- Privacy Policy

Home » Applied Research – Types, Methods and Examples

Applied Research – Types, Methods and Examples
Table of Contents

Applied Research
Definition:
Applied research is a type of scientific inquiry that focuses on developing practical solutions to real-world problems. It involves the use of existing knowledge, theories, and techniques to address specific problems or challenges in a particular field or industry.
Applied research is often conducted in collaboration with industry or government partners, who provide funding and expertise to support the research. The results of applied research are typically intended to be directly applicable to the real world, and may involve the development of new products, technologies, or processes.
Types of Applied Research
Types of Applied Research are as follows:
Action Research
This type of research is designed to solve specific problems within an organization or community. The research involves collaboration between researchers and stakeholders to develop solutions to issues that affect the organization or community.
Evaluation Research
This type of research is used to assess the effectiveness of a particular program, policy, or intervention. Evaluation research is often used in government, healthcare, and social service settings to determine whether programs are meeting their intended goals.
Developmental Research
This type of research is used to develop new products, technologies, or processes. The research may involve the testing of prototypes or the development of new methods for production or delivery.
Diagnostic Research
This type of research is used to identify the causes of problems or issues. Diagnostic research is often used in healthcare, where researchers may investigate the causes of a particular disease or condition.
Policy Research
This type of research is used to inform policy decisions. Policy research may involve analyzing the impact of existing policies or evaluating the potential outcomes of proposed policies.
Predictive Research
This type of research is used to forecast future trends or events. Predictive research is often used in marketing, where researchers may use data analysis to predict consumer behavior or market trends.
Data Collection Methods
In applied research, data collection methods can be broadly classified into two categories: Quantitative and Qualitative methods:
Quantitative Data Collection
Quantitative research methods involve collecting numerical data that can be analyzed statistically. The most commonly used quantitative data collection methods in applied research include:
- Surveys : Surveys are questionnaires designed to collect data from a large sample of people. Surveys can be conducted face-to-face, over the phone, or online.
- Experiments : Experiments involve manipulating variables to test cause-and-effect relationships. Experiments can be conducted in the lab or in the field.
- Observations : Observations involve watching and recording behaviors or events in a systematic way. Observations can be conducted in the lab or in natural settings.
- Secondary data analysis: Secondary data analysis involves analyzing data that has already been collected by someone else. This can include data from government agencies, research institutes, or other sources.
Qualitative Data Collection
Qualitative research methods involve collecting non-numerical data that can be analyzed for themes and patterns. The most commonly used qualitative data collection methods in applied research include:
- Interviews : Interviews involve asking open-ended questions to individuals or groups. Interviews can be conducted in-person, over the phone, or online.
- Focus groups : Focus groups involve a group of people discussing a topic with a moderator. Focus groups can be conducted in-person or online.
- Case studies : Case studies involve in-depth analysis of a single individual, group, or organization.
- Document analysis : Document analysis involves analyzing written or recorded documents to extract data. This can include analyzing written records, audio recordings, or video recordings.
Data Analysis Methods
In applied research, data analysis methods can be broadly classified into two categories: Quantitative and Qualitative methods:
Quantitative Data Analysis
Quantitative data analysis methods involve analyzing numerical data to identify patterns and trends. The most commonly used quantitative data analysis methods in applied research include:
- Descriptive statistics: Descriptive statistics involve summarizing and presenting data using measures such as mean, median, mode, and standard deviation.
- Inferential statistics : Inferential statistics involve testing hypotheses and making predictions about a population based on a sample of data. This includes methods such as t-tests, ANOVA, regression analysis, and correlation analysis.
- Data mining: Data mining involves analyzing large datasets to identify patterns and relationships using machine learning algorithms.
Qualitative Data Analysis
Qualitative data analysis methods involve analyzing non-numerical data to identify themes and patterns. The most commonly used qualitative data analysis methods in applied research include:
- Content analysis: Content analysis involves analyzing written or recorded data to identify themes and patterns. This includes methods such as thematic analysis, discourse analysis, and narrative analysis.
- Grounded theory: Grounded theory involves developing theories and hypotheses based on the analysis of data.
- Interpretative phenomenological analysis: Interpretative phenomenological analysis involves analyzing data to identify the subjective experiences of individuals.
- Case study analysis: Case study analysis involves analyzing a single individual, group, or organization in-depth to identify patterns and themes.
Applied Research Methodology
Applied research methodology refers to the set of procedures, tools, and techniques used to design, conduct, and analyze research studies aimed at solving practical problems in real-world settings. The general steps involved in applied research methodology include:
- Identifying the research problem: The first step in applied research is to identify the problem to be studied. This involves conducting a literature review to identify existing knowledge and gaps in the literature, and to determine the research question.
- Developing a research design : Once the research question has been identified, the next step is to develop a research design. This involves determining the appropriate research method (quantitative, qualitative, or mixed methods), selecting the data collection methods, and designing the sampling strategy.
- Collecting data: The third step in applied research is to collect data using the selected data collection methods. This can include surveys, interviews, experiments, observations, or a combination of methods.
- Analyzing data : Once the data has been collected, it needs to be analyzed using appropriate data analysis methods. This can include descriptive statistics, inferential statistics, content analysis, or other methods, depending on the type of data collected.
- Interpreting and reporting findings : The final step in applied research is to interpret the findings and report the results. This involves drawing conclusions from the data analysis and presenting the findings in a clear and concise manner.
Applications of Applied Research
Some applications of applied research are as follows:
- Product development: Applied research can help companies develop new products or improve existing ones. For example, a company might conduct research to develop a new type of battery that lasts longer or a new type of software that is more efficient.
- Medical research : Applied research can be used to develop new treatments or drugs for diseases. For example, a pharmaceutical company might conduct research to develop a new cancer treatment.
- Environmental research : Applied research can be used to study and address environmental problems such as pollution and climate change. For example, research might be conducted to develop new technologies for reducing greenhouse gas emissions.
- Agriculture : Applied research can be used to improve crop yields, develop new varieties of plants, and study the impact of pests and diseases on crops.
- Education : Applied research can be used to study the effectiveness of teaching methods or to develop new teaching strategies.
- Transportation : Applied research can be used to develop new technologies for transportation, such as electric cars or high-speed trains.
- Communication : Applied research can be used to improve communication technologies, such as developing new methods for wireless communication or improving the quality of video calls.
Examples of Applied Research
Here are some real-time examples of applied research:
- COVID-19 Vaccine Development: The development of COVID-19 vaccines is a prime example of applied research. Researchers applied their knowledge of virology and immunology to develop vaccines that could prevent or reduce the severity of COVID-19.
- Autonomous Vehicles : The development of autonomous vehicles involves applied research in areas such as artificial intelligence, computer vision, and robotics. Companies like Tesla, Waymo, and Uber are conducting extensive research to improve their autonomous vehicle technology.
- Renewable Energy : Research is being conducted on renewable energy sources like solar, wind, and hydro power to improve efficiency and reduce costs. This is an example of applied research that aims to solve environmental problems.
- Precision Agriculture : Applied research is being conducted in the field of precision agriculture, which involves using technology to optimize crop yields and reduce waste. This includes research on crop sensors, drones, and data analysis.
- Telemedicine : Telemedicine involves using technology to deliver healthcare remotely. Applied research is being conducted to improve the quality of telemedicine services, such as developing new technologies for remote diagnosis and treatment.
- Cybersecurity : Applied research is being conducted to improve cybersecurity measures and protect against cyber threats. This includes research on encryption, network security, and data protection.
Purpose of Applied Research
The purpose of applied research is to solve practical problems or improve existing products, technologies, or processes. Applied research is focused on specific goals and objectives and is designed to have direct practical applications in the real world. It seeks to address problems and challenges faced by individuals, organizations, or communities and aims to provide solutions that can be implemented in a practical manner.
The primary purpose of applied research is to generate new knowledge that can be used to solve real-world problems or improve the efficiency and effectiveness of existing products, technologies, or processes. Applied research is often conducted in collaboration with industry, government, or non-profit organizations to address practical problems and create innovative solutions.
Applied research is also used to inform policy decisions by providing evidence-based insights into the effectiveness of specific interventions or programs. By conducting research on the impact of policies and programs, decision-makers can make informed decisions about how to allocate resources and prioritize interventions.
Overall, the purpose of applied research is to improve people’s lives by developing practical solutions to real-world problems. It aims to bridge the gap between theory and practice, and to ensure that research findings are put into action to achieve tangible benefits.
When to use Applied Research
Here are some specific situations when applied research may be appropriate:
- When there is a need to develop a new product : Applied research can be used to develop new products that meet the needs of consumers. For example, a company may conduct research to develop a new type of smartphone with improved features.
- When there is a need to improve an existing product : Applied research can also be used to improve existing products. For example, a company may conduct research to improve the battery life of an existing product.
- When there is a need to solve a practical problem: Applied research can be used to solve practical problems faced by individuals, organizations, or communities. For example, research may be conducted to find solutions to problems related to healthcare, transportation, or environmental issues.
- When there is a need to inform policy decisions: Applied research can be used to inform policy decisions by providing evidence-based insights into the effectiveness of specific interventions or programs.
- When there is a need to improve efficiency and effectiveness: Applied research can be used to improve the efficiency and effectiveness of processes or systems. For example, research may be conducted to identify ways to streamline manufacturing processes or to improve the delivery of healthcare services.
Characteristics of Applied Research
The following are some of the characteristics of applied research:
- Focus on solving real-world problems : Applied research focuses on addressing specific problems or needs in a practical setting, with the aim of developing solutions that can be implemented in the real world.
- Goal-oriented: A pplied research is goal-oriented, with a specific aim of solving a particular problem or meeting a specific need. The research is usually designed to achieve a specific outcome, such as developing a new product, improving an existing process, or solving a particular issue.
- Practical and relevant: Applied research is practical and relevant to the needs of the industry or field in which it is conducted. It aims to provide practical solutions that can be implemented to improve processes or solve problems.
- Collaborative : Applied research often involves collaboration between researchers and practitioners, such as engineers, scientists, and business professionals. Collaboration allows for the exchange of knowledge and expertise, which can lead to more effective solutions.
- Data-driven: Applied research is data-driven, relying on empirical evidence to support its findings and recommendations. Data collection and analysis are important components of applied research, as they help to identify patterns and trends that can inform decision-making.
- Results-oriented: Applied research is results-oriented, with an emphasis on achieving measurable outcomes. Research findings are often used to inform decisions about product development, process improvement, or policy changes.
- Time-bound : Applied research is often conducted within a specific timeframe, with deadlines for achieving specific outcomes. This helps to ensure that the research stays focused on its goals and that the results are timely and relevant to the needs of the industry or field.
Advantages of Applied Research
Some of the advantages of applied research are as follows:
- Practical solutions: Applied research is focused on developing practical solutions to real-world problems, making it highly relevant to the needs of the industry or field in which it is conducted. The solutions developed through applied research are often highly effective and can be implemented quickly to address specific issues.
- Improved processes: Applied research can help organizations to improve their processes, leading to increased efficiency and productivity. The research can identify areas for improvement, such as bottlenecks or inefficiencies, and provide recommendations for optimizing processes.
- Innovation: Applied research can lead to the development of new products, services, and technologies that can transform industries and create new opportunities for growth and innovation. The research can help organizations to identify unmet needs and develop new solutions to meet them.
- Collaboration : Applied research often involves collaboration between researchers and practitioners, leading to the exchange of knowledge and expertise. Collaboration can result in more effective solutions and can help to build partnerships between academia and industry.
- Increased competitiveness : Applied research can help organizations to stay competitive by enabling them to adapt to changing market conditions and customer needs. The research can provide insights into emerging trends and technologies, helping organizations to stay ahead of the curve.
- Economic growth: Applied research can contribute to economic growth by creating new industries and jobs. The research can lead to the development of new technologies and products that can drive economic growth and create new opportunities for entrepreneurship and innovation.
Limitations of Applied Research
Some of the limitations of applied research are as follows:
- Limited generalizability: Applied research often focuses on specific contexts and may not be generalizable to other settings. This means that the findings of applied research may not be applicable to other industries, regions, or populations.
- Time and resource constraints: Applied research is often conducted within a specific timeframe and with limited resources. This can limit the scope and depth of the research and may prevent researchers from exploring all possible avenues.
- Potential for bias: Applied research may be influenced by the interests and perspectives of the organization or industry funding the research. This can lead to a bias in the research and potentially compromise the objectivity and validity of the findings.
- Ethical considerations: Applied research may raise ethical concerns, particularly if it involves human subjects or sensitive issues. Researchers must adhere to ethical standards and ensure that the research is conducted in a responsible and respectful manner.
- Limited theoretical development: Applied research tends to focus on practical solutions and may not contribute significantly to theoretical development in a particular field. This can limit the broader impact of the research and may hinder the development of new theories and frameworks.
- Limited focus on long-term impact: Applied research often focuses on short-term outcomes, such as developing a new product or improving a process. This may limit the focus on long-term impacts, such as the sustainability of the solution or its broader implications for the industry or society.
About the author
Muhammad Hassan
Researcher, Academic Writer, Web developer
You may also like

Questionnaire – Definition, Types, and Examples

Case Study – Methods, Examples and Guide

Observational Research – Methods and Guide

Quantitative Research – Methods, Types and...

