission VP10
- Mission VP2
- Mission VP3
- Mission VP4
- Mission VP5
- Mission VP6
- Mission VP7
- Mission VP8
- Mission VP9
- Mission WM1
- Mission WM2
- Mission WM3
- Mission WM4
- Mission WM5
- Mission WM6
- Mission WM7
- Mission WM8
- Mission WE1
- Mission WE10
- Mission WE2
- Mission WE3
- Mission WE4
- Mission WE5
- Mission WE6
- Mission WE7
- Mission WE8
- Mission WE9
- Vector Walk Interactive
- Name That Motion Interactive
- Kinematic Graphing 1 Concept Checker
- Kinematic Graphing 2 Concept Checker
- Graph That Motion Interactive
- Two Stage Rocket Interactive
- Rocket Sled Concept Checker
- Force Concept Checker
- Free-Body Diagrams Concept Checker
- Free-Body Diagrams The Sequel Concept Checker
- Skydiving Concept Checker
- Elevator Ride Concept Checker
- Vector Addition Concept Checker
- Vector Walk in Two Dimensions Interactive
- Name That Vector Interactive
- River Boat Simulator Concept Checker
- Projectile Simulator 2 Concept Checker
- Projectile Simulator 3 Concept Checker
- Hit the Target Interactive
- Turd the Target 1 Interactive
- Turd the Target 2 Interactive
- Balance It Interactive
- Go For The Gold Interactive
- Egg Drop Concept Checker
- Fish Catch Concept Checker
- Exploding Carts Concept Checker
- Collision Carts - Inelastic Collisions Concept Checker
- Its All Uphill Concept Checker
- Stopping Distance Concept Checker
- Chart That Motion Interactive
- Roller Coaster Model Concept Checker
- Uniform Circular Motion Concept Checker
- Horizontal Circle Simulation Concept Checker
- Vertical Circle Simulation Concept Checker
- Race Track Concept Checker
- Gravitational Fields Concept Checker
- Orbital Motion Concept Checker
- Angular Acceleration Concept Checker
- Balance Beam Concept Checker
- Torque Balancer Concept Checker
- Aluminum Can Polarization Concept Checker
- Charging Concept Checker
- Name That Charge Simulation
- Coulomb's Law Concept Checker
- Electric Field Lines Concept Checker
- Put the Charge in the Goal Concept Checker
- Circuit Builder Concept Checker (Series Circuits)
- Circuit Builder Concept Checker (Parallel Circuits)
- Circuit Builder Concept Checker (∆V-I-R)
- Circuit Builder Concept Checker (Voltage Drop)
- Equivalent Resistance Interactive
- Pendulum Motion Simulation Concept Checker
- Mass on a Spring Simulation Concept Checker
- Particle Wave Simulation Concept Checker
- Boundary Behavior Simulation Concept Checker
- Slinky Wave Simulator Concept Checker
- Simple Wave Simulator Concept Checker
- Wave Addition Simulation Concept Checker
- Standing Wave Maker Simulation Concept Checker
- Color Addition Concept Checker
- Painting With CMY Concept Checker
- Stage Lighting Concept Checker
- Filtering Away Concept Checker
- InterferencePatterns Concept Checker
- Young's Experiment Interactive
- Plane Mirror Images Interactive
- Who Can See Who Concept Checker
- Optics Bench (Mirrors) Concept Checker
- Name That Image (Mirrors) Interactive
- Refraction Concept Checker
- Total Internal Reflection Concept Checker
- Optics Bench (Lenses) Concept Checker
- Kinematics Preview
- Velocity Time Graphs Preview
- Moving Cart on an Inclined Plane Preview
- Stopping Distance Preview
- Cart, Bricks, and Bands Preview
- Fan Cart Study Preview
- Friction Preview
- Coffee Filter Lab Preview
- Friction, Speed, and Stopping Distance Preview
- Up and Down Preview
- Projectile Range Preview
- Ballistics Preview
- Juggling Preview
- Marshmallow Launcher Preview
- Air Bag Safety Preview
- Colliding Carts Preview
- Collisions Preview
- Engineering Safer Helmets Preview
- Push the Plow Preview
- Its All Uphill Preview
- Energy on an Incline Preview
- Modeling Roller Coasters Preview
- Hot Wheels Stopping Distance Preview
- Ball Bat Collision Preview
- Energy in Fields Preview
- Weightlessness Training Preview
- Roller Coaster Loops Preview
- Universal Gravitation Preview
- Keplers Laws Preview
- Kepler's Third Law Preview
- Charge Interactions Preview
- Sticky Tape Experiments Preview
- Wire Gauge Preview
- Voltage, Current, and Resistance Preview
- Light Bulb Resistance Preview
- Series and Parallel Circuits Preview
- Thermal Equilibrium Preview
- Linear Expansion Preview
- Heating Curves Preview
- Electricity and Magnetism - Part 1 Preview
- Electricity and Magnetism - Part 2 Preview
- Vibrating Mass on a Spring Preview
- Period of a Pendulum Preview
- Wave Speed Preview
- Slinky-Experiments Preview
- Standing Waves in a Rope Preview
- Sound as a Pressure Wave Preview
- DeciBel Scale Preview
- DeciBels, Phons, and Sones Preview
- Sound of Music Preview
- Shedding Light on Light Bulbs Preview
- Models of Light Preview
- Electromagnetic Radiation Preview
- Electromagnetic Spectrum Preview
- EM Wave Communication Preview
- Digitized Data Preview
- Light Intensity Preview
- Concave Mirrors Preview
- Object Image Relations Preview
- Snells Law Preview
- Reflection vs. Transmission Preview
- Magnification Lab Preview
- Reactivity Preview
- Ions and the Periodic Table Preview
- Periodic Trends Preview
- Chemical Reactions Preview
- Intermolecular Forces Preview
- Melting Points and Boiling Points Preview
- Bond Energy and Reactions Preview
- Reaction Rates Preview
- Ammonia Factory Preview
- Stoichiometry Preview
- Nuclear Chemistry Preview
- Gaining Teacher Access
- Task Tracker Directions
- Conceptual Physics Course
- On-Level Physics Course
- Honors Physics Course
- Chemistry Concept Builders
- All Chemistry Resources
- Users Voice
- Tasks and Classes
- Webinars and Trainings
- Subscription
- Subscription Locator
- 1-D Kinematics
- Newton's Laws
- Vectors - Motion and Forces in Two Dimensions
- Momentum and Its Conservation
- Work and Energy
- Circular Motion and Satellite Motion
- Thermal Physics
- Static Electricity
- Electric Circuits
- Vibrations and Waves
- Sound Waves and Music
- Light and Color
- Reflection and Mirrors
- About the Physics Interactives
- Task Tracker
- Usage Policy
- Newtons Laws
- Vectors and Projectiles
- Forces in 2D
- Momentum and Collisions
- Circular and Satellite Motion
- Balance and Rotation
- Electromagnetism
- Waves and Sound
- Atomic Physics
- Forces in Two Dimensions
- Work, Energy, and Power
- Circular Motion and Gravitation
- Sound Waves
- 1-Dimensional Kinematics
- Circular, Satellite, and Rotational Motion
- Einstein's Theory of Special Relativity
- Waves, Sound and Light
- QuickTime Movies
- About the Concept Builders
- Pricing For Schools
- Directions for Version 2
- Measurement and Units
- Relationships and Graphs
- Rotation and Balance
- Vibrational Motion
- Reflection and Refraction
- Teacher Accounts
- Kinematic Concepts
- Kinematic Graphing
- Wave Motion
- Sound and Music
- About CalcPad
- 1D Kinematics
- Vectors and Forces in 2D
- Simple Harmonic Motion
- Rotational Kinematics
- Rotation and Torque
- Rotational Dynamics
- Electric Fields, Potential, and Capacitance
- Transient RC Circuits
- Light Waves
- Units and Measurement
- Stoichiometry
- Molarity and Solutions
- Thermal Chemistry
- Acids and Bases
- Kinetics and Equilibrium
- Solution Equilibria
- Oxidation-Reduction
- Nuclear Chemistry
- Newton's Laws of Motion
- Work and Energy Packet
- Static Electricity Review
- NGSS Alignments
- 1D-Kinematics
- Projectiles
- Circular Motion
- Magnetism and Electromagnetism
- Graphing Practice
- About the ACT
- ACT Preparation
- For Teachers
- Other Resources
- Solutions Guide
- Solutions Guide Digital Download
- Motion in One Dimension
- Work, Energy and Power
- Chemistry of Matter
- Measurement and the Metric System
- Algebra Based On-Level Physics
- Honors Physics
- Conceptual Physics
- Other Tools
- Frequently Asked Questions
- Purchasing the Download
- Purchasing the Digital Download
- About the NGSS Corner
- NGSS Search
- Force and Motion DCIs - High School
- Energy DCIs - High School
- Wave Applications DCIs - High School
- Force and Motion PEs - High School
- Energy PEs - High School
- Wave Applications PEs - High School
- Crosscutting Concepts
- The Practices
- Physics Topics
- NGSS Corner: Activity List
- NGSS Corner: Infographics
- About the Toolkits
- Position-Velocity-Acceleration
- Position-Time Graphs
- Velocity-Time Graphs
- Newton's First Law
- Newton's Second Law
- Newton's Third Law
- Terminal Velocity
- Projectile Motion
- Forces in 2 Dimensions
- Impulse and Momentum Change
- Momentum Conservation
- Work-Energy Fundamentals
- Work-Energy Relationship
- Roller Coaster Physics
- Satellite Motion
- Electric Fields
- Circuit Concepts
- Series Circuits
- Parallel Circuits
- Describing-Waves
- Wave Behavior Toolkit
- Standing Wave Patterns
- Resonating Air Columns
- Wave Model of Light
- Plane Mirrors
- Curved Mirrors
- Teacher Guide
- Using Lab Notebooks
- Current Electricity
- Light Waves and Color
- Reflection and Ray Model of Light
- Refraction and Ray Model of Light
- Teacher Resources
- Subscriptions

- Newton's Laws
- Einstein's Theory of Special Relativity
- About Concept Checkers
- School Pricing
- Newton's Laws of Motion
- Newton's First Law
- Newton's Third Law
- The Speed of Sound
- Pitch and Frequency
- Intensity and the Decibel Scale
- The Human Ear
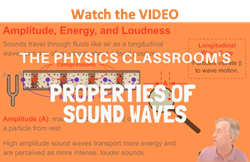
Since the speed of a wave is defined as the distance that a point on a wave (such as a compression or a rarefaction) travels per unit of time, it is often expressed in units of meters/second (abbreviated m/s). In equation form, this is
The faster a sound wave travels, the more distance it will cover in the same period of time. If a sound wave were observed to travel a distance of 700 meters in 2 seconds, then the speed of the wave would be 350 m/s. A slower wave would cover less distance - perhaps 660 meters - in the same time period of 2 seconds and thus have a speed of 330 m/s. Faster waves cover more distance in the same period of time.