Explanatory Research – Types, Methods, Guide

Survey Research – Types, Methods, Examples
- Skip to main content
- Skip to primary sidebar
- Skip to footer
- QuestionPro

- Solutions Industries Gaming Automotive Sports and events Education Government Travel & Hospitality Financial Services Healthcare Cannabis Technology Use Case NPS+ Communities Audience Contactless surveys Mobile LivePolls Member Experience GDPR Positive People Science 360 Feedback Surveys
- Resources Blog eBooks Survey Templates Case Studies Training Help center

Home Market Research Research Tools and Apps
Applied Research: Definition, Types & Examples
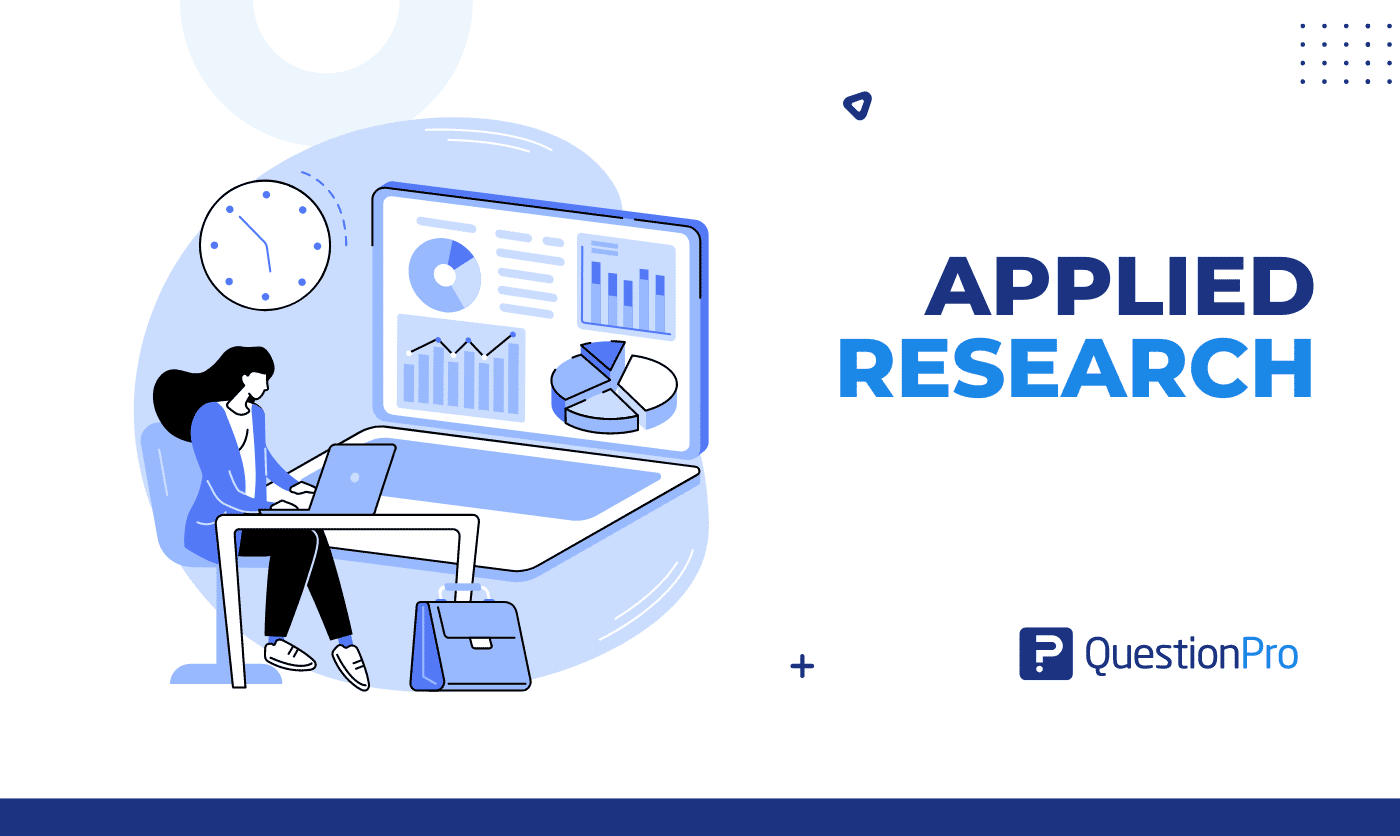
Every research project begins with a clear definition of the investigation’s purpose, which helps to identify the research procedure or approach used. In this sense, a researcher can conduct either basic or applied research.
This research focuses on answering specific questions to solve a specific problem. It tries to identify a solution to a cultural or organizational problem and is often a follow-up research plan for basic or pure research.
In this blog, we will explain the types of applied research and give some examples. But before that, we will go through what it is.
What is applied research?
Applied research is a non-systematic way of finding solutions to specific research problems or issues. These problems or issues can be on an individual, group, or societal level. It is called “non-systematic” because it goes straight to finding solutions.
It is often called a “scientific process” because it uses the available scientific tools and puts them to use to find answers.
Like in regular research, the researcher identifies the problem, makes a hypothesis, and then experiments to test it. It goes deeper into the findings of true or basic research.
LEARN ABOUT: Research Process Steps
Types of applied research
This research has three types:
- Evaluation research,
- Research and Development, and
- Action research.
The short versions of each type are explained below:
- Evaluation research
Evaluation research is one type of applied research. It looks at the information on a research subject. This kind of research leads to objective research or helps people make better decisions sooner. Most of the time, evaluation research is used in business settings.
The organization uses this research to figure out how the overhead costs can be cut down or cut down a lot.
- Research and development
Research and Development is the second type of applied research. Its main goal is to create or design new products, goods, or services that meet the needs of certain markets in society. It finds out what the needs of the market are. It focuses on finding new ways to improve products that already meet an organization’s needs.
- Action research
Action research is the third type of applied research. Action research is a way to learn about things that happen in everyday life and nature. Its goal is to find real-world solutions to business problems by pointing the business in the right direction.
LEARN ABOUT: Action Research
Examples of applied research
Applied study is used in many areas of study and research, from the sciences to the social sciences. We also talk about how it’s used in those fields and give some examples:
- Applied study in business
Applied study in business sectors is fully dependent on their products and services. It helps organizations understand market needs and trends, and then shape their products to fit customers.
Businesses benefit from This research because it allows them to detect gaps in their findings and obtain primary information on target market preferences.
- It can improve hiring.
- It improves work and policy.
- It identifies workplace skill gaps.
- Applied study in education
The applied study is used in the education field to test different ways of teaching and to find better ways of teaching and learning. Before implementing new education policies, they are tested to see how well they work, how they affect teaching, and how the classroom works.
Applied education research uses quantitative and qualitative methods to collect data from first-hand sources. This information is then looked at and interpreted differently to generate valuable results or conclusions.
LEARN ABOUT: Qualitative Interview
Most applied research in this field is done to develop and test different ways of doing things by trying them out in different situations. It is based on accurate observations and descriptions of the real world.
- Applied study to understand the reach of online learning initiatives.
- Applied study to promote teacher-student classroom engagement.
- Applied study on the new math program.
- Applied study in science
As already said, applied study is often called a scientific process because it uses the available scientific tools to find answers. It can be used in physics, microbiology, thermodynamics, and other fields.
- The applied study is put into practice to cure a disease.
- The applied study is put into practice to improve agricultural practices.
- The applied study is applied to testing new laboratory equipment.
- Applied study in psychology
Researchers use this research in psychology to figure out how people act at work, how HR works, and how the organization is growing and changing so they can come up with solutions.
It is used a lot in areas where researchers try to figure out how people think and then come up with solutions that fit their behavior best.
- Applied study to figure out new ways to deal with depression.
- Applied study to improve students’ grades by emphasizing practical Education.
- Applied study to create a plan to keep employees coming to work regularly.
- Applied study in health
This research is used to examine new drugs in the medical industry. It combines scientific knowledge and procedures with health experiences to produce evidence-based results.
- Applied study in heart surgery.
- Applied study to determine a drug’s efficacy.
- Applied study on a medicine’s adverse effects.
LEARN ABOUT: Theoretical Research
Applied research is an important way to research because it helps organizations find real-world solutions to specific problems while also increasing their output and productivity. In contrast to basic research, which focuses on making theories that explain things, applied research focuses on describing evidence to find solutions.
In the applied study, the researcher uses qualitative and quantitative methods to collect data, such as questionnaires, interviews, and observation methods. Conducting interviews is one of the examples of qualitative data in education . It helps the researcher collect real-world evidence, which is then tested depending on the type of applied research and the main focus.
At QuestionPro, we give researchers access to a library of long-term research insights and tools for collecting data, like our survey software. Go to InsightHub if you want to see a demo or learn more about it.
FREE TRIAL LEARN MORE
MORE LIKE THIS
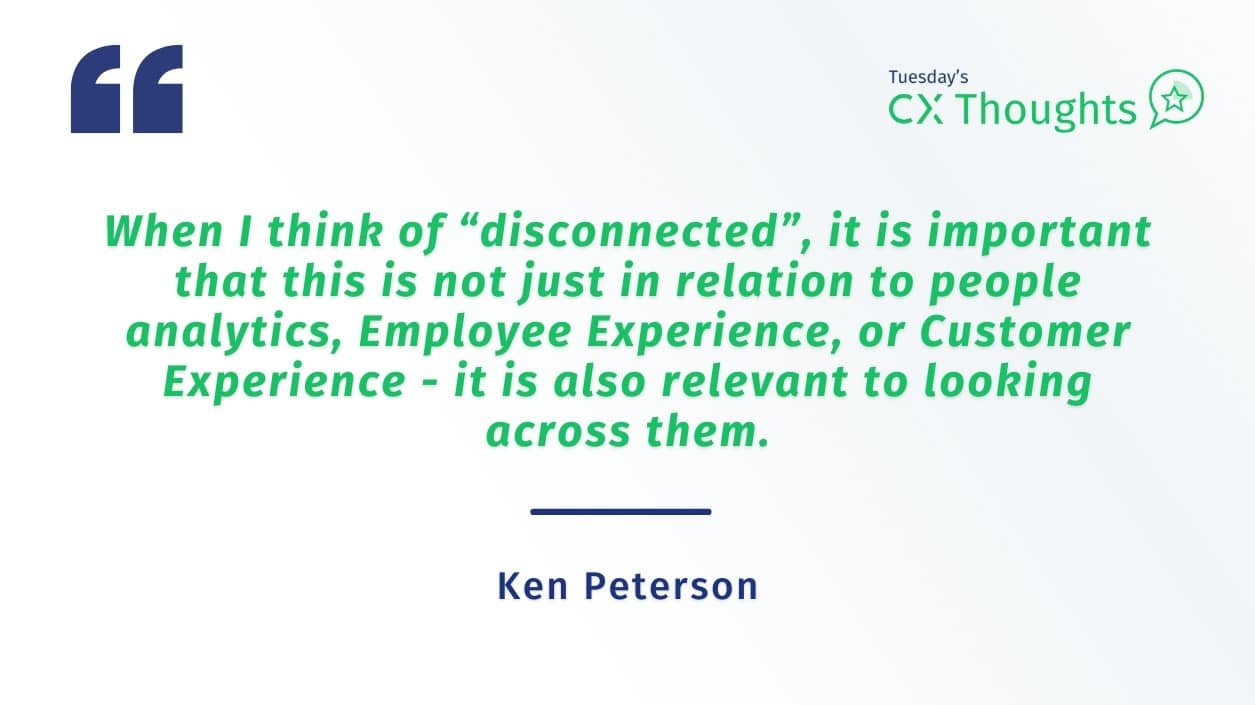
I Am Disconnected – Tuesday CX Thoughts
May 21, 2024
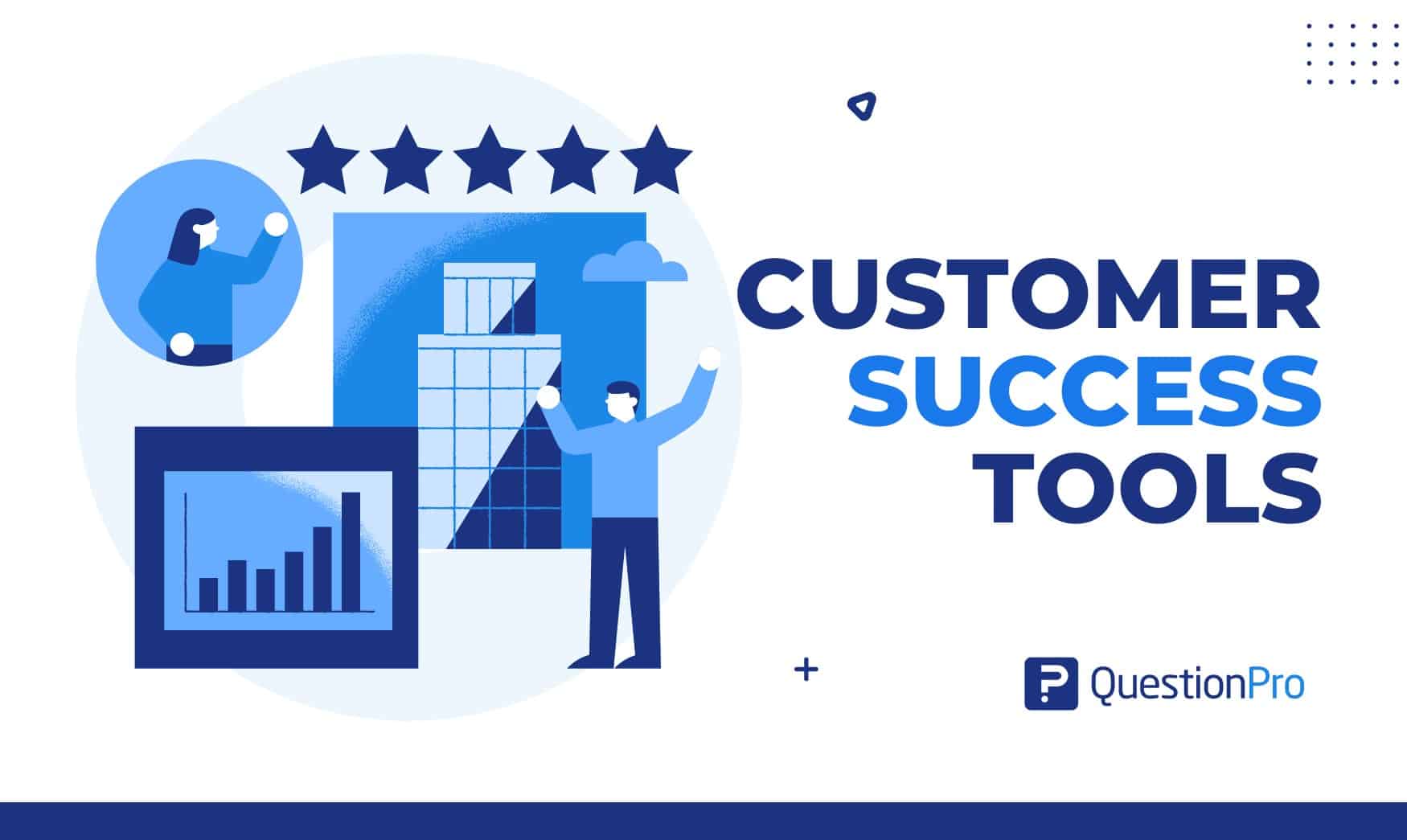
20 Best Customer Success Tools of 2024
May 20, 2024
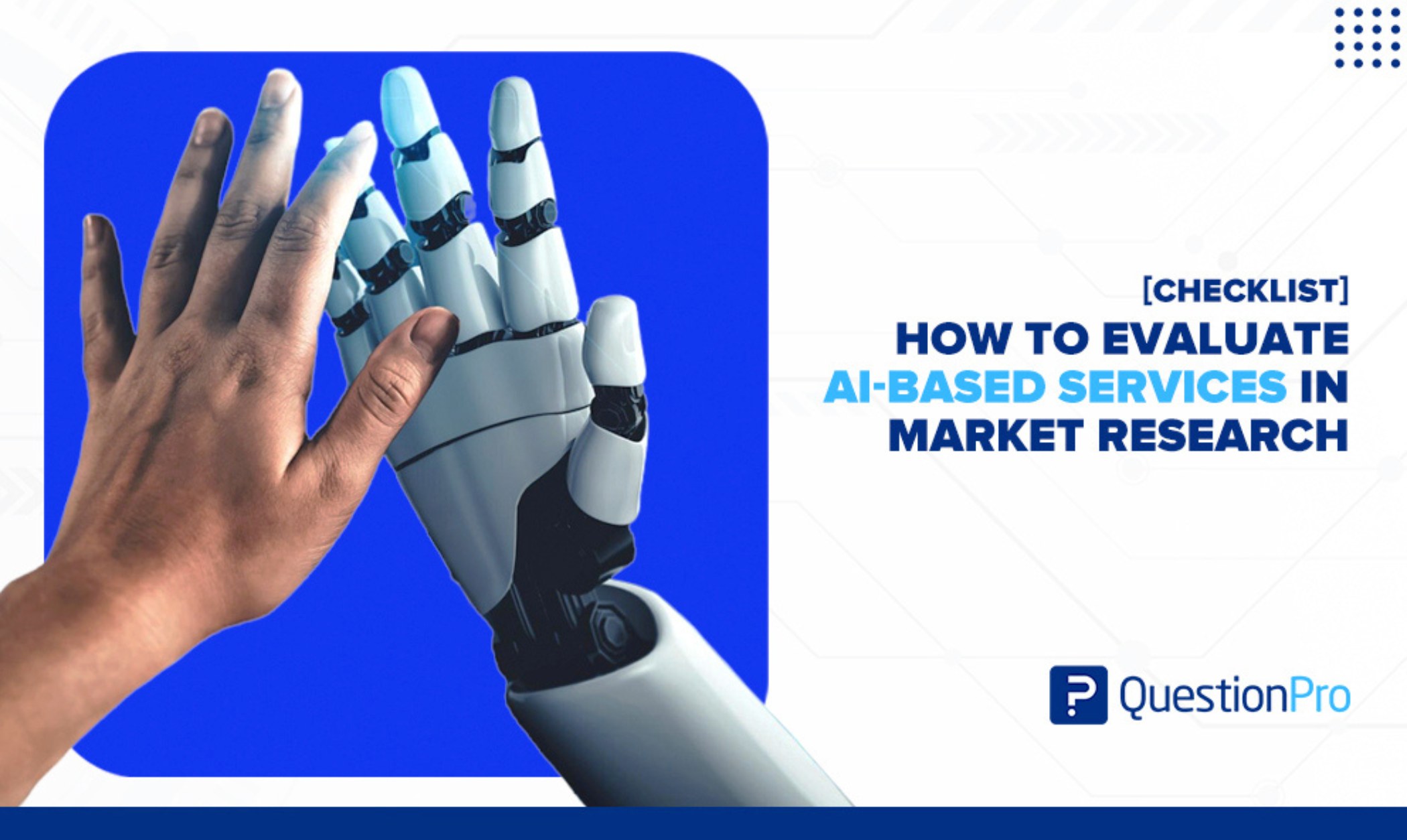
AI-Based Services Buying Guide for Market Research (based on ESOMAR’s 20 Questions)
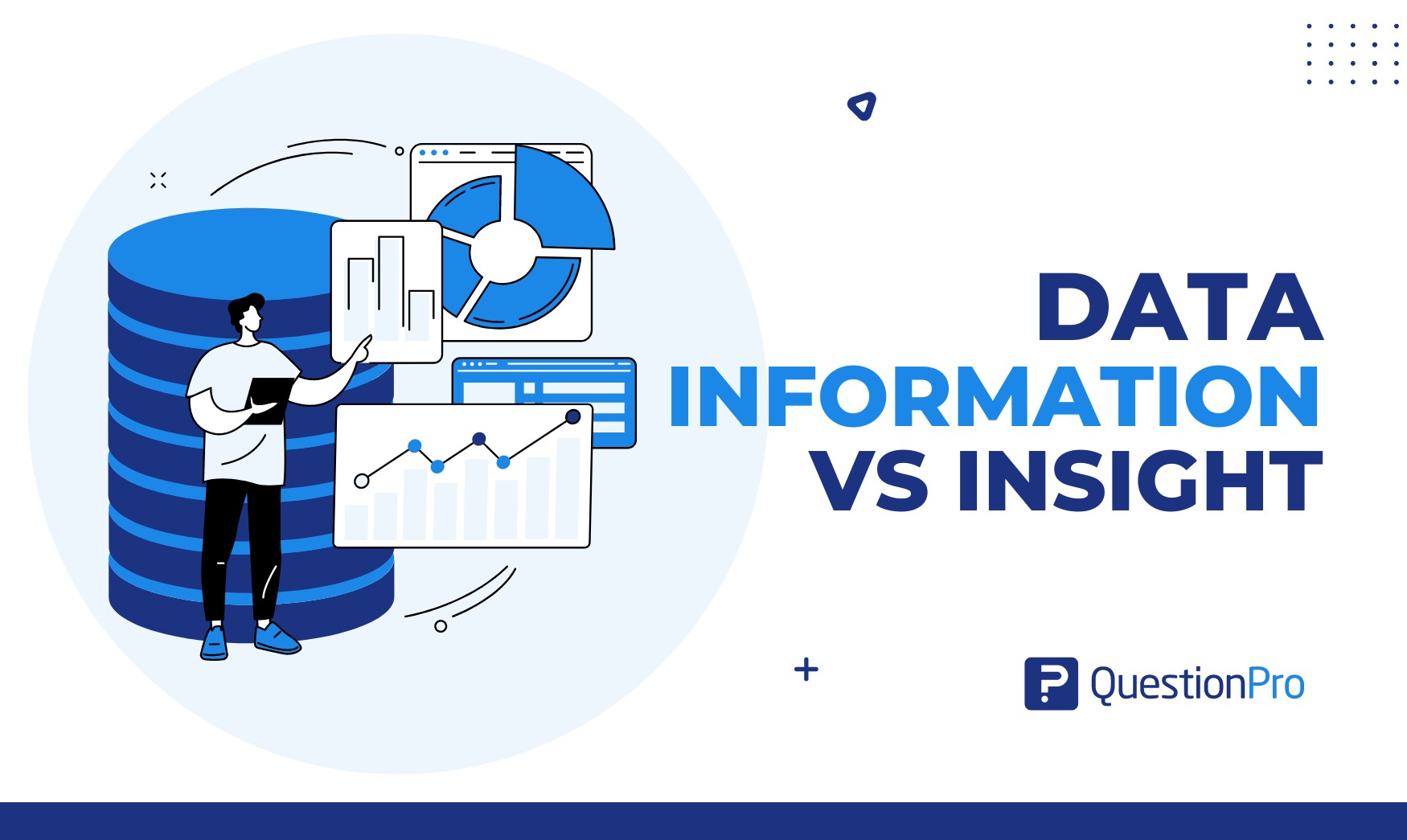
Data Information vs Insight: Essential differences
May 14, 2024
Other categories
- Academic Research
- Artificial Intelligence
- Assessments
- Brand Awareness
- Case Studies
- Communities
- Consumer Insights
- Customer effort score
- Customer Engagement
- Customer Experience
- Customer Loyalty
- Customer Research
- Customer Satisfaction
- Employee Benefits
- Employee Engagement
- Employee Retention
- Friday Five
- General Data Protection Regulation
- Insights Hub
- Life@QuestionPro
- Market Research
- Mobile diaries
- Mobile Surveys
- New Features
- Online Communities
- Question Types
- Questionnaire
- QuestionPro Products
- Release Notes
- Research Tools and Apps
- Revenue at Risk
- Survey Templates
- Training Tips
- Uncategorized
- Video Learning Series
- What’s Coming Up
- Workforce Intelligence
- Bipolar Disorder
- Therapy Center
- When To See a Therapist
- Types of Therapy
- Best Online Therapy
- Best Couples Therapy
- Best Family Therapy
- Managing Stress
- Sleep and Dreaming
- Understanding Emotions
- Self-Improvement
- Healthy Relationships
- Student Resources
- Personality Types
- Guided Meditations
- Verywell Mind Insights
- 2024 Verywell Mind 25
- Mental Health in the Classroom
- Editorial Process
- Meet Our Review Board
- Crisis Support
How Applied Research Is Used in Psychology
Kendra Cherry, MS, is a psychosocial rehabilitation specialist, psychology educator, and author of the "Everything Psychology Book."
:max_bytes(150000):strip_icc():format(webp)/IMG_9791-89504ab694d54b66bbd72cb84ffb860e.jpg)
Sean is a fact-checker and researcher with experience in sociology, field research, and data analytics.
:max_bytes(150000):strip_icc():format(webp)/Sean-Blackburn-1000-a8b2229366944421bc4b2f2ba26a1003.jpg)
Verywell / JR Bee
Basic vs. Applied Research
How it works, potential challenges.
- Real-World Applications
Applied research refers to scientific study and research that seeks to solve practical problems. This type of research plays an important role in solving everyday problems that can have an impact on life, work, health, and overall well-being. For example, it can be used to find solutions to everyday problems, cure illness, and develop innovative technologies.
There are many different types of psychologists who perform applied research. Human factors or industrial/organizational psychologists often do this type of research.
A few examples of applied research in psychology include:
- Analyzing what type of prompts will inspire people to volunteer their time to charities
- Investigating if background music in a work environment can contribute to greater productivity
- Investigating which treatment approach is the most effective for reducing anxiety
- Researching which strategies work best to motivate workers
- Studying different keyboard designs to determine which is the most efficient and ergonomic
As you may notice, all of these examples explore topics that will address real-world issues. This immediate and practical application of the findings is what distinguishes applied research from basic research , which instead focuses on theoretical concerns.
Basic research tends to focus on "big picture" topics, such as increasing the scientific knowledge base around a particular topic. Applied research tends to work toward solving specific problems that affect people in the here and now.
For example a social psychologist may perform basic research on how different factors may contribute to violence in general. But if a social psychologist were conducting applied research, they may be tackling the question of what specific programs can be implemented to reduce violence in school settings.
However, basic research and applied research are actually closely intertwined. The information learned from basic research often builds the basis on which applied research is formed.
Basic research often informs applied research, and applied research often helps basic researchers refine their theories.
Applied research usually starts by identifying a problem that exists in the real world. Then psychologists begin to conduct research in order to identify a solution.
The type of research used depends on a variety of factors. This includes unique characteristics of the situation and the kind of problem psychologists are looking to solve.
Researchers might opt to use naturalistic observation to see the problem as it occurs in a real-world setting. They may then conduct experiments to determine why the problem occurs and to explore different solutions that may solve it.
As with any type of research, challenges can arise when conducting applied research in psychology. Some potential problems that researchers may face include:
Ethical Challenges
When conducting applied research in a naturalistic setting, researchers have to avoid ethical issues, which can make research more difficult. For example, they may come across concerns about privacy and informed consent.
In some cases, such as in workplace studies conducted by industrial-organizational psychologists, participants may feel pressured or even coerced into participating as a condition of their employment. Such factors sometimes impact the result of research studies.
Problems With Validity
Since applied research often takes place in the field, it can be difficult for researchers to maintain complete control over all of the variables . Extraneous variables can also exert a subtle influence that experimenters may not even consider could have an effect on the results.
In many cases, researchers are forced to strike a balance between a study's ecological validity (which is usually quite high in applied research) and the study's internal validity .
Since applied research focuses on taking the results of scientific research and applying it to real-world situations, those who work in this line of research tend to be more concerned with the external validity of their work.
External validity refers to the extent that scientific findings can be generalized to other populations.
Researchers don't just want to know if the results of their experiments apply to the participants in their studies, rather they want these results to also apply to larger populations outside of the lab.
External validity is often of particular importance in applied research. Researchers want to know that their findings can be applied to real people in real settings.
How It's Used in the Real-World
Here are some examples of how applied research is used to solve real-world problems:
- A hospital may conduct applied research to figure out how to best prepare patients for certain types of surgical procedures.
- A business may hire an applied psychologist to assess how to design a workplace console to maximize efficiency and productivity while minimizing worker fatigue and error.
- An organization may hire an applied researcher to determine how to select employees that are best suited for certain positions within the company.
Applied research is an important tool in the process of understanding the human mind and behavior. Thanks to much of this research, psychologists are able to investigate problems that affect people's daily lives. This kind of research specifically targets real-world issues, however it also contributes to knowledge about how people think and behave.
National Science Foundation. Definitions of research and development: An annotated compilation of official sources .
CDC. Evaluation briefs .
Helmchen H. Ethical issues in naturalistic versus controlled trials . Dialogues Clin Neurosci . 2011;13(2):173‐182.
Truijens FL, Cornelis S, Desmet M, De Smet MM, Meganck R. Validity beyond measurement: Why psychometric validity is insufficient for valid psychotherapy research . Front Psychol . 2019;10:532. doi:10.3389/fpsyg.2019.00532
McBride D. The Process Of Research In Psychology . SAGE Publications; 2018.
By Kendra Cherry, MSEd Kendra Cherry, MS, is a psychosocial rehabilitation specialist, psychology educator, and author of the "Everything Psychology Book."