Factors Affecting Wave Speed
The speed of any wave depends upon the properties of the medium through which the wave is traveling. Typically there are two essential types of properties that affect wave speed - inertial properties and elastic properties. Elastic properties are those properties related to the tendency of a material to maintain its shape and not deform whenever a force or stress is applied to it. A material such as steel will experience a very small deformation of shape (and dimension) when a stress is applied to it. Steel is a rigid material with a high elasticity. On the other hand, a material such as a rubber band is highly flexible; when a force is applied to stretch the rubber band, it deforms or changes its shape readily. A small stress on the rubber band causes a large deformation. Steel is considered to be a stiff or rigid material, whereas a rubber band is considered a flexible material. At the particle level, a stiff or rigid material is characterized by atoms and/or molecules with strong attractions for each other. When a force is applied in an attempt to stretch or deform the material, its strong particle interactions prevent this deformation and help the material maintain its shape. Rigid materials such as steel are considered to have a high elasticity. (Elastic modulus is the technical term). The phase of matter has a tremendous impact upon the elastic properties of the medium. In general, solids have the strongest interactions between particles, followed by liquids and then gases. For this reason, longitudinal sound waves travel faster in solids than they do in liquids than they do in gases. Even though the inertial factor may favor gases, the elastic factor has a greater influence on the speed ( v ) of a wave, thus yielding this general pattern:
Inertial properties are those properties related to the material's tendency to be sluggish to changes in its state of motion. The density of a medium is an example of an inertial property . The greater the inertia (i.e., mass density) of individual particles of the medium, the less responsive they will be to the interactions between neighboring particles and the slower that the wave will be. As stated above, sound waves travel faster in solids than they do in liquids than they do in gases. However, within a single phase of matter, the inertial property of density tends to be the property that has a greatest impact upon the speed of sound. A sound wave will travel faster in a less dense material than a more dense material. Thus, a sound wave will travel nearly three times faster in Helium than it will in air. This is mostly due to the lower mass of Helium particles as compared to air particles.
The Speed of Sound in Air
The speed of a sound wave in air depends upon the properties of the air, mostly the temperature, and to a lesser degree, the humidity. Humidity is the result of water vapor being present in air. Like any liquid, water has a tendency to evaporate. As it does, particles of gaseous water become mixed in the air. This additional matter will affect the mass density of the air (an inertial property). The temperature will affect the strength of the particle interactions (an elastic property). At normal atmospheric pressure, the temperature dependence of the speed of a sound wave through dry air is approximated by the following equation:
where T is the temperature of the air in degrees Celsius. Using this equation to determine the speed of a sound wave in air at a temperature of 20 degrees Celsius yields the following solution.
v = 331 m/s + (0.6 m/s/C)•(20 C)
v = 331 m/s + 12 m/s
v = 343 m/s
(The above equation relating the speed of a sound wave in air to the temperature provides reasonably accurate speed values for temperatures between 0 and 100 Celsius. The equation itself does not have any theoretical basis; it is simply the result of inspecting temperature-speed data for this temperature range. Other equations do exist that are based upon theoretical reasoning and provide accurate data for all temperatures. Nonetheless, the equation above will be sufficient for our use as introductory Physics students.)
Look It Up!
Using wave speed to determine distances.
At normal atmospheric pressure and a temperature of 20 degrees Celsius, a sound wave will travel at approximately 343 m/s; this is approximately equal to 750 miles/hour. While this speed may seem fast by human standards (the fastest humans can sprint at approximately 11 m/s and highway speeds are approximately 30 m/s), the speed of a sound wave is slow in comparison to the speed of a light wave. Light travels through air at a speed of approximately 300 000 000 m/s; this is nearly 900 000 times the speed of sound. For this reason, humans can observe a detectable time delay between the thunder and the lightning during a storm. The arrival of the light wave from the location of the lightning strike occurs in so little time that it is essentially negligible. Yet the arrival of the sound wave from the location of the lightning strike occurs much later. The time delay between the arrival of the light wave (lightning) and the arrival of the sound wave (thunder) allows a person to approximate his/her distance from the storm location. For instance if the thunder is heard 3 seconds after the lightning is seen, then sound (whose speed is approximated as 345 m/s) has traveled a distance of
If this value is converted to miles (divide by 1600 m/1 mi), then the storm is a distance of 0.65 miles away.
Another phenomenon related to the perception of time delays between two events is an echo . A person can often perceive a time delay between the production of a sound and the arrival of a reflection of that sound off a distant barrier. If you have ever made a holler within a canyon, perhaps you have heard an echo of your holler off a distant canyon wall. The time delay between the holler and the echo corresponds to the time for the holler to travel the round-trip distance to the canyon wall and back. A measurement of this time would allow a person to estimate the one-way distance to the canyon wall. For instance if an echo is heard 1.40 seconds after making the holler , then the distance to the canyon wall can be found as follows:
The canyon wall is 242 meters away. You might have noticed that the time of 0.70 seconds is used in the equation. Since the time delay corresponds to the time for the holler to travel the round-trip distance to the canyon wall and back, the one-way distance to the canyon wall corresponds to one-half the time delay.
While an echo is of relatively minimal importance to humans, echolocation is an essential trick of the trade for bats. Being a nocturnal creature, bats must use sound waves to navigate and hunt. They produce short bursts of ultrasonic sound waves that reflect off objects in their surroundings and return. Their detection of the time delay between the sending and receiving of the pulses allows a bat to approximate the distance to surrounding objects. Some bats, known as Doppler bats, are capable of detecting the speed and direction of any moving objects by monitoring the changes in frequency of the reflected pulses. These bats are utilizing the physics of the Doppler effect discussed in an earlier unit (and also to be discussed later in Lesson 3 ). This method of echolocation enables a bat to navigate and to hunt.
The Wave Equation Revisited
Like any wave, a sound wave has a speed that is mathematically related to the frequency and the wavelength of the wave. As discussed in a previous unit , the mathematical relationship between speed, frequency and wavelength is given by the following equation.
Using the symbols v , λ , and f , the equation can be rewritten as
Check Your Understanding
1. An automatic focus camera is able to focus on objects by use of an ultrasonic sound wave. The camera sends out sound waves that reflect off distant objects and return to the camera. A sensor detects the time it takes for the waves to return and then determines the distance an object is from the camera. If a sound wave (speed = 340 m/s) returns to the camera 0.150 seconds after leaving the camera, how far away is the object?
Answer = 25.5 m
The speed of the sound wave is 340 m/s. The distance can be found using d = v • t resulting in an answer of 25.5 m. Use 0.075 seconds for the time since 0.150 seconds refers to the round-trip distance.
2. On a hot summer day, a pesky little mosquito produced its warning sound near your ear. The sound is produced by the beating of its wings at a rate of about 600 wing beats per second.
a. What is the frequency in Hertz of the sound wave? b. Assuming the sound wave moves with a velocity of 350 m/s, what is the wavelength of the wave?
Part a Answer: 600 Hz (given)
Part b Answer: 0.583 meters
3. Doubling the frequency of a wave source doubles the speed of the waves.
a. True b. False
Doubling the frequency will halve the wavelength; speed is unaffected by the alteration in the frequency. The speed of a wave depends upon the properties of the medium.
4. Playing middle C on the piano keyboard produces a sound with a frequency of 256 Hz. Assuming the speed of sound in air is 345 m/s, determine the wavelength of the sound corresponding to the note of middle C.
Answer: 1.35 meters (rounded)
Let λ = wavelength. Use v = f • λ where v = 345 m/s and f = 256 Hz. Rearrange the equation to the form of λ = v / f. Substitute and solve.
5. Most people can detect frequencies as high as 20 000 Hz. Assuming the speed of sound in air is 345 m/s, determine the wavelength of the sound corresponding to this upper range of audible hearing.
Answer: 0.0173 meters (rounded)
Let λ = wavelength. Use v = f • λ where v = 345 m/s and f = 20 000 Hz. Rearrange the equation to the form of λ = v / f. Substitute and solve.
6. An elephant produces a 10 Hz sound wave. Assuming the speed of sound in air is 345 m/s, determine the wavelength of this infrasonic sound wave.
Answer: 34.5 meters
Let λ = wavelength. Use v = f • λ where v = 345 m/s and f = 10 Hz. Rearrange the equation to the form of λ = v / f. Substitute and solve.
7. Determine the speed of sound on a cold winter day (T=3 degrees C).
Answer: 332.8 m/s
The speed of sound in air is dependent upon the temperature of air. The dependence is expressed by the equation:
v = 331 m/s + (0.6 m/s/C) • T
where T is the temperature in Celsius. Substitute and solve.
v = 331 m/s + (0.6 m/s/C) • 3 C v = 331 m/s + 1.8 m/s v = 332.8 m/s
8. Miles Tugo is camping in Glacier National Park. In the midst of a glacier canyon, he makes a loud holler. He hears an echo 1.22 seconds later. The air temperature is 20 degrees C. How far away are the canyon walls?
Answer = 209 m
The speed of the sound wave at this temperature is 343 m/s (using the equation described in the Tutorial). The distance can be found using d = v • t resulting in an answer of 343 m. Use 0.61 second for the time since 1.22 seconds refers to the round-trip distance.
9. Two sound waves are traveling through a container of unknown gas. Wave A has a wavelength of 1.2 m. Wave B has a wavelength of 3.6 m. The velocity of wave B must be __________ the velocity of wave A.
a. one-ninth b. one-third c. the same as d. three times larger than
The speed of a wave does not depend upon its wavelength, but rather upon the properties of the medium. The medium has not changed, so neither has the speed.
10. Two sound waves are traveling through a container of unknown gas. Wave A has a wavelength of 1.2 m. Wave B has a wavelength of 3.6 m. The frequency of wave B must be __________ the frequency of wave A.
Since Wave B has three times the wavelength of Wave A, it must have one-third the frequency. Frequency and wavelength are inversely related.