An official website of the United States government
The .gov means it’s official. Federal government websites often end in .gov or .mil. Before sharing sensitive information, make sure you’re on a federal government site.
The site is secure. The https:// ensures that you are connecting to the official website and that any information you provide is encrypted and transmitted securely.
- Publications
- Account settings
Preview improvements coming to the PMC website in October 2024. Learn More or Try it out now .
- Advanced Search
- Journal List
- Turk J Anaesthesiol Reanim
- v.44(4); 2016 Aug

What is Scientific Research and How Can it be Done?
Scientific researches are studies that should be systematically planned before performing them. In this review, classification and description of scientific studies, planning stage randomisation and bias are explained.
Research conducted for the purpose of contributing towards science by the systematic collection, interpretation and evaluation of data and that, too, in a planned manner is called scientific research: a researcher is the one who conducts this research. The results obtained from a small group through scientific studies are socialised, and new information is revealed with respect to diagnosis, treatment and reliability of applications. The purpose of this review is to provide information about the definition, classification and methodology of scientific research.
Before beginning the scientific research, the researcher should determine the subject, do planning and specify the methodology. In the Declaration of Helsinki, it is stated that ‘the primary purpose of medical researches on volunteers is to understand the reasons, development and effects of diseases and develop protective, diagnostic and therapeutic interventions (method, operation and therapies). Even the best proven interventions should be evaluated continuously by investigations with regard to reliability, effectiveness, efficiency, accessibility and quality’ ( 1 ).
The questions, methods of response to questions and difficulties in scientific research may vary, but the design and structure are generally the same ( 2 ).
Classification of Scientific Research
Scientific research can be classified in several ways. Classification can be made according to the data collection techniques based on causality, relationship with time and the medium through which they are applied.
- Observational
- Experimental
- Descriptive
- Retrospective
- Prospective
- Cross-sectional
- Social descriptive research ( 3 )
Another method is to classify the research according to its descriptive or analytical features. This review is written according to this classification method.
I. Descriptive research
- Case series
- Surveillance studies
II. Analytical research
- Observational studies: cohort, case control and cross- sectional research
- Interventional research: quasi-experimental and clinical research
- Case Report: it is the most common type of descriptive study. It is the examination of a single case having a different quality in the society, e.g. conducting general anaesthesia in a pregnant patient with mucopolysaccharidosis.
- Case Series: it is the description of repetitive cases having common features. For instance; case series involving interscapular pain related to neuraxial labour analgesia. Interestingly, malignant hyperthermia cases are not accepted as case series since they are rarely seen during historical development.
- Surveillance Studies: these are the results obtained from the databases that follow and record a health problem for a certain time, e.g. the surveillance of cross-infections during anaesthesia in the intensive care unit.
Moreover, some studies may be experimental. After the researcher intervenes, the researcher waits for the result, observes and obtains data. Experimental studies are, more often, in the form of clinical trials or laboratory animal trials ( 2 ).
Analytical observational research can be classified as cohort, case-control and cross-sectional studies.
Firstly, the participants are controlled with regard to the disease under investigation. Patients are excluded from the study. Healthy participants are evaluated with regard to the exposure to the effect. Then, the group (cohort) is followed-up for a sufficient period of time with respect to the occurrence of disease, and the progress of disease is studied. The risk of the healthy participants getting sick is considered an incident. In cohort studies, the risk of disease between the groups exposed and not exposed to the effect is calculated and rated. This rate is called relative risk. Relative risk indicates the strength of exposure to the effect on the disease.
Cohort research may be observational and experimental. The follow-up of patients prospectively is called a prospective cohort study . The results are obtained after the research starts. The researcher’s following-up of cohort subjects from a certain point towards the past is called a retrospective cohort study . Prospective cohort studies are more valuable than retrospective cohort studies: this is because in the former, the researcher observes and records the data. The researcher plans the study before the research and determines what data will be used. On the other hand, in retrospective studies, the research is made on recorded data: no new data can be added.
In fact, retrospective and prospective studies are not observational. They determine the relationship between the date on which the researcher has begun the study and the disease development period. The most critical disadvantage of this type of research is that if the follow-up period is long, participants may leave the study at their own behest or due to physical conditions. Cohort studies that begin after exposure and before disease development are called ambidirectional studies . Public healthcare studies generally fall within this group, e.g. lung cancer development in smokers.
- Case-Control Studies: these studies are retrospective cohort studies. They examine the cause and effect relationship from the effect to the cause. The detection or determination of data depends on the information recorded in the past. The researcher has no control over the data ( 2 ).
Cross-sectional studies are advantageous since they can be concluded relatively quickly. It may be difficult to obtain a reliable result from such studies for rare diseases ( 2 ).
Cross-sectional studies are characterised by timing. In such studies, the exposure and result are simultaneously evaluated. While cross-sectional studies are restrictedly used in studies involving anaesthesia (since the process of exposure is limited), they can be used in studies conducted in intensive care units.
- Quasi-Experimental Research: they are conducted in cases in which a quick result is requested and the participants or research areas cannot be randomised, e.g. giving hand-wash training and comparing the frequency of nosocomial infections before and after hand wash.
- Clinical Research: they are prospective studies carried out with a control group for the purpose of comparing the effect and value of an intervention in a clinical case. Clinical study and research have the same meaning. Drugs, invasive interventions, medical devices and operations, diets, physical therapy and diagnostic tools are relevant in this context ( 6 ).
Clinical studies are conducted by a responsible researcher, generally a physician. In the research team, there may be other healthcare staff besides physicians. Clinical studies may be financed by healthcare institutes, drug companies, academic medical centres, volunteer groups, physicians, healthcare service providers and other individuals. They may be conducted in several places including hospitals, universities, physicians’ offices and community clinics based on the researcher’s requirements. The participants are made aware of the duration of the study before their inclusion. Clinical studies should include the evaluation of recommendations (drug, device and surgical) for the treatment of a disease, syndrome or a comparison of one or more applications; finding different ways for recognition of a disease or case and prevention of their recurrence ( 7 ).
Clinical Research
In this review, clinical research is explained in more detail since it is the most valuable study in scientific research.
Clinical research starts with forming a hypothesis. A hypothesis can be defined as a claim put forward about the value of a population parameter based on sampling. There are two types of hypotheses in statistics.
- H 0 hypothesis is called a control or null hypothesis. It is the hypothesis put forward in research, which implies that there is no difference between the groups under consideration. If this hypothesis is rejected at the end of the study, it indicates that a difference exists between the two treatments under consideration.
- H 1 hypothesis is called an alternative hypothesis. It is hypothesised against a null hypothesis, which implies that a difference exists between the groups under consideration. For example, consider the following hypothesis: drug A has an analgesic effect. Control or null hypothesis (H 0 ): there is no difference between drug A and placebo with regard to the analgesic effect. The alternative hypothesis (H 1 ) is applicable if a difference exists between drug A and placebo with regard to the analgesic effect.
The planning phase comes after the determination of a hypothesis. A clinical research plan is called a protocol . In a protocol, the reasons for research, number and qualities of participants, tests to be applied, study duration and what information to be gathered from the participants should be found and conformity criteria should be developed.
The selection of participant groups to be included in the study is important. Inclusion and exclusion criteria of the study for the participants should be determined. Inclusion criteria should be defined in the form of demographic characteristics (age, gender, etc.) of the participant group and the exclusion criteria as the diseases that may influence the study, age ranges, cases involving pregnancy and lactation, continuously used drugs and participants’ cooperation.
The next stage is methodology. Methodology can be grouped under subheadings, namely, the calculation of number of subjects, blinding (masking), randomisation, selection of operation to be applied, use of placebo and criteria for stopping and changing the treatment.
I. Calculation of the Number of Subjects
The entire source from which the data are obtained is called a universe or population . A small group selected from a certain universe based on certain rules and which is accepted to highly represent the universe from which it is selected is called a sample and the characteristics of the population from which the data are collected are called variables. If data is collected from the entire population, such an instance is called a parameter . Conducting a study on the sample rather than the entire population is easier and less costly. Many factors influence the determination of the sample size. Firstly, the type of variable should be determined. Variables are classified as categorical (qualitative, non-numerical) or numerical (quantitative). Individuals in categorical variables are classified according to their characteristics. Categorical variables are indicated as nominal and ordinal (ordered). In nominal variables, the application of a category depends on the researcher’s preference. For instance, a female participant can be considered first and then the male participant, or vice versa. An ordinal (ordered) variable is ordered from small to large or vice versa (e.g. ordering obese patients based on their weights-from the lightest to the heaviest or vice versa). A categorical variable may have more than one characteristic: such variables are called binary or dichotomous (e.g. a participant may be both female and obese).
If the variable has numerical (quantitative) characteristics and these characteristics cannot be categorised, then it is called a numerical variable. Numerical variables are either discrete or continuous. For example, the number of operations with spinal anaesthesia represents a discrete variable. The haemoglobin value or height represents a continuous variable.
Statistical analyses that need to be employed depend on the type of variable. The determination of variables is necessary for selecting the statistical method as well as software in SPSS. While categorical variables are presented as numbers and percentages, numerical variables are represented using measures such as mean and standard deviation. It may be necessary to use mean in categorising some cases such as the following: even though the variable is categorical (qualitative, non-numerical) when Visual Analogue Scale (VAS) is used (since a numerical value is obtained), it is classified as a numerical variable: such variables are averaged.
Clinical research is carried out on the sample and generalised to the population. Accordingly, the number of samples should be correctly determined. Different sample size formulas are used on the basis of the statistical method to be used. When the sample size increases, error probability decreases. The sample size is calculated based on the primary hypothesis. The determination of a sample size before beginning the research specifies the power of the study. Power analysis enables the acquisition of realistic results in the research, and it is used for comparing two or more clinical research methods.
Because of the difference in the formulas used in calculating power analysis and number of samples for clinical research, it facilitates the use of computer programs for making calculations.
It is necessary to know certain parameters in order to calculate the number of samples by power analysis.
- Type-I (α) and type-II (β) error levels
- Difference between groups (d-difference) and effect size (ES)
- Distribution ratio of groups
- Direction of research hypothesis (H1)
a. Type-I (α) and Type-II (β) Error (β) Levels
Two types of errors can be made while accepting or rejecting H 0 hypothesis in a hypothesis test. Type-I error (α) level is the probability of finding a difference at the end of the research when there is no difference between the two applications. In other words, it is the rejection of the hypothesis when H 0 is actually correct and it is known as α error or p value. For instance, when the size is determined, type-I error level is accepted as 0.05 or 0.01.
Another error that can be made during a hypothesis test is a type-II error. It is the acceptance of a wrongly hypothesised H 0 hypothesis. In fact, it is the probability of failing to find a difference when there is a difference between the two applications. The power of a test is the ability of that test to find a difference that actually exists. Therefore, it is related to the type-II error level.
Since the type-II error risk is expressed as β, the power of the test is defined as 1–β. When a type-II error is 0.20, the power of the test is 0.80. Type-I (α) and type-II (β) errors can be intentional. The reason to intentionally make such an error is the necessity to look at the events from the opposite perspective.
b. Difference between Groups and ES
ES is defined as the state in which statistical difference also has clinically significance: ES≥0.5 is desirable. The difference between groups is the absolute difference between the groups compared in clinical research.
c. Allocation Ratio of Groups
The allocation ratio of groups is effective in determining the number of samples. If the number of samples is desired to be determined at the lowest level, the rate should be kept as 1/1.
d. Direction of Hypothesis (H1)
The direction of hypothesis in clinical research may be one-sided or two-sided. While one-sided hypotheses hypothesis test differences in the direction of size, two-sided hypotheses hypothesis test differences without direction. The power of the test in two-sided hypotheses is lower than one-sided hypotheses.
After these four variables are determined, they are entered in the appropriate computer program and the number of samples is calculated. Statistical packaged software programs such as Statistica, NCSS and G-Power may be used for power analysis and calculating the number of samples. When the samples size is calculated, if there is a decrease in α, difference between groups, ES and number of samples, then the standard deviation increases and power decreases. The power in two-sided hypothesis is lower. It is ethically appropriate to consider the determination of sample size, particularly in animal experiments, at the beginning of the study. The phase of the study is also important in the determination of number of subjects to be included in drug studies. Usually, phase-I studies are used to determine the safety profile of a drug or product, and they are generally conducted on a few healthy volunteers. If no unacceptable toxicity is detected during phase-I studies, phase-II studies may be carried out. Phase-II studies are proof-of-concept studies conducted on a larger number (100–500) of volunteer patients. When the effectiveness of the drug or product is evident in phase-II studies, phase-III studies can be initiated. These are randomised, double-blinded, placebo or standard treatment-controlled studies. Volunteer patients are periodically followed-up with respect to the effectiveness and side effects of the drug. It can generally last 1–4 years and is valuable during licensing and releasing the drug to the general market. Then, phase-IV studies begin in which long-term safety is investigated (indication, dose, mode of application, safety, effectiveness, etc.) on thousands of volunteer patients.
II. Blinding (Masking) and Randomisation Methods
When the methodology of clinical research is prepared, precautions should be taken to prevent taking sides. For this reason, techniques such as randomisation and blinding (masking) are used. Comparative studies are the most ideal ones in clinical research.
Blinding Method
A case in which the treatments applied to participants of clinical research should be kept unknown is called the blinding method . If the participant does not know what it receives, it is called a single-blind study; if even the researcher does not know, it is called a double-blind study. When there is a probability of knowing which drug is given in the order of application, when uninformed staff administers the drug, it is called in-house blinding. In case the study drug is known in its pharmaceutical form, a double-dummy blinding test is conducted. Intravenous drug is given to one group and a placebo tablet is given to the comparison group; then, the placebo tablet is given to the group that received the intravenous drug and intravenous drug in addition to placebo tablet is given to the comparison group. In this manner, each group receives both the intravenous and tablet forms of the drug. In case a third party interested in the study is involved and it also does not know about the drug (along with the statistician), it is called third-party blinding.
Randomisation Method
The selection of patients for the study groups should be random. Randomisation methods are used for such selection, which prevent conscious or unconscious manipulations in the selection of patients ( 8 ).
No factor pertaining to the patient should provide preference of one treatment to the other during randomisation. This characteristic is the most important difference separating randomised clinical studies from prospective and synchronous studies with experimental groups. Randomisation strengthens the study design and enables the determination of reliable scientific knowledge ( 2 ).
The easiest method is simple randomisation, e.g. determination of the type of anaesthesia to be administered to a patient by tossing a coin. In this method, when the number of samples is kept high, a balanced distribution is created. When the number of samples is low, there will be an imbalance between the groups. In this case, stratification and blocking have to be added to randomisation. Stratification is the classification of patients one or more times according to prognostic features determined by the researcher and blocking is the selection of a certain number of patients for each stratification process. The number of stratification processes should be determined at the beginning of the study.
As the number of stratification processes increases, performing the study and balancing the groups become difficult. For this reason, stratification characteristics and limitations should be effectively determined at the beginning of the study. It is not mandatory for the stratifications to have equal intervals. Despite all the precautions, an imbalance might occur between the groups before beginning the research. In such circumstances, post-stratification or restandardisation may be conducted according to the prognostic factors.
The main characteristic of applying blinding (masking) and randomisation is the prevention of bias. Therefore, it is worthwhile to comprehensively examine bias at this stage.
Bias and Chicanery
While conducting clinical research, errors can be introduced voluntarily or involuntarily at a number of stages, such as design, population selection, calculating the number of samples, non-compliance with study protocol, data entry and selection of statistical method. Bias is taking sides of individuals in line with their own decisions, views and ideological preferences ( 9 ). In order for an error to lead to bias, it has to be a systematic error. Systematic errors in controlled studies generally cause the results of one group to move in a different direction as compared to the other. It has to be understood that scientific research is generally prone to errors. However, random errors (or, in other words, ‘the luck factor’-in which bias is unintended-do not lead to bias ( 10 ).
Another issue, which is different from bias, is chicanery. It is defined as voluntarily changing the interventions, results and data of patients in an unethical manner or copying data from other studies. Comparatively, bias may not be done consciously.
In case unexpected results or outliers are found while the study is analysed, if possible, such data should be re-included into the study since the complete exclusion of data from a study endangers its reliability. In such a case, evaluation needs to be made with and without outliers. It is insignificant if no difference is found. However, if there is a difference, the results with outliers are re-evaluated. If there is no error, then the outlier is included in the study (as the outlier may be a result). It should be noted that re-evaluation of data in anaesthesiology is not possible.
Statistical evaluation methods should be determined at the design stage so as not to encounter unexpected results in clinical research. The data should be evaluated before the end of the study and without entering into details in research that are time-consuming and involve several samples. This is called an interim analysis . The date of interim analysis should be determined at the beginning of the study. The purpose of making interim analysis is to prevent unnecessary cost and effort since it may be necessary to conclude the research after the interim analysis, e.g. studies in which there is no possibility to validate the hypothesis at the end or the occurrence of different side effects of the drug to be used. The accuracy of the hypothesis and number of samples are compared. Statistical significance levels in interim analysis are very important. If the data level is significant, the hypothesis is validated even if the result turns out to be insignificant after the date of the analysis.
Another important point to be considered is the necessity to conclude the participants’ treatment within the period specified in the study protocol. When the result of the study is achieved earlier and unexpected situations develop, the treatment is concluded earlier. Moreover, the participant may quit the study at its own behest, may die or unpredictable situations (e.g. pregnancy) may develop. The participant can also quit the study whenever it wants, even if the study has not ended ( 7 ).
In case the results of a study are contrary to already known or expected results, the expected quality level of the study suggesting the contradiction may be higher than the studies supporting what is known in that subject. This type of bias is called confirmation bias. The presence of well-known mechanisms and logical inference from them may create problems in the evaluation of data. This is called plausibility bias.
Another type of bias is expectation bias. If a result different from the known results has been achieved and it is against the editor’s will, it can be challenged. Bias may be introduced during the publication of studies, such as publishing only positive results, selection of study results in a way to support a view or prevention of their publication. Some editors may only publish research that extols only the positive results or results that they desire.
Bias may be introduced for advertisement or economic reasons. Economic pressure may be applied on the editor, particularly in the cases of studies involving drugs and new medical devices. This is called commercial bias.