- Interference and Beats

- Science Notes Posts
- Contact Science Notes
- Todd Helmenstine Biography
- Anne Helmenstine Biography
- Free Printable Periodic Tables (PDF and PNG)
- Periodic Table Wallpapers
- Interactive Periodic Table
- Periodic Table Posters
- Science Experiments for Kids
- How to Grow Crystals
- Chemistry Projects
- Fire and Flames Projects
- Holiday Science
- Chemistry Problems With Answers
- Physics Problems
- Unit Conversion Example Problems
- Chemistry Worksheets
- Biology Worksheets
- Periodic Table Worksheets
- Physical Science Worksheets
- Science Lab Worksheets
- My Amazon Books
Speed of Sound in Physics

In physics, the speed of sound is the distance traveled per unit of time by a sound wave through a medium. It is highest for stiff solids and lowest for gases. There is no sound or speed of sound in a vacuum because sound (unlike light ) requires a medium in order to propogate.
What Is the Speed of Sound?
Usually, conversations about the speed of sound refer to the speed of sound of dry air (humidity changes the value). The value depends on temperature.
- at 20 ° C or 68 ° F: 343 m/s or 1234.8 kph or 1125ft/s or 767 mph
- at 0 ° C or 32 ° F: 331 m/s or 1191.6 kph or 1086 ft/s or 740 mph
Mach Numher
The Mach number is the ratio of air speed to the speed of sound. So, an object at Mach 1 is traveling at the speed of sound. Exceeding Mach 1 is breaking the sound barrier or is supersonic . At Mach 2, the object travels twice the speed of sound. Mach 3 is three times the speed of sound, and so on.
Remember that the speed of sound depends on temperature, so you break sound barrier at a lower speed when the temperature is colder. To put it another way, it gets colder as you get higher in the atmosphere, so an aircraft might break the sound barrier at a higher altitude even if it does not increase its speed.
Solids, Liquids, and Gases
The speed of sound is greatest for solids, intermediate for liquids, and lowest for gases:
v solid > v liquid >v gas
Particles in a gas undergo elastic collisions and the particles are widely separated. In contrast, particles in a solid are locked into place (rigid or stiff), so a vibration readily transmits through chemical bonds.
Here are examples of the difference between the speed of sound in different materials:
- Diamond (solid): 12000 m/s
- Copper (solid): 6420 m/s
- Iron (solid): 5120 m/s
- Water (liquid) 1481 m/s
- Helium (gas): 965 m/s
- Dry air (gas): 343 m/s
Sounds waves transfer energy to matter via a compression wave (in all phases) and also shear wave (in solids). The pressure disturbs a particle, which then impacts its neighbor, and continues traveling through the medium. The speed is how quickly the wave moves, while the frequency is the number of vibrations the particle makes per unit of time.
The Hot Chocolate Effect
The hot chocolate effect describes the phenomenon where the pitch you hear from tapping a cup of hot liquid rises after adding a soluble powder (like cocoa powder into hot water). Stirring in the powder introduces gas bubbles that reduce the speed of sound of the liquid and lower the frequency (pitch) of the waves. Once the bubbles clear, the speed of sound and the frequency increase again.
Speed of Sound Formulas
There are several formulas for calculating the speed of sound. Here are a few of the most common ones:
For gases these approximations work in most situations:
For this formula, use the Celsius temperature of the gas.
v = 331 m/s + (0.6 m/s/C)•T
Here is another common formula:
v = (γRT) 1/2
- γ is the ratio of specific heat values or adiabatic index (1.4 for air at STP )
- R is a gas constant (282 m 2 /s 2 /K for air)
- T is the absolute temperature (Kelvin)
The Newton-Laplace formula works for both gases and liquids (fluids):
v = (K s /ρ) 1/2
- K s is the coefficient of stiffness or bulk modulus of elasticity for gases
- ρ is the density of the material
So solids, the situation is more complicated because shear waves play into the formula. There can be sound waves with different velocities, depending on the mode of deformation. The simplest formula is for one-dimensional solids, like a long rod of a material:
v = (E/ρ) 1/2
- E is Young’s modulus
Note that the speed of sound decreases with density! It increases according to the stiffness of a medium. This is not intuitively obvious, since often a dense material is also stiff. But, consider that the speed of sound in a diamond is much faster than the speed in iron. Diamond is less dense than iron and also stiffer.
Factors That Affect the Speed of Sound
The primary factors affecting the speed of sound of a fluid (gas or liquid) are its temperature and its chemical composition. There is a weak dependence on frequency and atmospheric pressure that is omitted from the simplest equations.
While sound travels only as compression waves in a fluid, it also travels as shear waves in a solid. So, a solid’s stiffness, density, and compressibility also factor into the speed of sound.
Speed of Sound on Mars
Thanks to the Perseverance rover, scientists know the speed of sound on Mars. The Martian atmosphere is much colder than Earth’s, its thin atmosphere has a much lower pressure, and it consists mainly of carbon dioxide rather than nitrogen. As expected, the speed of sound on Mars is slower than on Earth. It travels at around 240 m/s or about 30% slower than on Earth.
What scientists did not expect is that the speed of sound varies for different frequencies. A high pitched sound, like from the rover’s laser, travels faster at around 250 m/s. So, for example, if you listened to a symphony recording from a distance on Mars you’d hear the various instruments at different times. The explanation has to do with the vibrational modes of carbon dioxide, the primary component of the Martian atmosphere. Also, it’s worth noting that the atmospheric pressure is so low that there really isn’t any much sound at all from a source more than a few meters away.
Speed of Sound Example Problems
Find the speed of sound on a cold day when the temperature is 2 ° C.
The simplest formula for finding the answer is the approximation:
v = 331 m/s + (0.6 m/s/C) • T
Since the given temperature is already in Celsius, just plug in the value:
v = 331 m/s + (0.6 m/s/C) • 2 C = 331 m/s + 1.2 m/s = 332.2 m/s
You’re hiking in a canyon, yell “hello”, and hear an echo after 1.22 seconds. The air temperature is 20 ° C. How far away is the canyon wall?
The first step is finding the speed of sound at the temperature:
v = 331 m/s + (0.6 m/s/C) • T v = 331 m/s + (0.6 m/s/C) • 20 C = 343 m/s (which you might have memorized as the usual speed of sound)
Next, find the distance using the formula:
d = v• T d = 343 m/s • 1.22 s = 418.46 m
But, this is the round-trip distance! The distance to the canyon wall is half of this or 209 meters.
If you double the frequency of sound, it double the speed of its waves. True or false?
This is (mostly) false. Doubling the frequency halves the wavelength, but the speed depends on the properties of the medium and not its frequency or wavelength. Frequency only affects the speed of sound for certain media (like the carbon dioxide atmosphere of Mars).
- Everest, F. (2001). The Master Handbook of Acoustics . New York: McGraw-Hill. ISBN 978-0-07-136097-5.
- Kinsler, L.E.; Frey, A.R.; Coppens, A.B.; Sanders, J.V. (2000). Fundamentals of Acoustics (4th ed.). New York: John Wiley & Sons. ISBN 0-471-84789-5.
- Maurice, S.; et al. (2022). “In situ recording of Mars soundscape:. Nature. 605: 653-658. doi: 10.1038/s41586-022-04679-0
- Wong, George S. K.; Zhu, Shi-ming (1995). “Speed of sound in seawater as a function of salinity, temperature, and pressure”. The Journal of the Acoustical Society of America . 97 (3): 1732. doi: 10.1121/1.413048
Related Posts
This is a simulation of a standard physics demonstration to measure the speed of sound in air. A vibrating tuning fork is held above a tube - the tube has some water in it, and the level of the water in the tube can be adjusted. This gives a column of air in the tube, between the top of the water and the top of the tube. By setting the water level appropriately, the height of the air column can be such that it gives a resonance condition for the sound wave produced by the tuning fork. In the real experiment, resonance is found by listening - the sound from the tube is loudest at resonance. In the simulation, resonance is shown by the amplitude of the wave in the air column. The larger the amplitude, the closer to resonance. Note that at certain special heights of the air column, no sound is heard - this is because of completely destructive interference.
In addition, there is always a node (for displacement of the air molecules) at the water surface. To a first approximation, resonance occurs when there is an anti-node at the top of the tube. Knowing the frequency of the tuning fork, the height of the air column, and the appropriate equation for standing waves in a tube like this, the speed of sound in air can be determined experimentally. What do you get for the speed of sound in air in this simulation?

Accessibility Links
- Skip to content
- Skip to search IOPscience
- Skip to Journals list
- Accessibility help
- Accessibility Help
Click here to close this panel.
Purpose-led Publishing is a coalition of three not-for-profit publishers in the field of physical sciences: AIP Publishing, the American Physical Society and IOP Publishing.
Together, as publishers that will always put purpose above profit, we have defined a set of industry standards that underpin high-quality, ethical scholarly communications.
We are proudly declaring that science is our only shareholder.
Measuring the speed of sound in air using a smartphone and a cardboard tube
Simen Hellesund 1
Published 4 April 2019 • © 2019 IOP Publishing Ltd Physics Education , Volume 54 , Number 3 Citation Simen Hellesund 2019 Phys. Educ. 54 035015 DOI 10.1088/1361-6552/ab0e21
Article metrics
31800 Total downloads
Share this article
Author e-mails.
Author affiliations
1 University of Oslo, Oslo, Norway
Simen Hellesund https://orcid.org/0000-0002-4424-4643
- Received 22 January 2019
- Accepted 8 March 2019
- Published 4 April 2019
Peer review information
Method : Single-anonymous Revisions: 1 Screened for originality? Yes
Buy this article in print
This paper demonstrates a variation on the classic Kundt's tube experiment for measuring the speed of sound. The speed of sound in air is measured using a smartphone and a cardboard tube, making the experiment very economical in terms of equipment. The speed of sound in air is measured to within 3% of the theoretical prediction.
Export citation and abstract BibTeX RIS
Content from this work may be used under the terms of the Creative Commons Attribution 3.0 licence . Any further distribution of this work must maintain attribution to the author(s) and the title of the work, journal citation and DOI.
Introduction
In recent years, smartphones have become packed with sensors; microphones, cameras, accelerometers, magnetometers, gyroscopes, thermometers, proximity sensors etc. Having become ubiquitous in society, smartphones can provide economical alternatives to expensive laboratory equipment in physics education.
Several papers have examined the use of smartphones in acoustic experiments for educational purposes [ 1 – 5 ]. A common experiment in physics education is to measure the speed of sound c in air, or other gasses, by observing standing acoustic waves in a tube. August Kundt first described this experiment in 1866 [ 6 ]. Such an experiment is therefore often referred to as Kundt's tube .
Parolin and Pezzi have shown how the experiment can be performed using two smartphones [ 7 ]. Yavuz has shown how it can be done using a single smartphone by partially submerging the tube in water [ 8 ]. The aim of this paper is to attempt to perform the experiment using only a smartphone and a cardboard tube, offering an alternative method to the one outlined in [ 8 ].
This means that if we can determine both the frequency and wavelength of the wave, we can measure the speed of sound in the medium. For this experiment, the medium in question is air at room temperature and atmospheric pressure.
When an acoustic wave enters through the open end of a half-closed tube and hits the closed end, part of the wave is reflected back down the tube towards the open end. At specific wavelengths, the incident and the reflected wave form a standing wave. In the antinodes of the standing wave, the points on the standing wave where the amplitude is maximal, the amplitude of the standing wave is greater than the amplitude of the incident wave alone. The opening of the tube will always be a displacement antinode of the standing waves. The wavelengths at which the standing waves occur are called the resonance wavelengths of the tube. For the half-closed tube, the resonances occur when the length of the tube equals an odd number of quarter wavelengths of the incident wave:
The number n is often referred to as the nth harmonic of the tube. L is the length of the tube.
The resonance frequencies f n of the tube, the frequencies at which standing waves occur in the tube, can be found by combining equations ( 1 ) and ( 2 ):
We see that the resonance frequencies as a function of n is the equation of a straight line. The slope a of this line is given by
This means that if we can identify the resonance frequencies of the tube and fit a straight line to them, we can calculate the speed of sound as
The following equipment is used for this experiment: a smartphone with a signal generator app and a recording app installed; a cardboard tube, closed in one end; a thermometer; a tape measure; a computer.
The smartphone used is a Motorola Moto g6 Plus. There are several apps available both for generating audio sine waves and for recording. The function generator app must be able to perform a sweep over frequencies. The generator app used in this experiment is called Function Generator and is available in the Google Play Store [ 10 ]. The recording app must record audio with a sampling frequency known to the user. The recording app used for this experiment is called Smart Recorder , also available in the Google Play Store [ 11 ]. For users of Apple products, the recording app Voice Recorder Lite: HD Audio Recording & Playback [ 12 ] and the signal generator Audio Function Generator [ 13 ], both available from the Apple App Store, may be used.
The cardboard tube used is one intended for storing or shipping posters. One end is stopped by a plastic plug. The length of the tube is measured, using the tape measure, to be 47.6 cm. The inner diameter of the tube is measured to be 7.5 cm. The tube and the smartphone used in the experiment can be seen in figure 1 .
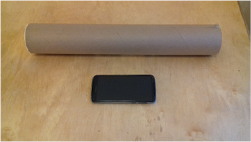
Figure 1. The smartphone and the cardboard tube used in the experiment.
Download figure:
The experiment is performed in an anechoic chamber at the physics department at the University of Oslo. Having access to such a room is not critical to the experiment, although one should aim to limit background noise as much as possible.
We place the smartphone such that the microphone is located in the opening of the tube. This is shown in figure 2 . The phone is set to record audio with a sampling frequency of 44.1 kHz. While the phone is recording, the function generator app emits a pure sine wave. The sine wave sweeps from 50 Hz to 3000 Hz at a rate of 1 Hz s −1 . The audio recording is stored in .wav format. This format makes for easy data analysis later.
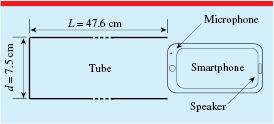
Figure 2. Schematic illustrating the placement of the tube and smartphone during the experiment.
Measuring frequency
We need to know the frequencies at which the resonances occur. The function generator does not store any information on which frequency is emitted at which time. Thus, the frequency has to be inferred from the recording.
Frequency cannot be instantaneously measured. The signal must be monitored over some period of time to count how many times it oscillates per second.
In this case, where we are trying to identify a pure sine wave in a high signal to noise ratio environment, we can use the method known as the zero crossing method (ZCM) [ 14 ]. The ZCM works by determining the points in time where the waveform of the recording crosses from negative to positive values (or vice versa). These points are approximated by identifying the points on either side of such a crossing and drawing a straight line between them. The zero crossing point of the signal is approximated as the point at which this straight line becomes zero. The distance from one such zero crossing point to the next is an approximation of the period of oscillation of the signal. The inverse of this period is the frequency of the signal. The ZCM procedure is illustrated in figure 3 .
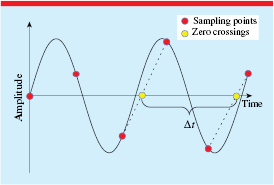
Figure 3. The ZCM illustrated. The black line is the pure sine wave signal. The red points are the points sampled by the smartphone. The yellow points are the zero crossing points used to approximate the frequency of the signal.
The samples of the audio recording are split into one-second intervals. In each of these intervals, the ZCM is used to find all the zero crossings. The measured frequency in each interval is taken to be the mean of all the frequencies measured from these zero crossings. These frequencies are plotted as a function of time in figure 4 .
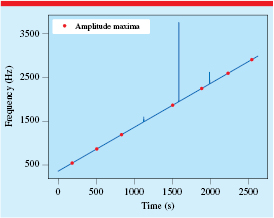
Figure 4. Frequencies measured using the ZCM.
Looking at figure 4 , we notice that there are a few points where the ZCM clearly fails to identify the frequency emitted by the function generator. In our case, these points can be ignored, as they are far away from the points where the sound intensity of the recording peaks.
The results obtained using the ZCM are cross-checked by measuring the frequency in the recording using a fast fourier transfer (FFT) in each second interval. The most prominent frequency in the FFT spectrogram of each interval is taken to be the frequency of the signal. This method yields identical results to those obtained by the ZCM. However, the FFT method is significantly slower and is therefore not used in the final analysis.
Data analysis
The start of the audio recording proves too noisy to be of use. The low frequencies emitted by the function generator are perhaps causing the smartphone to vibrate. This region of the recording is therefore cut before the analysis.
The data analysis is performed in Python. The SciPy python library contains the packages needed for reading the .wav file, as well as for signal processing and curve fitting [ 15 ].
We need to identify the points where the sound intensity of the recording is maximal, as these maxima will occur at the resonance frequencies of the tube. First, the recording is split into the same one-second increments used to measure the frequency. The absolute value of the digital samples is taken in each of these intervals. The peaks in the resulting signal are identified using the find_peaks function of the SciPy signal processing package. The mean height of the peaks found in each second increment is taken to be the sound amplitude. The resulting distribution is shown in figure 5 .
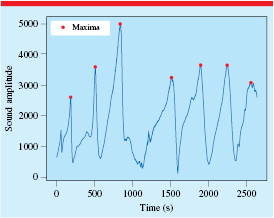
Figure 5. Sound amplitude of the audio recording.
Next, the find_peaks function is used once more to identify the peaks in the sound amplitude distribution. These peaks are also shown in figure 5 . The frequencies measured at the times of these peaks are the resonance frequencies of the cardboard tube. These frequencies are recorded in table 1 in the results section. It seems one resonance frequency fails to give a peak in the sound amplitude distribution. The reason for this is unknown. The frequencies at n = 7 and above are therefore shifted up by one.
Table 1. Frequencies at peak amplitudes.
Frequency (Hz) | |
---|---|
1 | 544 |
3 | 867 |
5 | 1130 |
7 | Unknown |
9 | 1865 |
11 | 2247 |
13 | 2594 |
15 | 2906 |
The resonance frequencies identified in the previous section are listen in table 1 . A straight line is fitted to these frequencies as a function of n using the polyfit function of the Python module NumPy [ 16 ]. The frequencies as well as the line of best fit is shown in figure 6 . The slope of the straight line is used to calculate c using equation ( 6 ). The speed of sound in air at 24 °C is measured to be c = 335 m s −1 .
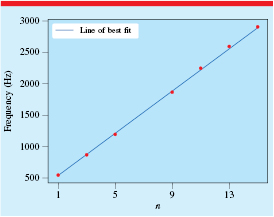
Figure 6. Resonance frequencies of the cardboard tube with line of best fit.
In this paper, a method for measuring the speed of sound in air has been demonstrated using a smartphone and a cardboard tube. The experiment is very economical, and can thus be performed in places with limited access to laboratory equipment.
The experiment requires some amount of programming. It can provide learning opportunities for students both in experimental methods as well as in data analysis.
Biographies

Simen Hellesund is a PhD student at the University of Oslo. His main research interest is experimental high energy physics, and he is a member of the ATLAS collaboration at CERN. He also enjoys teaching physics and is researching ways to improve physics education. In particular, he is interested in how to perform physics experiments or measurements for educational purposes, with limited access to laboratory equipment.
Remember Me
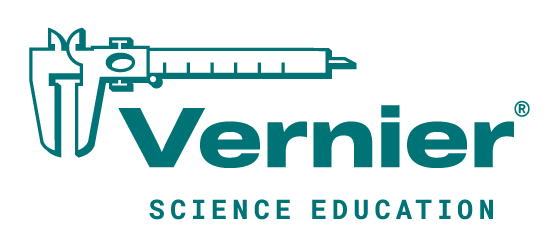
Shop Experiment Speed of Sound Experiments
Speed of sound.
Experiment #33 from Physics with Vernier
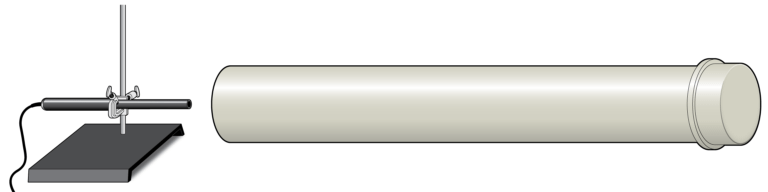
Introduction
Compared to most things you study in the physics lab, sound waves travel very fast. It is fast enough that measuring the speed of sound is a technical challenge. One method you could use would be to time an echo. For example, if you were in an open field with a large building a quarter of a kilometer away, you could start a stopwatch when a loud noise was made and stop it when you heard the echo. You could then calculate the speed of sound.
To use the same technique over short distances, you need a faster timing system, such as a data-collection interface. In this experiment, you will use this technique with a Microphone connected to an interface to determine the speed of sound at room temperature. The Microphone will be placed next to the opening of a hollow tube. When you make a sound by snapping your fingers next to the opening, the computer will begin collecting data. After the sound reflects off the opposite end of the tube, a graph will be displayed showing the initial sound and the echo. You will then be able to determine the round trip time and calculate the speed of sound.
- Measure how long it takes sound to travel down and back in a long tube.
- Determine the speed of sound.
- Compare the speed of sound in air to the accepted value.
Sensors and Equipment
This experiment features the following sensors and equipment. Additional equipment may be required.