In recent years, before beginning a study, it has been recommended to record it on the Web site www.clinicaltrials.gov for the purpose of facilitating systematic interpretation and analysis in scientific research, informing other researchers, preventing bias, provision of writing in a standard format, enhancing contribution of research results to the general literature and enabling early intervention of an institution for support. This Web site is a service of the US National Institutes of Health.
The last stage in the methodology of clinical studies is the selection of intervention to be conducted. Placebo use assumes an important place in interventions. In Latin, placebo means ‘I will be fine’. In medical literature, it refers to substances that are not curative, do not have active ingredients and have various pharmaceutical forms. Although placebos do not have active drug characteristic, they have shown effective analgesic characteristics, particularly in algology applications; further, its use prevents bias in comparative studies. If a placebo has a positive impact on a participant, it is called the placebo effect ; on the contrary, if it has a negative impact, it is called the nocebo effect . Another type of therapy that can be used in clinical research is sham application. Although a researcher does not cure the patient, the researcher may compare those who receive therapy and undergo sham. It has been seen that sham therapies also exhibit a placebo effect. In particular, sham therapies are used in acupuncture applications ( 11 ). While placebo is a substance, sham is a type of clinical application.
Ethically, the patient has to receive appropriate therapy. For this reason, if its use prevents effective treatment, it causes great problem with regard to patient health and legalities.
Before medical research is conducted with human subjects, predictable risks, drawbacks and benefits must be evaluated for individuals or groups participating in the study. Precautions must be taken for reducing the risk to a minimum level. The risks during the study should be followed, evaluated and recorded by the researcher ( 1 ).
After the methodology for a clinical study is determined, dealing with the ‘Ethics Committee’ forms the next stage. The purpose of the ethics committee is to protect the rights, safety and well-being of volunteers taking part in the clinical research, considering the scientific method and concerns of society. The ethics committee examines the studies presented in time, comprehensively and independently, with regard to ethics and science; in line with the Declaration of Helsinki and following national and international standards concerning ‘Good Clinical Practice’. The method to be followed in the formation of the ethics committee should be developed without any kind of prejudice and to examine the applications with regard to ethics and science within the framework of the ethics committee, Regulation on Clinical Trials and Good Clinical Practice ( www.iku.com ). The necessary documents to be presented to the ethics committee are research protocol, volunteer consent form, budget contract, Declaration of Helsinki, curriculum vitae of researchers, similar or explanatory literature samples, supporting institution approval certificate and patient follow-up form.
Only one sister/brother, mother, father, son/daughter and wife/husband can take charge in the same ethics committee. A rector, vice rector, dean, deputy dean, provincial healthcare director and chief physician cannot be members of the ethics committee.
Members of the ethics committee can work as researchers or coordinators in clinical research. However, during research meetings in which members of the ethics committee are researchers or coordinators, they must leave the session and they cannot sign-off on decisions. If the number of members in the ethics committee for a particular research is so high that it is impossible to take a decision, the clinical research is presented to another ethics committee in the same province. If there is no ethics committee in the same province, an ethics committee in the closest settlement is found.
Thereafter, researchers need to inform the participants using an informed consent form. This form should explain the content of clinical study, potential benefits of the study, alternatives and risks (if any). It should be easy, comprehensible, conforming to spelling rules and written in plain language understandable by the participant.
This form assists the participants in taking a decision regarding participation in the study. It should aim to protect the participants. The participant should be included in the study only after it signs the informed consent form; the participant can quit the study whenever required, even when the study has not ended ( 7 ).
Peer-review: Externally peer-reviewed.
Author Contributions: Concept - C.Ö.Ç., A.D.; Design - C.Ö.Ç.; Supervision - A.D.; Resource - C.Ö.Ç., A.D.; Materials - C.Ö.Ç., A.D.; Analysis and/or Interpretation - C.Ö.Ç., A.D.; Literature Search - C.Ö.Ç.; Writing Manuscript - C.Ö.Ç.; Critical Review - A.D.; Other - C.Ö.Ç., A.D.
Conflict of Interest: No conflict of interest was declared by the authors.
Financial Disclosure: The authors declared that this study has received no financial support.
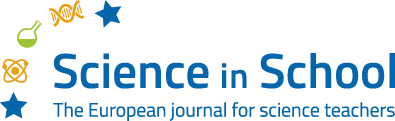
Science in School
What is it good for basic versus applied research teach article.
Author(s): Martin McHugh, Marcus Baumann, Sarah Hayes, F. Jerry Reen, Laurie Ryan, Davide Tiana, Jessica Whelan
Basic research is often misunderstood by the public and misconstrued by the media. Try this role play to learn how research is funded and how basic research advances and protects society.
In 2019, an international research group published a paper examining the effect of the song Scary Monsters and Nice Sprites by Skrillex on the breeding behaviours of mosquitos. [ 1 ] The paper became a viral news story, with many media outlets using the ‘obscure’ research story to generate clicks. However, the research concluded that, when mosquitos were exposed to the song, they bit less and refrained from mating. The paper generated equal amounts of praise and criticism but highlights the potential of basic research and creative thinking in science. Indeed, the historical problem with basic research is the lack of immediate commercial objectives. To non-scientists, basic research can seem like a waste of money, whereas applied research, designed to solve practical problems with obvious scientific and societal benefits, seems like a better use of resources.
The following activity will bring the debate into the classroom and allow students to explore the pros and cons of basic and applied research. Using an argumentation framework, students will discuss the merits of a variety of research projects, with updates to show how some of them later turned out to be important for vaccine development for COVID-19.
What kinds of research should be funded?
In this activity, students will be divided into groups of funders and scientists. Using the materials provided, the scientists will pitch their research proposals to the funders, who will have €100 000 at their disposal. The activity will also provide cues to promote argumentation among students to develop critical thinking, reasoning, communication, and scientific literacy skills. [ 2 ]
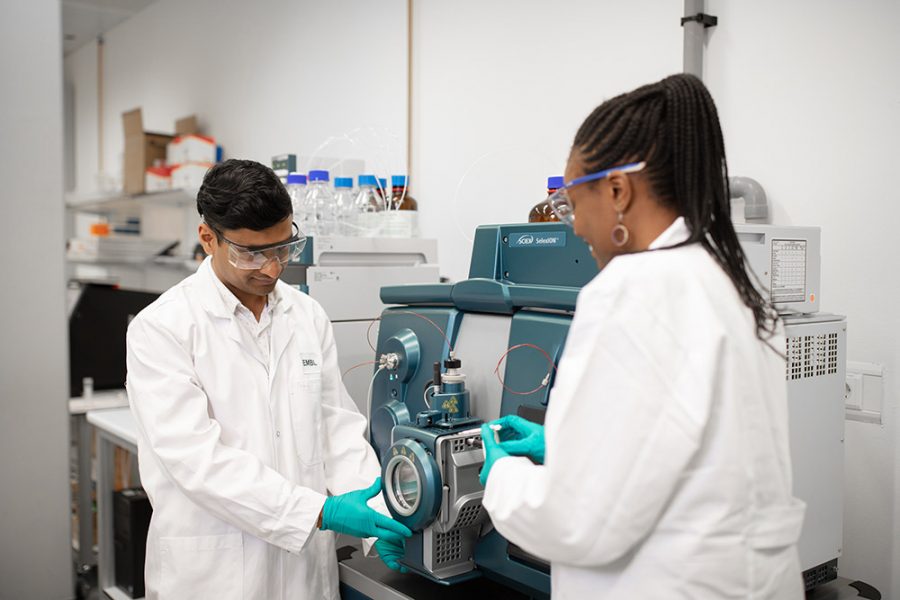
Learning objectives and context
After the activity, students should understand
- how scientific research is funded and that this involves difficult decisions;
- the difference between basic and applied research;
- how applied research relies on basic research findings, and that it is difficult to predict what might become useful.
To set the scene, students should be asked who they think funds scientific research. Students will generate multiple answers, from the government to universities and industry. Truthfully, funding can come from a variety of sources and can be public, private, national, or international.
The next question is how do funding bodies select what research should be funded. Scientific research is often broadly divided into two types: basic research (also called fundamental research) and applied research.
- Basic research is about pushing the boundaries of our understanding and generating new knowledge. An example is researching how a physiological process works at the molecular level.
- Applied research involves applying existing knowledge to create solutions to specific problems. An example is developing a treatment for a disease.
However, many research projects have elements of both basic and applied research. Research scientists from around the world must compete and push the merits of their work to get funding.
The following role-play activity will put students in the shoes of both the funding bodies and scientists. In groups, students will be asked to pitch their project proposal to the funders, who will ultimately decide how to allocate €100 000 to a variety of projects.
A key element of this lesson is to encourage debate and argumentation. Students acting as scientists should try to convince funders with their words. They should be encouraged to make claims, rebuttals, and back up their statements with data, if possible. Each scientist will have an individual text that will give them the information to argue effectively. To support debate, funders are given a list of key questions, along with more probing questions. This activity can also be extended over multiple lessons to allow students time to debate.
Funder information sheet
Project proposal cards
Discussion cards
- For this role-play activity, divide students into groups of five or six. Each group requires four scientists and at least one funder.
- Hand out the project proposal cards to the four scientists in each group.There are four project proposals and each scientist should get a different one. One of these proposals is highly applied, while the others are more basic. All funders receive the same information sheet and can allocate €100 000. If there are two funders in a single group, then they must come to a consensus.
- Give students 10 minutes to read over their documents. Funders need to be aware of the key questions (on the information sheet) they can use to assess the proposals. Scientists need to be aware of the key arguments they need to make to receive funding (on the proposal cards).
- Each scientist then gets 2 minutes uninterrupted to make their ‘pitch’ for funding. Once complete, funders need to ask key questions and all scientists are allowed argue their positions against each other. This should take around 15 minutes.
- At the end of the activity, funders are asked to fill in the funding-allocation table at the bottom of their information sheet. This is to be kept private.
- In turn, ask the funders from each group to the front of the class. The table on their sheets can be copied onto the board and funders can fill this out. Once complete, they need to give a brief justification to the class for their decision.
- Throughout this process, ask the students if they are seeing any patterns emerging in the funding between groups.
- Ask whether the students think each project is more basic or applied.
- Next, hand out the discussion cards to each group. Project 3 is purely applied and has a clear link to vaccines, but these cards describe how proposals 1, 2, and 4 turned out to be fundamental to the development of the COVID-19 vaccine in unexpected ways.
- Get the class to discuss whether this new information would have changed their funding decisions.
- Discuss whether the applications envisioned by the researchers were necessarily those that turned out to be important.
As previously stated, the goal of this activity is that students understand how research is funded and the differences between applied and basic research. The activity is designed to highlight how basic research often forms the foundation for applied research. Both types of research are important, but basic research can be perceived negatively in the eyes of the public. It is often impossible to predict how knowledge gained through a basic research project could be vital for an application in the future. Often multiple scientific advances have to be combined for an applied impact. Sometimes, scientists must accept that they may not be able to identify an immediate application for new knowledge generated. However, without new knowledge, we may lack the foundation for future applications that could be years away.
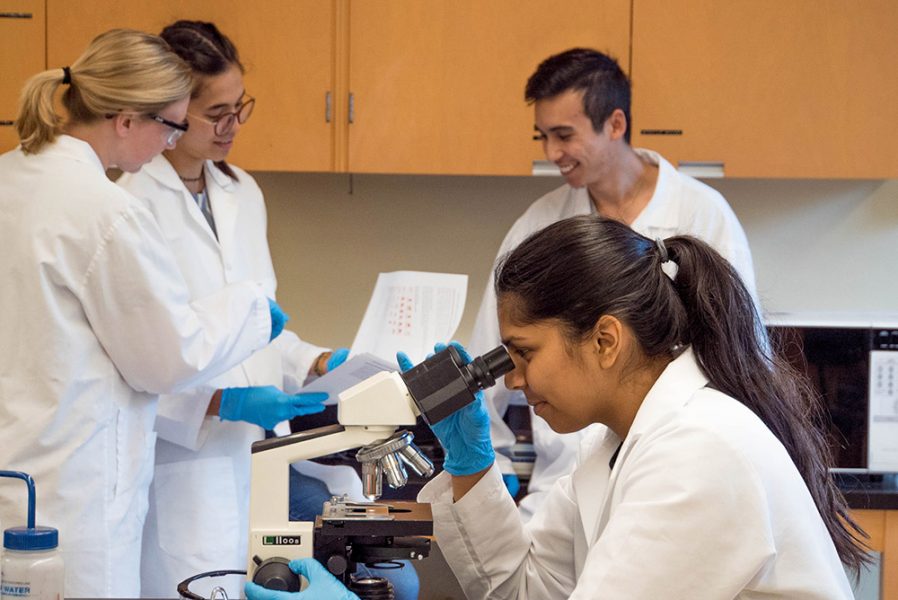
In this example, the three more basic research proposals proved to be vital to the final application. This can be easily illustrated with proposal cards 1 and 3. Proposal card 1 discusses modified mRNA, and this research underpinned the manufacture of the COVID-19 vaccine. The two proposals are so closely linked that you can replace the word ‘polynucleotide(s)’ with mRNA on proposal card 3 and the document still makes perfect sense.
As a follow up to this activity, ask students to go online and find the most obscure and weird basic scientific research (that has been published in a peer-reviewed journal) they can find. The Ig Nobel Prizes are a good source of inspiration for this. Similar to the mosquito example used in the introduction to this activity, get the students to find practical applications behind the headlines and articles.
[1] Dieng H et al. (2019). The electronic song “Scary Monsters and Nice Sprites” reduces host attack and mating success in the dengue vector Aedes aegypti . Acta tropica 194 :93–99. doi: 10.1016/j.actatropica.2019.03.027
[2] Erduran S, Ozdem Y, Park JY (2015). Research trends on argumentation in science education: a journal content analysis from 1998–2014 . International Journal of STEM Education , 2 :5. doi: 10.1186/s40594-015-0020-1.
- Discover CRISPR-Cas9 and how it revolutionized gene editing: Chan H (2016) Faster, cheaper, CRISPR: the new gene technology revolution . Science in School 38 :18–21.
- Read an article on different techniques to resolve and predict protein structures: Heber S (2021) From gaming to cutting-edge biology: AI and the protein folding problem . Science in School 52 .
- Read an article on how modern vaccines work: Paréj K (2021) Vaccines in the spotlight . Science in School 53 .
- Visit the Annals of Improbable Research , which runs the Ig Nobel Prizes, to learn more about research that makes you laugh and then makes you think.
- Read a simple explanation of basic research and its importance from the National Institute of Health.
- Read a short article from Harvard University on the importance of basic research .
- Watch a video on the potential uses of CRISPR outside gene editing.
- Watch a video on how 50 years of fundamental research enabled the rapid development of mRNA vaccines for COVID-19.
- Read an article from STAT describing the main steps that – 50 years later – led to COVID-19 mRNA vaccines .
- Watch a video introducing the ESRF and its 41 beamlines .
- Read an article from Scientific American that underlines the important issue of research funding and final profits .
- Read an article from c&en magazine on synchrotrons and their uses .
- Read an interview with Katalin Karikó in Scientific American that discusses her role in developing the mRNA technology used in COVID-19 vaccines.
Dr Martin McHugh is the education and public engagement officer for SSPC , the Science Foundation Ireland (SFI) research centre for pharmaceuticals at the University of Limerick. Formerly a researcher in informal learning and part-time lecturer on science education, he has degrees from NUI Galway and the University of Edinburgh in environmental science and teaching. He is also a qualified secondary school science and biology teacher.
Dr Marcus Baumann is an assistant professor in the School of Chemistry at University College Dublin. He leads a research group aiming to develop new methods for the sustainable generation of drug-like molecules through the use of continuous-flow technologies. These methods are based on using light and enzymes in combination with machines to synthesise biologically active molecules.
Dr Sarah Hayes is the chief operating officer (COO) of SSPC . Sarah’s background is in physical chemistry and she received her PhD in Science Education. Sarah has many years of teaching experience as a physics and chemistry teacher. Through her various roles, she has been involved in research, curriculum development, and continuous professional development courses. Her most significant focus has been informal and non-formal learning and engagement.
Dr Jerry Reen is a lecturer in molecular microbial ecology at University College Cork. His research team study polymicrobial biofilm communities to understand molecular communication systems between species in disease and biotechnology. They also apply molecular technologies to harness biocatalytic proteins and bioactive compounds of marine origin.
Laurie Ryan is an assistant lecturer in general science at Athlone Institute of Technology (AIT). She is a former secondary school science teacher and conducts research in the area of STEM education and outreach. She is currently finishing her PhD examining argumentation in non-formal learning environments.
Dr Davide Tiana is a lecturer in inorganic chemistry at University College Cork. His independent group uses computational chemistry to study, understand, and explain chemistry. Their research goals range from developing new models to better explain chemical interactions (e.g., chemical bonding, dispersion forces) to the design of new molecules such as nanodrugs.
Dr Jessica Whelan is a lecturer at the University College Dublin School of Chemical and Bioprocess Engineering. Her research focuses on developing tools and approaches to optimize the production of proteins, vaccines, and cell and gene therapies. The aim is to make medicines available to patients at the highest quality and lowest cost possible.
Supporting materials
Download this article as a PDF
Share this article
Subscribe to our newsletter.
Thank you for visiting nature.com. You are using a browser version with limited support for CSS. To obtain the best experience, we recommend you use a more up to date browser (or turn off compatibility mode in Internet Explorer). In the meantime, to ensure continued support, we are displaying the site without styles and JavaScript.
- View all journals
- My Account Login
- Explore content
- About the journal
- Publish with us
- Sign up for alerts
- Open access
- Published: 03 February 2023
The value of applied science
Nature Communications volume 14 , Article number: 587 ( 2023 ) Cite this article
34k Accesses
8 Altmetric
Metrics details
Every area of science can contribute to the changes that are required for a sustainable future through the application of its fundamental discoveries. While some fields have clear paths to application, Nature Communications believes that there is great potential for utility and application to be found in, and across, all of the different disciplines that we publish.
In 1932, Nature published an article entitled Relations between Pure and Applied Science that argued strongly for the application of scientific ideas to get the recognition it deserved. In the intervening 90 years, the central thesis of the article still rings true: the importance of applied science should not be overlooked.
In medicine, there’s the often used term ‘bench to bedside’ to describe how fundamental discoveries of important biological insight become the techniques and medicines that save or improve lives. Similarly, for the development of new and improved materials, energy systems, and electronics, to name but a few, we need applied science to develop our initial discoveries into usable technologies. It is this application of insight into a system that brings discoveries out of the laboratory and into the everyday world. The line between the pure and the applied sciences is not a hard border, but a bridge linking the two.
Today’s world faces problems with complex and multifaceted causes. From the continuing impact of SARS-CoV-2 to the crisis of climate change and the work necessary to achieve the Sustainable Development Goals, science and technology will play an important role in addressing the problems we collectively face. If the world is to build a sustainable and equitable future, with rising standards of living for all, advances in our scientific understanding need to go from the bench to not just the bedside but into every aspect of life.
To provide a home for these studies, we recognise that manuscripts presenting applications should be assessed on different criteria from those reporting a novel discovery. It is important to sometimes put aside the requirement for novel insight and rather consider potential uses and current challenges faceing effective application. When reaching a decision on the suitability of such manuscripts for publication, we will use as guidance parameters such as the Technology Readiness Level [Box 1]—an estimation of the maturity of a technology originally developed by NASA and now widely adopted—of an application, as well as an assessment of how market ready a technology or application is.
“It is important to sometimes put aside the requirement for novel insight and rather consider potential uses and current challenges facing effective application.”
Technology readiness levels (TRL).
TRL 1: Basic principles are observed and reported
TRL 2: Concept or application is formulated
TRL 3: Demonstration of analytical and experimental critical function or characteristic proof-of-concept
TRL 4: Demonstration of basic validation of the technology in a laboratory environment
TRL 5: Demonstration of basic validation of the technology in a relevant environment
TRL 6: Model or prototype demonstration of the technology in a relevant environment
TRL 7: Prototype demonstration of the technology in an operational environment
TRL 8: Actual technology completed and qualified through testing and demonstration
TRL 9: Actual technology qualified through successful operational use.
Just as editors and reviewers should think in terms of utility over discovery for those applied studies, we also encourage authors to consider the potential applications of their work and plan ahead for commercialisation and patentability when applicable. While as a journal we neither encourage nor discourage this—as only the authors of a work can make these decisions—the potential commercialisation of research should not be seen as a barrier to publication.
Our Perspectives and Comments have always provided a way for authors to give their views on where the research community should be heading. We recognise that the deployment of the applied sciences requires more than retrospective commissioned content reviewing a field’s development, and needs to include forward-facing papers that help to guide a field.
To help bridge the academic-commercial divide, we have reached out to authors that move in both worlds for insight and guidance on how to transition research from academia to industry. We have a Comment from William Hait and Paulus Stoffels at Johnson & Johnson, giving guidance on academic–industrial partnerships , and a discussion about commercialisation of research from an academic standpoint by Professor Kylie Vincent. Also, in the Q&A with Dr. Andrea Crottini we look at research commercialisation from the point of view of a technology transfer officer. To highlight some of the great content we receive in this area, our editors have also curated a Collection of research articles on applied science across the chemical, physical, materials, and biological sciences. We will continue to update this collection as new work is published, so be sure to check back regularly for the latest additions. We encourage you to submit your applied work to Nature Communications for consideration in the collection.
Whether your work involves fundamental basic science discoveries or the development of new technologies and methods, whether you wish to patent or not, whether the work is from an academic laboratory, an established company or a young start-up just spun out from a university, the editors at Nature Communications want to ensure we can support all of our authors in finding a home for their work.
Rights and permissions
Open Access This article is licensed under a Creative Commons Attribution 4.0 International License, which permits use, sharing, adaptation, distribution and reproduction in any medium or format, as long as you give appropriate credit to the original author(s) and the source, provide a link to the Creative Commons license, and indicate if changes were made. The images or other third party material in this article are included in the article’s Creative Commons license, unless indicated otherwise in a credit line to the material. If material is not included in the article’s Creative Commons license and your intended use is not permitted by statutory regulation or exceeds the permitted use, you will need to obtain permission directly from the copyright holder. To view a copy of this license, visit http://creativecommons.org/licenses/by/4.0/ .
Reprints and permissions
About this article
Cite this article.
The value of applied science. Nat Commun 14 , 587 (2023). https://doi.org/10.1038/s41467-023-36307-4
Download citation
Published : 03 February 2023
DOI : https://doi.org/10.1038/s41467-023-36307-4
Share this article
Anyone you share the following link with will be able to read this content:
Sorry, a shareable link is not currently available for this article.
Provided by the Springer Nature SharedIt content-sharing initiative
Quick links
- Explore articles by subject
- Guide to authors
- Editorial policies
Sign up for the Nature Briefing newsletter — what matters in science, free to your inbox daily.