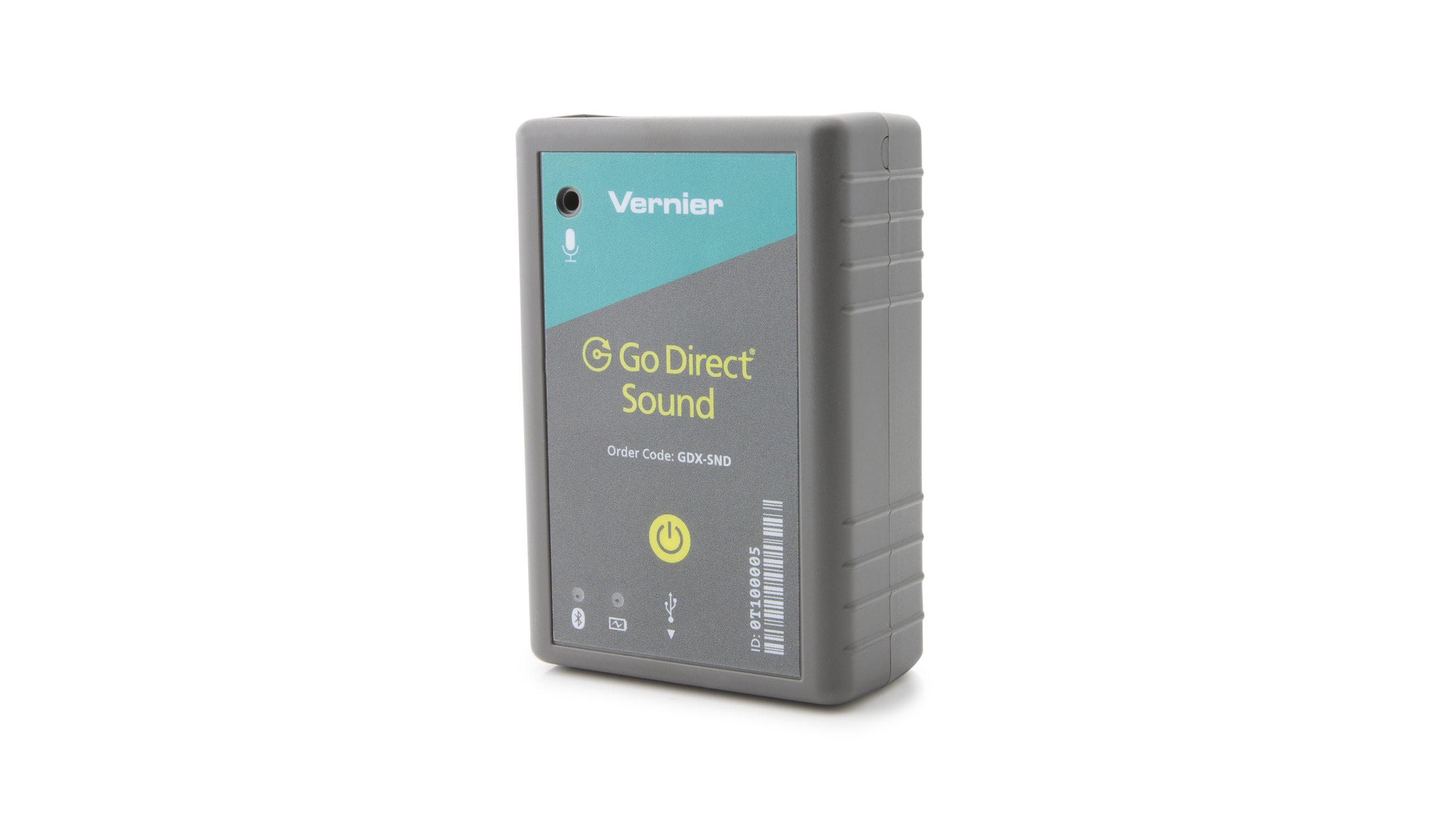
Correlations
Teaching to an educational standard? This experiment supports the standards below.

Ready to Experiment?
Ask an expert.
Get answers to your questions about how to teach this experiment with our support team.
- Call toll-free: 888-837-6437
- Chat with Us
- Email [email protected]
Purchase the Lab Book
This experiment is #33 of Physics with Vernier . The experiment in the book includes student instructions as well as instructor information for set up, helpful hints, and sample graphs and data.
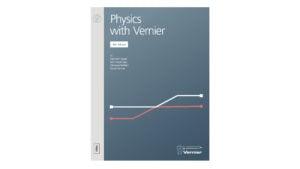
Measuring the Speed of Sound ( CIE IGCSE Physics )
Revision note.
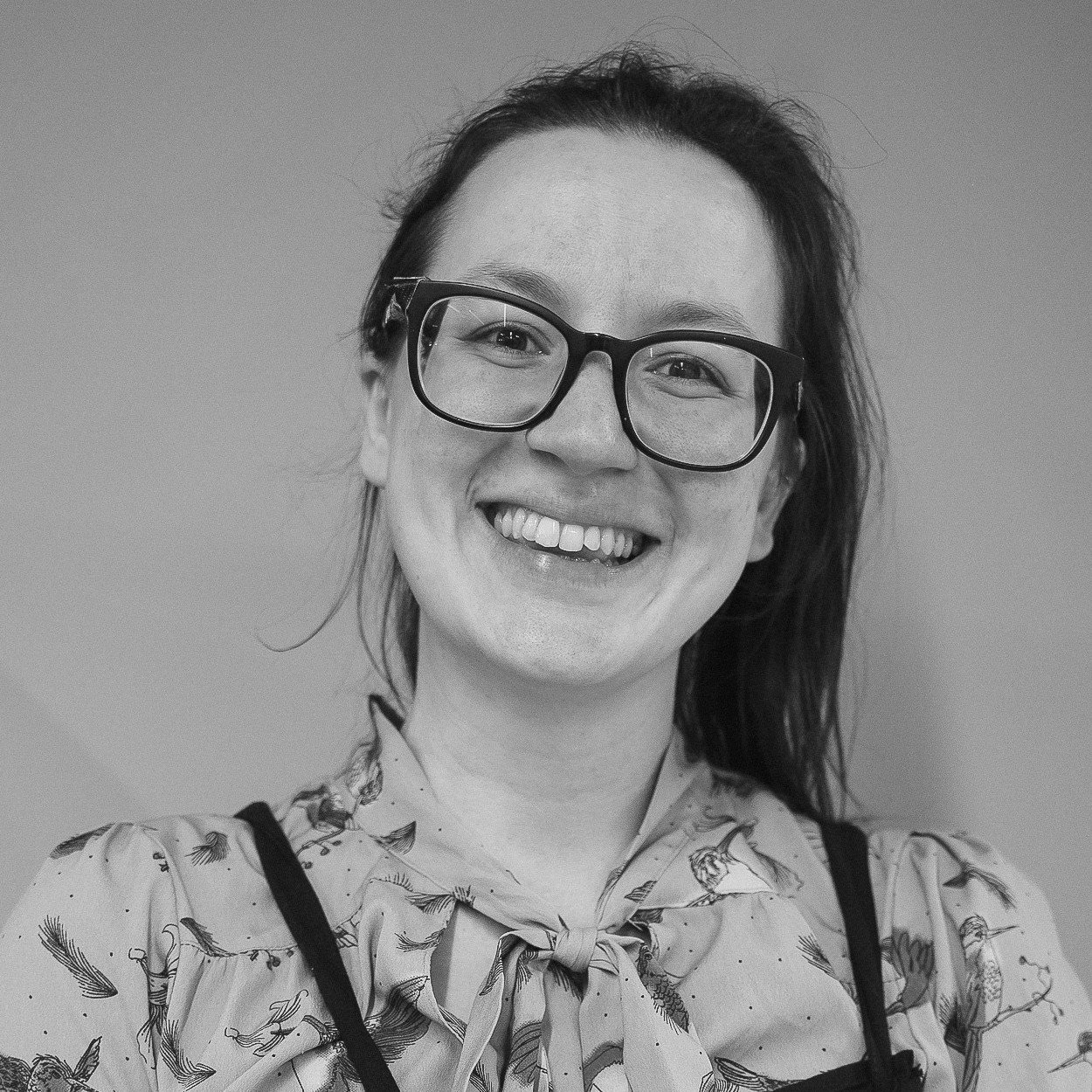
Speed of sound in air
- The higher the air temperature , the greater the speed of sound
Speed of sound in materials
Extended tier only
- Sound travels fastest in solids
- Sound travels slowest in gases
- Around 1500 m/s in liquids
- Around 5000 m/s in solids
Measuring the speed of sound
Equipment list.
Trundle Wheel | To measure the distance travelled by the sound waves |
Wooden Blocks | To create a sound when banged together |
Stopwatch | To time how long it takes the sound waves to travel |
Oscilloscope | To display the sound wave electronically |
Microphones x2 | To detect sound waves and turn them into an electrical signal |
Tape Measure | To measure the distance between microphones |
- Trundle wheel = 0.01 m
- Tape measure = 0.1 cm
- Stopwatch = 0.01 s
Experiment 1: measuring the speed of sound between two points
- This experiment aims to measure the speed of sound in air between two points
- Independent variable = Distance
- Dependent variable = Time
- Same location to carry out the experiment
Measuring the speed of sound in air
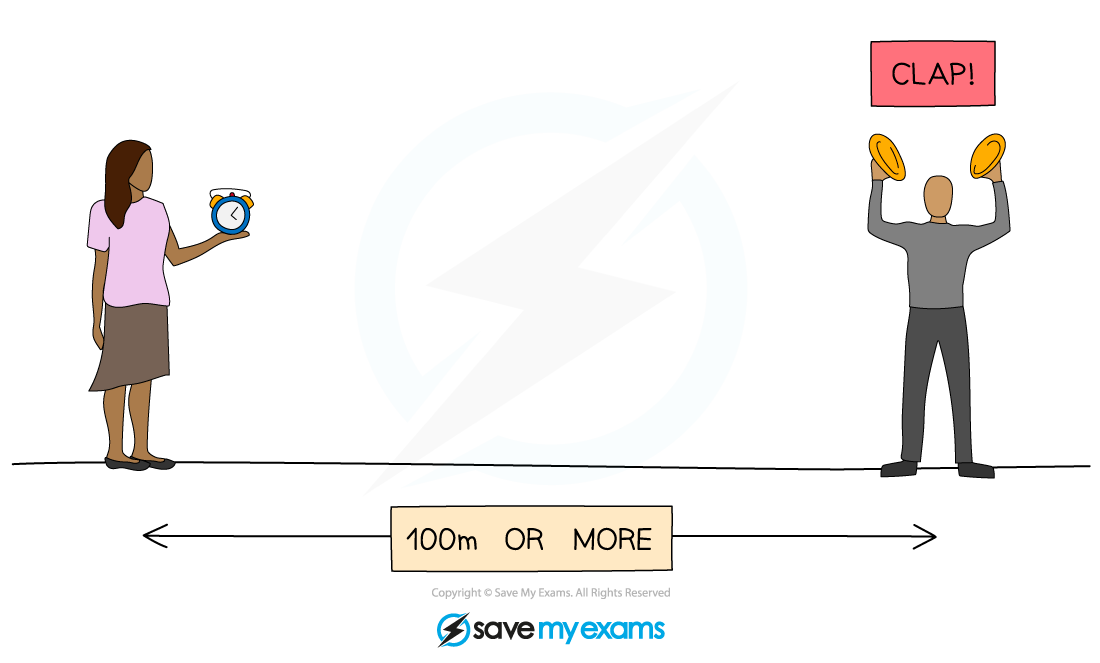
Measuring the speed of sound directly between two points
- Use the trundle wheel to measure a distance of 100 m between two people
- One of the people should have two wooden blocks, which they will bang together above their head to generate sound waves
- The second person should have a stopwatch which they start when they see the first person banging the blocks together and stop when they hear the sound
- This should be repeated several times and an average taken for the time travelled by the sound waves
- Repeat this experiment for various distances, e.g. 120 m, 140 m, 160 m, 180 m
An example results table for the speed of sound in air
100 | ||||
120 | ||||
140 | ||||
160 | ||||
180 |
Analysis of results
- The speed of sound can be calculated using the equation:
- The speed of sound in the air should work out to be about 340 m/s
Experiment 2: measuring the speed of sound with oscilloscopes
- This experiment aims to measure the speed of sound in air between two points using an oscilloscope
- Same set of microphones for each trial
Measuring the speed of sound with an oscilloscope
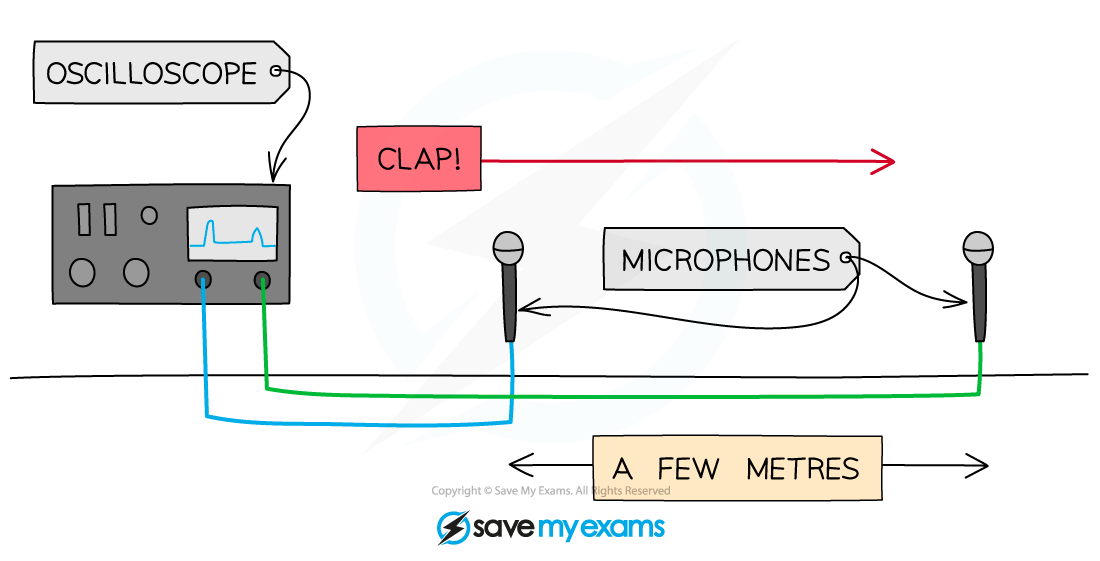
Measuring the speed of sound using an oscilloscope
- Connect two microphones to an oscilloscope
- Place them about 2 m apart using a tape measure to measure the distance between them
- Set up the oscilloscope so that it triggers when the first microphone detects a sound, and adjust the time base so that the sound arriving at both microphones can be seen on the screen
- Make a large clap using the two wooden blocks next to the first microphone
- Use the oscilloscope to determine the time at which the clap reaches each microphone and the time difference between them
- Repeat this experiment for several distances, e.g. 2 m, 2.5 m, 3 m, 3.5 m
An example results table for obtaining the speed of sound using an oscilloscope
2.0 | ||||
2.5 | ||||
3.0 | ||||
3.5 | ||||
4.0 |
Evaluating the experiments
Systematic Errors :
- The scale is likely to be small (e.g. milliseconds) so ensure this is taken into account when calculating speed
Random errors :
- Ensure to take repeat readings when timing intervals and calculate an average to keep this error to a minimum
- Maximise the distance between the two people where possible. This will reduce the error in measurements of time because the time taken by the sound waves to travel will be greater
When answering questions about methods to measure waves, the question could ask you to comment on the accuracy of the measurements.
In the case of measuring the speed of sound:
- Experiment 2 is the most accurate because the timing is done automatically
- Experiment 1 is the least accurate because the time interval is very short
Whilst this may not be too important when giving a method, you should be able to explain why each method is accurate or inaccurate and suggest ways of making them better (use bigger distances)
- For example, if a manual stopwatch is being used there could be variation in the time measured which can be up to 0.2 seconds due to a person's reaction time
- The time interval could be as little as 0.3 seconds for sound travelling in the air
- This means that the variation due to the stopwatch readings has a big influence on the results and they may not be reliable
You've read 0 of your 10 free revision notes
Get unlimited access.
to absolutely everything:
- Downloadable PDFs
- Unlimited Revision Notes
- Topic Questions
- Past Papers
- Model Answers
- Videos (Maths and Science)
Join the 100,000 + Students that ❤️ Save My Exams
the (exam) results speak for themselves:
Did this page help you?
Author: Katie M
Katie has always been passionate about the sciences, and completed a degree in Astrophysics at Sheffield University. She decided that she wanted to inspire other young people, so moved to Bristol to complete a PGCE in Secondary Science. She particularly loves creating fun and absorbing materials to help students achieve their exam potential.
- CBSE Class 11
- CBSE Class 11 Physics Practical
- To Find The Speed Of Sound In Air At Room Temperature Using A Resonance Tube By Two Resonance Positions
To Find the Speed of Sound in Air at Room Temperature Using a Resonance Tube by Two Resonance Positions
To find the speed of sound in air at room temperature using a resonance tube by two resonance positions.
Apparatus/Materials Required
- Resonance tube
- Thermometer
- Set Squares
- Water in a beaker
- Two-timing forks of known frequency
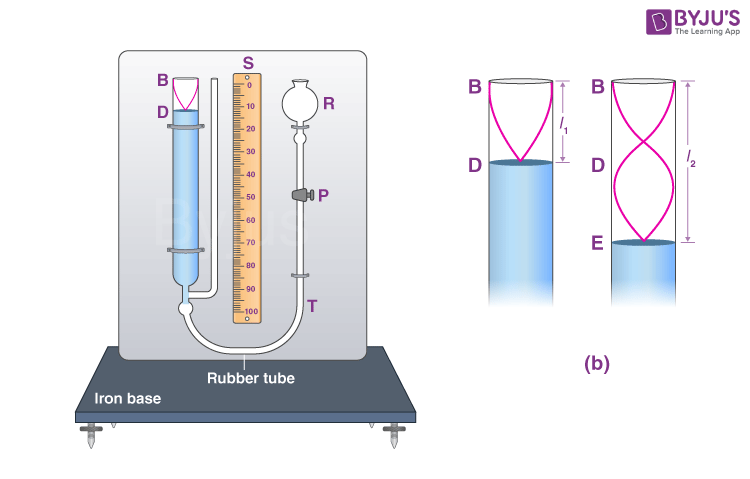
Let l 1 and l 2 be the length of the air column for the first and second resonance respectively with a tuning fork of frequency f .
The speed is given by the formula
Substituting, we get
- By making base horizontal with the help of levelling screws, set the resonance tube vertical.
- Fix the reservoir R in the uppermost position.
- Loosen the pinch cock P and fill the reservoir and metallic tube completely with water by a beaker.
- Tighten the pinch cock, lower the reservoir and fix it in the lowest position.
- Take a tuning fork of higher frequency
Observation
The temperature of the air in the air column:
(i) in the beginning ____ °C
(ii) at the end _____°C
The mean temperature is calculated as follows:
Frequency of first tuning fork = f 1
Frequency of second tuning fork = f 2
| ||||||
| ||||||
| ||||||
| ||||||
|
Calculation
From the first tuning fork,
From the second tuning fork,
The mean velocity at room temperature is given as follows:
At room temperature, the velocity of sound in air is _____ m/s.
1. What is the working principle of the resonance tube?
It works on the principle of resonance of the air column with a tuning fork.
2. What types of waves are produced in the air column?
Longitudinal stationary waves are produced in the air column.
3. Do you find the velocity of sound in air column or in the water column?
The velocity of sound is found in the air column above the water column.
4. What are the possible errors in the result?
The two possible errors in the result are:
(i) The enclosed air in the air column is denser than the outside air, this may reduce the velocity of air.
(ii) The humidity above the enclosed water column may increase the velocity of sound.
5. Will the result be affected if we take other liquids than water?
No, it will not be affected.
Sound Visualisation
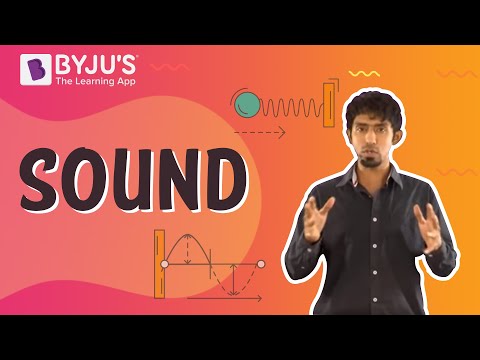
Stay tuned with BYJU’S to get the latest notification on CBSE along with CBSE syllabus, sample papers, marking scheme and more.
|
|
|
|
|
|
|
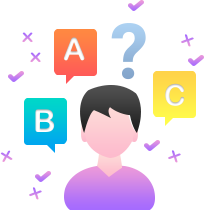
Put your understanding of this concept to test by answering a few MCQs. Click ‘Start Quiz’ to begin!
Select the correct answer and click on the “Finish” button Check your score and answers at the end of the quiz
Visit BYJU’S for all Physics related queries and study materials
Your result is as below
Request OTP on Voice Call
PHYSICS Related Links | |
Leave a Comment Cancel reply
Your Mobile number and Email id will not be published. Required fields are marked *
Post My Comment

Register with BYJU'S & Download Free PDFs
Register with byju's & watch live videos.