Applied science
Combining computer science with physics and biology to create breakthroughs that help the world.
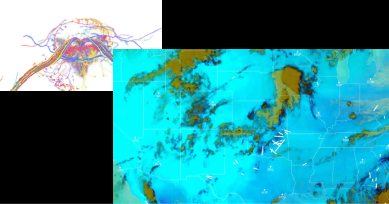
About the team
Computer science and natural science are complementary: breakthroughs in one can lead to remarkable advances in the other. The goal of the Applied Science organization at Google is to cross-fertilize these two fields. There are four main efforts in Applied Science: Quantum Computing, Google Accelerated Science, Climate and Energy, and Scientific Computing Tools.
Quantum Computing uses advances in applied physics to push the state-of-the-art in computation. Google Accelerated Science and Climate and Energy do the opposite: they use the latest advances in machine learning and artificial intelligence to accelerate progress in natural sciences, including societally-important areas such as biomedical research and zero-carbon energy sources. Finally, we supply Scientific Computing Tools such as Colab to many internal groups to enhance their data and machine learning productivity.
Team focus summaries
Climate and energy.
The Climate & Energy team is exploring how to use large-scale computing and machine intelligence to diminish or avoid climate disruption. We partner with fusion companies to accelerate the progress of commercially viable fusion, model techno-economic scenarios for decarbonization, research new techniques for carbon sequestration, and look for novel ways to improve our understanding of earth's ecosystem response to climate change.
The physics team seeks to combine advances in machine learning and other Google technologies to advance our understanding of the physical world. Current projects range from machine learning for scientific computing, developing efficient algorithms for solving nonlinear partial differential equations, applying differentiable algorithms to interpret microscopy data, enabling microscopy on phones, and building algorithms for understanding dysarthric speech.
The Quantum AI Lab is building quantum processors and algorithms to dramatically accelerate computational tasks for machine intelligence. We are developing quantum algorithms with a particular focus on those which can already run on today’s pre-error corrected quantum processors. Quantum algorithms for optimization, sampling, and quantum simulation hold the promise of dramatic speedups over the fastest classical computers.
Featured publications
Highlighted work.
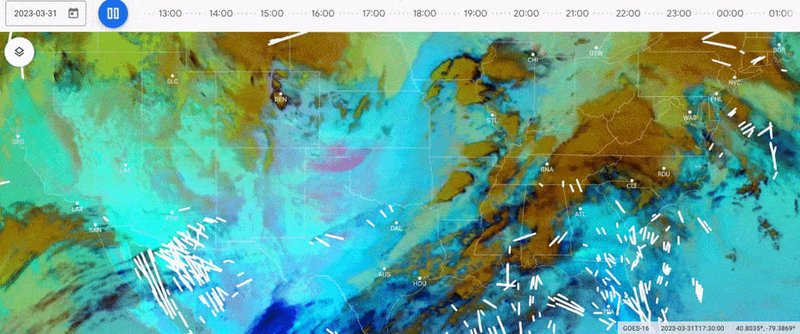
Some of our locations
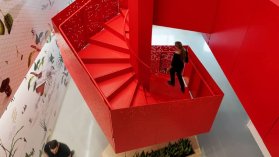
Some of our people
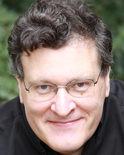
John C. Platt
- General Science
- Machine Intelligence
- Quantum Computing
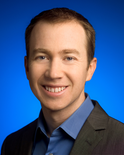
Jason Miller
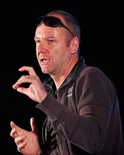
Hartmut Neven
- Hardware and Architecture
- Human-Computer Interaction and Visualization
- Algorithms and Theory
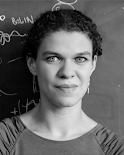
Marissa Giustina
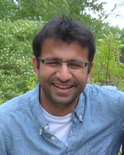
Varun Gulshan
- Machine Perception