Talk to our experts
1800-120-456-456
- Measuring the Speed of Sound
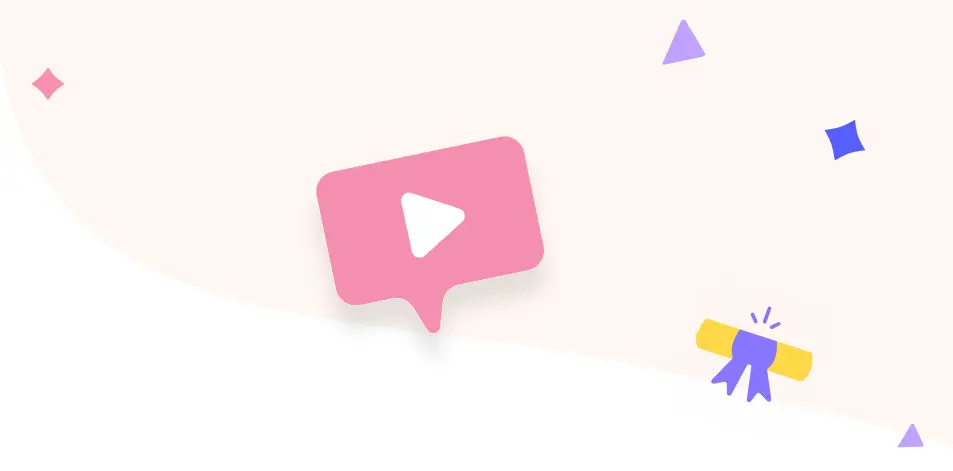
Acoustic Sound Meaning
The word “acoustic” is related to sound or the sense of hearing. It is a branch of sound that deals with understudies of mechanical waves in solids, liquids, and gases. These mechanical waves can be of sound, vibrations, ultrasound, and infrasound.
Since we are dealing with sound waves, so we will talk about Acoustic Sound. Sound travelling in the form of waves has some speed that can be in m/s, kmph, and mph.
Speed of sound is the distance travelled by a unit wave through the air/elastic medium carrying various units of measurement.
On this page, you will find multiple units of sound with the experiment to measure speed of sound in air.
Important Point:
In the 17th century, the French scientist and philosopher Pierre Gassendi is known to be the dist to attempt the measuring the speed of sound in air.
Speed of Sound in Air
For measuring the speed of sound, we need to measure the distance it covers through the medium.
For instance, the speed of sound in meters per second (in dry air) is 343 meters per second; however, this velocity value is considered at a temperature of 20 0 C (68 0 F).
Moving forward, the speed of sound is measurable in various media and units like we discussed for m/s (m/s = SI unit of speed).
Now, let’s discuss the speed of sound in miles per hour, speed of sound km per hour, and how the experiment for the determination of the speed of sound can be performed:
Speed of Sound in Various Units
The speed of sound is measured in the following units:
Speed of sound in Km per hour - 1,235 Kmph
Speed of Sound in miles per hour - 767 mph
Speed of sound in air feet per second - 1,125 ft s⁻¹
The velocity of sound is measured in hydrogen and oxygen (H 2 and O 2 ) is always 332 m/s.
Before starting with the experiment to measure the speed of sound, we must know the sound measurements symbols:
Measuring the Speed of Sound in Air
Characteristic | Symbols |
Sound Pressure | p, SPL, LPA |
Particle Velocity | v, SVL |
Particle Displacement | δ |
Sound Intensity | I, SIL |
Sound Power | P, SWL, LWA |
Sound Energy | W |
Sound Energy Density | w |
Sound Exposure | E, SEL |
Acoustic Impedance | Z |
Audio frequency | AL |
Transmission Loss | TL |
Point to Note:
The speed of sound strongly depends on the temperature and the medium through which it propagates.
Do You Know the Speed of Sound is Measured by Which Instrument?
We can measure the speed of sound by using an oscilloscope, a square-wave oscillator, and a piezo-electric pick-up.
A study of the connection between the space travelled, and therefore, the time of arrival of the sound wave allows a graphical determination of the speed of the heartbeat within the lucite rod.
Now, let us understand the experiment to measure speed of sound in air:
Experiment to Determine the Speed of Sound
Theory .
In 1866, August Kundt first described this experiment. In this context, we will be performing a common experiment in physics. The acoustic tube is also known as the Kundt’s tube.
Aim of this Experiment:
This experiment aims to measure the speed of sound “c” in air, or other gasses, by observing standing acoustic waves in a tube.
[Image will be Uploaded Soon]
Do You Know?
We can determine the speed of sound in the air by using a smartphone and a cardboard tube.
However, for making the experiment economical/affordable in terms of equipment. We are measuring the speed of sound within 3% of the theoretical prediction.
Theory of the Experiment:
Now, start with the theoretical part:
We place the smartphone such the microphone is found within the opening of the tube. this is shown in the figure below:
[Image will be Uploaded Soon]
The phone is about to record audio with a frequency of 44.1 kHz. During the phone recording, the function generator app emits a pure wave.
The wave traverses a path from 50 Hz to 3000 Hz at a rate of 1 Hz s −1 . The sound recording is stored in .wave format. This format makes for straightforward data analysis later.
For a sinusoidal wave with constant frequency f and wavelength , propagating in a medium, the speed of sound in the said medium is given by:
c = fλ
On determining the wave’s frequency and wavelength, we can measure the speed of sound in the medium.
When an acoustic wave enters through the open end of a half-closed tube and hits the closed-end, part of the wave is reflected towards the tube’s opened end. At specific wavelengths, the incident and the reflected wave form a standing wave.
In the antinodes of the standing wave, the points on the standing wave where the amplitude is maximum, the amplitude of the standing wave is greater than the amplitude of the incident wave.
The displacement antinode of the standing waves is the opening of the tube. The resonance wavelengths are the wavelengths at which the standing waves occur.
For the half-closed tube, the resonances occur when the tube’s length equals an odd number of quarter wavelengths of the incident wave:
λ n = 4L/n,
n = 1, 3, 5,....
“n” is the nth harmonic of the tube
L is the length of the tube.
The resonance frequencies f n of the tube, the frequencies at which standing waves occur in the tube, can be found by combining both equations:
λ n = 4cL/n,
n = 1, 3, 5,.....
Frequencies at peak amplitudes:
n | Frequency |
1 | 544 |
3 | 867 |
5 | 1130 |
7 | - |
9 | 1865 |
11 | 2247 |
13 | 2594 |
15 | 2906 |
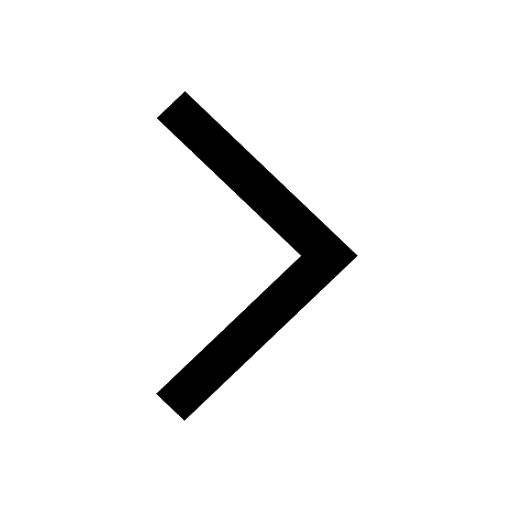
FAQs on Measuring the Speed of Sound
Q1: What is Acoustic?
Ans: Acoustic is a part of science that deals with the study of sound, its production, transmission, and effects.
A scientist or researcher who studies acoustics is called an Acoustician, whole someone working in the field of acoustics technology will be called Acoustical Engineering.
Q2: What is Acoustic Physics?
Ans: Acoustics is the science that concerns production, control, transmission, reception, and sound effects.
The term “acoustic,” is derived from the Greek word “akoustos,” meaning “heard.”.
Application of Acoustic
The main functioning of acoustics is to make the music or speech sound as good as possible (the high-quality sound or music).
We can achieve acoustic by reducing the sound barriers and increasing the factors that help in the smooth transmission of sound waves.
Q3: What is the Process of Acoustic Energy?
Ans: Acoustic energy is the energy concerning the mechanical vibrations from its components.
Any acoustic event has the following stages. The process in which a form of energy is transformed into sonic energy for producing a sound wave is called the transduction process.
Q4: What is the Speed of Sound at 25 Degrees Celsius?
Ans: The speed of sound in the air is 346 m/s at 25 °C and the speed of sound in the air is 386 m/s at 100 °C.
Now, if the sound of speed in the air is increased by 0.60 m/s, then for each increase of degree in air temperature, the speed of sound also increases because, at higher temperatures, molecules collide more often.

Teacher Resource Center
Pasco partnerships.

2024 Catalogs & Brochures
Measuring the speed of sound with an echo.
Students will first predict the speed of sound in the air of their classroom using a simple relationship accounting for temperature. Students will measure the time it takes a short pulse of sound to travel the length of a tube, reflect off the closed end, and return. Using this measurement, they will calculate the speed of sound and compare it to their prediction.
Grade Level: High School
Subject: Physics
Student Files
94.10 KB | ||
134.70 KB |
Teacher Files
Sign In to your PASCO account to access teacher files and sample data.
Watch Video
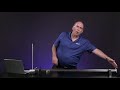
Measuring the Speed of Sound Using an Echo
Measure the time it takes a short pulse of sound to travel the length of a tube, reflect off the closed end, and return. Using this measurement, calculate the...
Featured Equipment
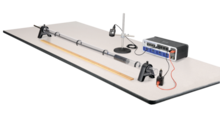
Resonance Air Column
Resonance tube with plunger, node markers and detachable stands for performing waves and sound experiments.
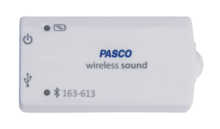
Wireless Sound Sensor
The Wireless Sound Sensor measures sound wave forms and sound level in one compact and portable sensor.
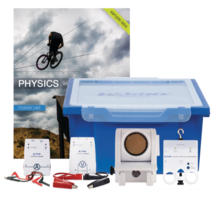
Physics Starter Lab Station
This starter Lab Station includes the wireless motion, force, current and voltage sensors used to perform some of the key lab activities from the Physics Lab Station Investigations manual.
Many lab activities can be conducted with our Wireless , PASPORT , or even ScienceWorkshop sensors and equipment. For assistance with substituting compatible instruments, contact PASCO Technical Support . We're here to help. Copyright © 2020 PASCO
Source Collection: Lab #13
Physics Lab Station Investigations
Source Collection: Lab #03
Physics Lab Station: Waves and Sound
More experiments.
Advanced Placement
- Magnetic Fields
- Momentum and Explosions
- Electric Field Mapping
- LRC Series Circuit
- Driven Damped Harmonic Oscillations
High School
June 9, 2003
How were the speed of sound and the speed of light determined and measured?
Chris Oates, a physicist in the Time and Frequency Division of the National Institute of Standards and Technology (NIST), explains.
Despite the differences between light and sound, the same two basic methods have been used in most measurements of their respective speeds. The first method is based on simply measuring the time it takes a pulse of light or sound to traverse a known distance; dividing the distance by the transit time then gives the speed. The second method makes use of the wave nature common to these phenomena: by measuring both the frequency (f) and the wavelength () of the propagating wave, one can derive the speed of the wave from the simple wave relation, speed = f×. (The frequency of a wave is the number of crests that pass per second, whereas the wavelength is the distance between crests). Although the two phenomena share these measurement approaches, the fundamental differences between light and sound have led to very different experimental implementations, as well as different historical developments, in the determination of their speeds.
In its simplest form, sound can be thought of as a longitudinal wave consisting of compressions and extensions of a medium along the direction of propagation. Because sound requires a medium through which to propagate, the speed of a sound wave is determined by the properties of the medium itself (such as density, stiffness, and temperature). These parameters thus need to be included in any reported measurements. In fact, one can turn such measurements around and actually use them to determine thermodynamic properties of the medium (the ratio of specific heats, for example).
On supporting science journalism
If you're enjoying this article, consider supporting our award-winning journalism by subscribing . By purchasing a subscription you are helping to ensure the future of impactful stories about the discoveries and ideas shaping our world today.
The first known theoretical treatise on sound was provided by Sir Isaac Newton in his Principia, which predicted a value for the speed of sound in air that differs by about 16 percent from the currently accepted value. Early experimental values were based on measurements of the time it took the sound of cannon blasts to cover a given distance and were good to better than 1 percent of the currently accepted value of 331.5 m/s at 0 degrees Celsius. Daniel Colladon and Charles-Francois Sturm first performed similar measurements in water in Lake Geneva in 1826. They found a value only 0.2 percent below the currently accepted value of ~1,440 m/s at 8 degrees C. These measurements all suffered from variations in the media themselves over long distances, so most subsequent determinations have been performed in the laboratory, where environmental parameters could be better controlled, and a larger variety of gases and liquids could be investigated. These experiments often use tubes of gas or liquid (or bars of solid material) with precisely calibrated lengths. One can then derive the speed of sound from a measurement of the time that an impulse of sound takes to traverse the tube. Alternatively (and usually more accurately), one can excite resonant frequencies of the tube (much like those of a flute) by inducing a vibration at one end with a loudspeaker, tuning fork, or other type of transducer. Because the corresponding resonant wavelengths have a simple relationship to the tube length, one can then determine the speed of sound from the wave relation and make corrections for tube geometry for comparisons with speeds in free space.
The wave nature of light is quite different from that of sound. In its simplest form, an electromagnetic wave (such as light, radio, or microwave) is transverse, consisting of oscillating electric and magnetic fields that are perpendicular to the direction of propagation. Moreover, although the medium through which light travels does affect its speed (reducing it by the index of refraction of the material), light can also travel through a vacuum, thus providing a unique context for defining its speed. In fact, the speed of light in a vacuum, c, is a fundamental building block of Einstein's theory of relativity, because it sets the upper limit for speeds in the universe. As a result, it appears in a wide range of physical formulae, perhaps the most famous of which is E=mc 2 . The speed of light can thus be measured in a variety of ways, but due to its extremely high value (~300,000 km/s or 186,000 mi/s), it was initially considerably harder to measure than the speed of sound. Early efforts such as Galileo's pair of observers sitting on opposing hills flashing lanterns back and forth lacked the technology needed to measure accurately the transit times of only a few microseconds. Remarkably, astronomical observations in the 18th century led to a determination of the speed of light with an uncertainty of only 1 percent. Better measurements, however, required a laboratory environment. Louis Fizeau and Leon Foucault were able to perform updated versions of Galileo¿s experiment through the use of ingenious combinations of rotating mirrors (along with improved measurement technology) and they made a series of beautiful measurements of the speed of light. With still further improvements, Albert A. Michelson performed measurements good to nearly one part in ten thousand.
Metrology of the speed of light changed dramatically with a determination made here at NIST in 1972. This measurement was based on a helium-neon laser whose frequency was fixed by a feedback loop to match the frequency corresponding to the splitting between two quantized energy levels of the methane molecule. Both the frequency and wavelength of this highly stable laser were accurately measured, thereby leading to a 100-times reduction in the uncertainty for the value of the speed of light. This measurement and subsequent measurements based on other atomic/molecular standards were limited not by the measurement technique, but by uncertainties in the definition of the meter itself. Because it was clear that future measurements would be similarly limited, the 17th Conf¿rence G¿n¿rale des Poids et Mesures (General Conference on Weights and Measures) decided in 1983 to redefine the meter in terms of the speed of light. The speed of light thus became a constant (defined to be 299,792,458 m/s), never to be measured again. As a result, the definition of the meter is directly linked (via the relation c= f×) to that of frequency, which is by far the most accurately measured physical quantity (presently the best cesium atomic fountain clocks have a fractional frequency uncertainty of about 1x10 -15 ).

IMAGES
COMMENTS
Method. Apparatus setup to measure the speed of sound in a column of air. Place the tube inside the beaker, so the water comes up a quarter of the way. The side of the tube in the water acts as a closed-end. When resonance (loudest sound) is heard, mark the water level with a rubber band or marker pen. Record this as L1.
Measuring the speed of sound. In this investigation, students measure distance and time in order to calculate the speed of a sound wave. The investigation supports the science capability 'Gather and interpret data'. It also provides a real-world context in which to practise mathematical skills. By the end of this investigation, students ...
The speed of sound in air is approximately 1,130 feet (344 meters) per second at room temperature, though this speed can vary with changes in temperature and humidity. You can perform a simple experiment involving two blocks of wood, a stopwatch and a tape measure to measure the speed of sound in air by calculating the time it takes for the ...
Calculate the velocity of sound at this height using the following equation: v = 4f (H + 0.4D) v is the velocity of sound. f is the frequency of the tuning fork in Hz. H is the height distance between the top of the water and the lip of the cylinder. D is the diameter of the water container in meters.
Measuring the speed of sound directly between two points. Use the trundle wheel to measure a distance of 100 m between two people. One of the people should have two wooden blocks, which they will bang together above their head to generate sound waves. The second person should have a stopwatch which they start when they see the first person ...
Although sound travels quite fast, it is still possible to measure its speed in air. To do this, you need to measure the time it takes a sound to travel a measured distance. To reduce errors ...
A sound wave is a mechanical vibration that propagates through a medium, such as air or a liquid. The speed of sound is the speed at which this wave propagates in this m edium, it depends on the temperature, the pressure and the density of the medium through which it propagates. In air, if we assimilate it to a perfect diatomic gas, we can calculate the speed of sound by the equation: c = sqrt ...
6. To determine , you must find at least one other resonance location in order to calculate /2. Find two more. 7. Calculate avg. 8. Calculate the speed of sound using avg for this tuning fork. 9. Repeat the above procedure for the other tuning fork. 10. Calculate an average value for the speed of sound. 11. Calculate a theoretical value for the
This video shows how we can measure the speed of sound in air. In order to calculate the speed of a wave, we need to measure the distance covered by a wave i...
The speed of a sound wave refers to how fast a sound wave is passed from particle to particle through a medium. The speed of a sound wave in air depends upon the properties of the air - primarily the temperature. Sound travels faster in solids than it does in liquids; sound travels slowest in gases such as air. The speed of sound can be calculated as the distance-per-time ratio or as the ...
With two smartphones you can easily determine the speed of sound.Get phyphox for free on Android and iOS: http://phyphox.orgSupport us: https://phyphox.org/d...
The speed of sound in dry air at room temperature is 343 m/s or 1125 ft/s. In physics, the speed of sound is the distance traveled per unit of time by a sound wave through a medium. It is highest for stiff solids and lowest for gases. There is no sound or speed of sound in a vacuum because sound (unlike light) requires a medium in order to ...
This video will outline how to perform an experiment to measure the speed of sound using standing waves.
Measuring the speed of sound. This is a simulation of a standard physics demonstration to measure the speed of sound in air. A vibrating tuning fork is held above a tube - the tube has some water in it, and the level of the water in the tube can be adjusted. This gives a column of air in the tube, between the top of the water and the top of the ...
Abstract. This paper demonstrates a variation on the classic Kundt's tube experiment for measuring the speed of sound. The speed of sound in air is measured using a smartphone and a cardboard tube, making the experiment very economical in terms of equipment. The speed of sound in air is measured to within 3% of the theoretical prediction.
When you make a sound by snapping your fingers next to the opening, the computer will begin collecting data. After the sound reflects off the opposite end of the tube, a graph will be displayed showing the initial sound and the echo. You will then be able to determine the round trip time and calculate the speed of sound.
Measuring the speed of sound using an oscilloscope. Connect two microphones to an oscilloscope. Place them about 2 m apart using a tape measure to measure the distance between them. Set up the oscilloscope so that it triggers when the first microphone detects a sound, and adjust the time base so that the sound arriving at both microphones can ...
Fix the reservoir R in the uppermost position. Loosen the pinch cock P and fill the reservoir and metallic tube completely with water by a beaker. Tighten the pinch cock, lower the reservoir and fix it in the lowest position. Take a tuning fork of higher frequency.
The speed of sound is measured in the following units: Speed of sound in Km per hour - 1,235 Kmph. Speed of Sound in miles per hour - 767 mph. Speed of sound in air feet per second - 1,125 ft s⁻¹. The velocity of sound is measured in hydrogen and oxygen (H2 and O2) is always 332 m/s. Before starting with the experiment to measure the speed ...
Students will first predict the speed of sound in the air of their classroom using a simple relationship accounting for temperature. Students will measure the time it takes a short pulse of sound to travel the length of a tube, reflect off the closed end, and return. Using this measurement, they will calculate the speed of sound and compare it to their prediction.
The speed of sound is the distance travelled per unit of time by a sound wave as it propagates through an elastic medium. More simply, the speed of sound is how fast vibrations travel. At 20 °C (68 °F), the speed of sound in air is about 343 m/s (1,125 ft/s; 1,235 km/h; 767 mph; 667 kn), or 1 km in 2.91 s or one mile in 4.69 s.It depends strongly on temperature as well as the medium through ...
The first method is based on simply measuring the time it takes a pulse of light or sound to traverse a known distance; dividing the distance by the transit time then gives the speed. The second ...