Michael P Brenner
- Health & Bioscience
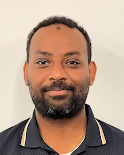
- Education Innovation
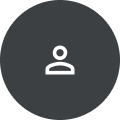
Christopher H Van Arsdale
- Economics and Electronic Commerce
- Distributed Systems and Parallel Computing
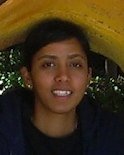
Subhashini Venugopalan
We're always looking for more talented, passionate people.
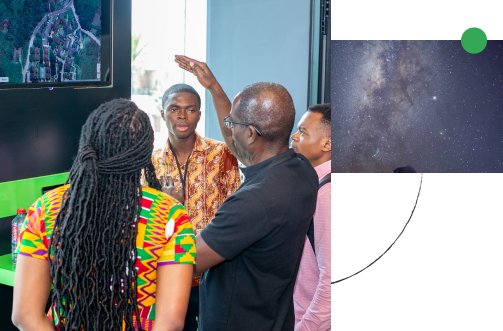
What is Applied Research? Definition, Types, Examples
Appinio Research · 10.01.2024 · 35min read
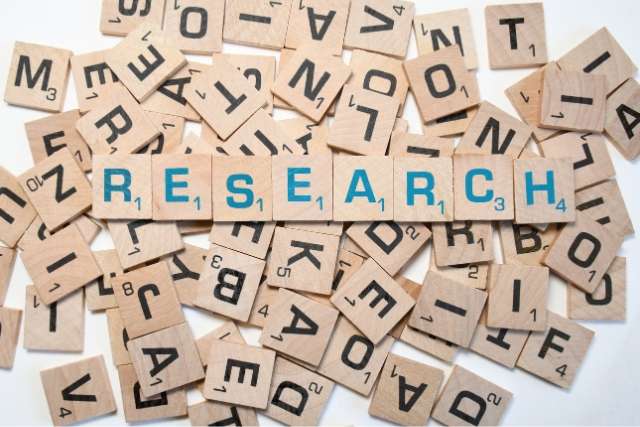
Ever wondered how groundbreaking solutions to real-world challenges are developed, or how innovations come to life? Applied research holds the key. In this guide, we will delve deep into the world of applied research, uncovering its principles, methodologies, and real-world impact. From harnessing cutting-edge technology to address healthcare crises to revolutionizing industries through data-driven insights, we'll explore the diverse domains where applied research thrives.
What is Applied Research?
Applied research is a systematic and organized inquiry aimed at solving specific real-world problems or improving existing practices, products, or services. Unlike basic research, which focuses on expanding general knowledge, applied research is all about using existing knowledge to address practical issues.
The primary purpose of applied research is to generate actionable insights and solutions that have a direct impact on practical situations. It seeks to bridge the gap between theory and practice by taking existing knowledge and applying it in real-world contexts. Applied research is driven by the need to address specific challenges, make informed decisions, and drive innovation in various domains.
Importance of Applied Research
Applied research holds immense significance across various fields and industries. Here's a list of reasons why applied research is crucial:
- Problem Solving: Applied research provides effective solutions to real-world problems, improving processes, products, and services.
- Innovation: It drives innovation by identifying opportunities for enhancement and developing practical solutions.
- Evidence-Based Decision-Making: Policymakers and decision-makers rely on applied research findings to make informed choices and shape effective policies.
- Competitive Advantage: In business, applied research can lead to improved products, increased efficiency, and a competitive edge in the market.
- Social Impact: Applied research contributes to solving societal issues, from healthcare improvements to environmental sustainability.
- Technological Advancement: In technology and engineering, it fuels advancements by applying scientific knowledge to practical applications.
Applied Research vs. Basic Research
Applied research differs from basic research in several key ways:
- Objectives: Applied research aims to address specific practical problems or improve existing processes, while basic research seeks to expand general knowledge.
- Focus: Applied research focuses on solving real-world challenges, whereas basic research explores fundamental principles and concepts.
- Applicability: Applied research findings are directly applicable to practical situations, while basic research often lacks immediate practical applications.
- Immediate Impact: Applied research has a more immediate impact on solving problems and improving practices, whereas basic research may have longer-term or indirect effects on knowledge and innovation.
- Research Questions: Applied research formulates research questions related to practical issues, while basic research poses questions to explore theoretical or fundamental concepts.
Understanding these distinctions is essential for researchers, policymakers, and stakeholders in various fields, as it guides the choice of research approach and the expected outcomes of a research endeavor.
Types of Applied Research
Applied research encompasses various types, each tailored to specific objectives and domains. Understanding these types is essential for choosing the right approach to address real-world problems effectively. Here are some common types of applied research, each with its distinct focus and methodologies.
Evaluation Research
Purpose: Evaluation research assesses the effectiveness, efficiency, and impact of programs, interventions, or policies. It aims to determine whether these initiatives meet their intended goals and objectives.
Methodology: Researchers employ a range of quantitative and qualitative methods , including surveys, interviews, observations, and data analysis, to evaluate the outcomes and outcomes of programs or interventions.
Example: Evaluating the impact of a public health campaign aimed at reducing smoking rates by analyzing pre- and post-campaign survey data on smoking habits and attitudes.
Action Research
Purpose: Action research focuses on solving practical problems within a specific organizational or community context. It involves collaboration between researchers and practitioners to implement and assess solutions.
Methodology: Action research is iterative and participatory, with researchers and stakeholders working together to identify problems, develop interventions, and assess their effectiveness. It often involves cycles of planning, action, reflection, and adjustment.
Example: Teachers collaborating with researchers to improve classroom teaching methods and student outcomes by implementing and refining innovative teaching strategies.
Case Study Research
Purpose: Case study research investigates a particular individual, organization, or situation in-depth to gain a comprehensive understanding of a specific phenomenon or issue.
Methodology: Researchers collect and analyze a wealth of data, which may include interviews, documents, observations, and archival records. The goal is to provide a detailed and context-rich description of the case.
Example: A detailed examination of a successful startup company's growth strategies and challenges, offering insights into factors contributing to its success.
Applied Experimental Research
Purpose: Applied experimental research seeks to establish causal relationships between variables by manipulating one or more factors and observing their impact on outcomes. It helps determine cause-and-effect relationships in real-world settings.
Methodology: Researchers conduct controlled experiments, similar to those in basic research, but within practical contexts. They manipulate variables and use statistical analysis to assess their effects on specific outcomes.
Example: Testing the impact of different website designs on user engagement and conversion rates by randomly assigning visitors to various design versions and measuring their interactions.
Survey Research
Purpose: Survey research involves collecting data from a sample of individuals or organizations to understand their opinions, attitudes, behaviors, or characteristics. It is commonly used to gather quantitative data on specific topics.
Methodology: Researchers design surveys with carefully crafted questions and administer them to a representative sample of the target population . Statistical analysis is used to draw conclusions based on survey responses.
Example: Conducting a national survey to assess public sentiment and preferences on environmental conservation initiatives and policies.
These types of applied research provide a framework for approaching real-world challenges systematically. Researchers can choose the most appropriate type based on their research goals, objectives, and the nature of the problem or phenomenon they seek to address. By selecting the right approach, applied researchers can generate valuable insights and practical solutions in various fields and disciplines.
How to Prepare for Applied Research?
In the preparatory phase of your applied research journey, you'll lay the groundwork for a successful study. This phase involves a series of crucial steps that will shape the direction and ethics of your research project.
Identifying Research Questions
One of the key starting points for any applied research endeavor is identifying the right research questions. Your research questions should be clear, specific, and directly related to the problem or issue you aim to address.
- Engage with Stakeholders: Reach out to individuals or groups who are affected by or have an interest in the issue you're researching. Their perspectives can help you formulate relevant questions.
- Consider Feasibility: Ensure that your research questions are feasible within your available resources, including time, budget, and access to data or participants.
- Prioritize Impact: Focus on questions that have the potential to create meaningful change or provide valuable insights in your chosen field.
Formulating Hypotheses
Hypotheses serve as the guiding stars of your research, providing a clear direction for your investigation. Formulating hypotheses is a critical step that sets the stage for testing and validating your ideas.
- Testable Predictions: Your hypotheses should be testable and capable of being proven or disproven through empirical research.
- Informed by Literature: Base your hypotheses on existing knowledge and insights gained from the literature review. They should build upon what is already known and aim to expand that knowledge.
- Clarity and Precision: Write your hypotheses in a clear and precise manner, specifying the expected relationship or outcome you intend to explore.
Literature Review
Conducting a thorough literature review is like embarking on a treasure hunt through existing knowledge in your field. It's a comprehensive exploration of what other researchers have already discovered and what gaps in knowledge still exist.
- Search Strategies: Utilize academic databases, journals, books, and credible online sources to search for relevant literature.
- Analyze Existing Research: Examine the findings, methodologies, and conclusions of previous studies related to your research topic.
- Identify Research Gaps: Look for areas where current knowledge is insufficient or contradictory. These gaps will be the foundation for your own research.
Data Collection Methods
Selecting the proper data collection methods is crucial to gather the information needed to address your research questions. The choice of methods will depend on the nature of your research and the type of data you require.
- Quantitative vs. Qualitative: Decide whether you will collect numerical data (quantitative) or focus on descriptive insights and narratives (qualitative).
- Survey Design : If surveys are part of your data collection plan, carefully design questions that are clear, unbiased, and aligned with your research goals.
- Sampling Strategies: Determine how you will select participants or data points to ensure representativeness and reliability.
Ethical Considerations
Ethical considerations are at the heart of responsible research. Ensuring that your study is conducted ethically and with integrity is paramount.
- Informed Consent: Obtain informed consent from participants, ensuring they understand the purpose of the research, potential risks, and their right to withdraw at any time.
- Confidentiality: Safeguard participants' personal information and ensure their anonymity when reporting findings.
- Minimizing Harm: Take measures to mitigate any physical or emotional harm that participants may experience during the research process.
- Ethical Reporting: Accurately represent your research findings, avoiding manipulation or selective reporting that may mislead readers or stakeholders.
By diligently addressing these aspects of research preparation, you are building a solid foundation for your applied research project, setting the stage for effective data collection and meaningful analysis in the subsequent phases of your study.
How to Design Your Research Study?
When it comes to applied research, the design of your study is paramount. It shapes the entire research process, from data collection to analysis and interpretation. In this section, we will explore the various elements that make up the foundation of your research design.
Research Design Types
Your choice of research design is like selecting the blueprint for your research project. Different research design types offer unique advantages and are suited for different research questions. Here are some common research design types:
- Experimental Design : In this design, researchers manipulate one or more variables to observe their impact on outcomes. It allows for causal inference but may not always be feasible in applied research due to ethical or practical constraints.
- Descriptive Design: This design aims to describe a phenomenon or population without manipulating variables. It is often used when researchers want to provide a snapshot of a situation or gain insights into a specific context.
- Correlational Design : In this design, researchers examine relationships between variables without manipulating them. It helps identify associations but does not establish causation.
- Longitudinal Design : Longitudinal studies involve collecting data from the same subjects over an extended period. They are valuable for tracking changes or developments over time.
- Cross-Sectional Design : This design involves data collection from a diverse group of subjects at a single point in time. It's helpful in studying differences or variations among groups.
Sampling Methods
Sampling methods determine who or what will be included in your study. The choice of sampling method has a significant impact on the generalizability of your findings. Here are some standard sampling methods:
- Random Sampling: This method involves selecting participants or data points entirely at random from the population. It ensures every element has an equal chance of being included, which enhances representativeness .
- Stratified Sampling: In stratified sampling, the population is divided into subgroups or strata, and then random samples are drawn from each stratum. This method ensures that each subgroup is adequately represented.
- Convenience Sampling: Researchers choose subjects or data points that are readily available and accessible. While convenient, this method may lead to sampling bias as it may not accurately represent the entire population.
- Purposive Sampling: In purposive sampling, researchers deliberately select specific individuals or groups based on their expertise, experience, or relevance to the research topic. It is often used when seeking specialized knowledge.
Data Collection Tools
Selecting the right data collection tools is essential to gather accurate and relevant information. Your choice of tools will depend on the research design and objectives. Standard data collection tools include:
- Questionnaires and Surveys: These structured instruments use standardized questions to gather data from participants. They are suitable for collecting large amounts of quantitative data.
- Interviews: Interviews can be structured, semi-structured, or unstructured. They provide an opportunity to gather in-depth, qualitative insights from participants.
- Observation: Direct observation involves systematically watching and recording behaviors or events. It's valuable for studying behaviors or phenomena in their natural context.
- Secondary Data : Researchers can also utilize existing data sources, such as government reports, databases, or historical records, for their research.
Variables and Measurement
Defining variables and choosing appropriate measurement methods is crucial for ensuring the reliability and validity of your research. Variables are characteristics, phenomena, or factors that can change or vary in your study. They can be categorized into:
- Independent Variables: These are the variables you manipulate or control in your study to observe their effects on other variables.
- Dependent Variables: These are the variables you measure to assess the impact of the independent variables.
Choosing the right measurement techniques, scales, or instruments is essential to accurately quantify variables and collect valid data. It's crucial to establish clear operational definitions for each variable to ensure consistency in measurement.
Data Analysis Techniques
Once you have collected your data, the next step is to analyze it effectively. Data analysis involves:
- Data Cleaning: Removing any errors, inconsistencies, or outliers from your dataset to ensure data quality.
- Statistical Analysis : Depending on your research design and data type, you may use various statistical techniques such as regression analysis , t-tests, ANOVA, or chi-square tests.
- Qualitative Analysis: For qualitative data, techniques like thematic analysis, content analysis, or discourse analysis help uncover patterns and themes.
- Data Visualization: Using graphs, charts, and visual representations to present your data effectively.
Selecting the appropriate analysis techniques depends on your research questions, data type, and objectives. Proper data analysis is crucial for drawing meaningful conclusions and insights from your research.
With a solid understanding of research design, sampling methods, data collection tools, variables, and measurement, you are well-equipped to embark on your applied research journey. These elements lay the groundwork for collecting valuable data and conducting meaningful analyses in the subsequent phases of your study.
How to Conduct Applied Research?
Now that you've prepared and designed your research study, it's time to delve into the practical aspects of conducting applied research. This phase involves the execution of your research plan, from collecting data to drawing meaningful conclusions. Let's explore the critical components in this stage.
Data Collection Phase
The data collection phase is where your research plan comes to life. It's a crucial step that requires precision and attention to detail to ensure the quality and reliability of your data.
- Implement Data Collection Methods: Execute the data collection methods you've chosen, whether they involve surveys, interviews, observations, or the analysis of existing datasets.
- Maintain Consistency: Ensure that data collection is carried out consistently according to your research design and protocols. Minimize any variations or deviations that may introduce bias .
- Document the Process: Keep thorough records of the data collection process. Note any challenges, unexpected occurrences, or deviations from your original plan. Documentation is essential for transparency and replication.
- Quality Assurance: Continuously monitor the quality of the data you collect. Check for errors, missing information, or outliers. Implement data validation and cleaning procedures to address any issues promptly.
- Participant Engagement: If your research involves human participants, maintain open and respectful communication with them. Address any questions or concerns and ensure participants' comfort and willingness to participate.
Data Analysis Phase
Once you've collected your data, it's time to make sense of the information you've gathered. The data analysis phase involves transforming raw data into meaningful insights and patterns.
- Data Preparation: Start by organizing and cleaning your data. This includes dealing with missing values, outliers, and ensuring data consistency.
- Selecting Analysis Methods: Depending on your research design and data type, choose the appropriate statistical or qualitative analysis methods. Common techniques include regression analysis , content analysis, or thematic coding .
- Conducting Analysis: Perform the chosen analysis systematically and according to established protocols. Ensure that your analysis is reproducible by documenting every step.
- Interpreting Results: Interpretation involves making sense of your findings in the context of your research questions and hypotheses. Consider the statistical significance of the results and any practical implications they may have.
- Visualization: Create visual representations of your data, such as graphs, charts, or tables, to convey your findings effectively. Visualizations make complex data more accessible to a broader audience.
Interpretation of Results
Interpreting research results is a critical step that bridges the gap between data analysis and drawing conclusions. This process involves making sense of the patterns and insights that emerge from your analysis.
- Relate to Hypotheses: Determine whether your results support or refute your hypotheses. Be prepared to explain any unexpected findings.
- Contextualize Findings: Consider the broader context in which your research takes place. How do your results fit into the larger body of knowledge in your field?
- Identify Patterns : Highlight significant trends, correlations, or relationships you've uncovered. Discuss their practical implications and relevance.
- Acknowledge Limitations: Be transparent about any limitations in your study that may affect the interpretation of results. This includes sample size, data quality, and potential biases.
Drawing Conclusions
Drawing conclusions is the ultimate goal of your research. It involves synthesizing your findings and answering the research questions you initially posed.
- Answer Research Questions: Explicitly address the research questions you formulated at the beginning of your study. State whether your findings confirm or challenge your initial hypotheses.
- Highlight Insights: Emphasize the key insights and contributions of your research. Discuss the practical implications of your findings and their relevance to the field.
- Recommend Actions: Based on your conclusions, suggest practical steps, recommendations, or future research directions. How can your research contribute to addressing the problem or challenge you investigated?
- Consider Implications: Reflect on the broader implications of your research for stakeholders, policymakers, or practitioners in your field.
Common Pitfalls to Avoid
During the data collection, analysis, interpretation, and conclusion-drawing phases, it's essential to be aware of common pitfalls that can affect the quality and integrity of your research.
- Sampling Bias : Ensure that your sample is representative of the population you intend to study. Address any bias that may have been introduced during data collection.
- Data Manipulation: Avoid manipulating or selectively reporting data to fit preconceived notions. Maintain transparency in your analysis and reporting.
- Overinterpretation: Be cautious of drawing overly broad conclusions based on limited data. Acknowledge the limitations of your study.
- Ignoring Ethical Considerations: Continuously uphold ethical standards in your research, from data collection to reporting. Protect participants' rights and privacy.
- Lack of Validation: Ensure that the methods and tools you use for data collection and analysis are valid and reliable. Validation helps establish the credibility of your findings.
By navigating the data collection, analysis, interpretation, and conclusion-drawing phases with care and attention to detail, you'll be well-prepared to confidently share your research findings and contribute to advancing knowledge in your field.
How to Report Applied Research Results?
Now that you've conducted your applied research and drawn meaningful conclusions, it's time to share your insights with the world. Effective reporting and communication are crucial to ensure that your research has a real impact and contributes to the broader knowledge base.
Writing Research Reports
Writing a comprehensive research report is the cornerstone of communicating your findings. It provides a detailed account of your research process, results, and conclusions. Here's what you need to consider:

Structure of a Research Report
- Title: Create a concise, informative title that reflects the essence of your research.
- Abstract: Summarize your research in a clear and concise manner, highlighting key objectives, methods, results, and conclusions.
- Introduction: Provide an overview of your research topic, objectives, significance, and research questions.
- Literature Review: Summarize relevant literature and identify gaps in existing knowledge that your research addresses.
- Methodology: Describe your research design, sampling methods, data collection tools, and data analysis techniques.
- Results: Present your findings using tables, charts, and narratives. Be transparent and objective in reporting your results.
- Discussion: Interpret your results, discuss their implications, and relate them to your research questions and hypotheses.
- Conclusion: Summarize your main findings, their significance, and the implications for future research or practical applications.
- References: Cite all sources and studies you referenced in your report using a consistent citation style (e.g., APA, MLA).
Writing Tips
- Use clear and concise language, avoiding jargon or overly technical terms.
- Organize your report logically, with headings and subheadings for easy navigation.
- Provide evidence and data to support your claims and conclusions.
- Consider your target audience and tailor the report to their level of expertise and interest.
Creating Visualizations
Visualizations are powerful tools for conveying complex data and making your research findings more accessible. Here are some types of visualizations commonly used in research reports:
Charts and Graphs
- Bar Charts: Ideal for comparing categories or groups.
- Line Charts: Effective for showing trends or changes over time.
- Pie Charts: Useful for displaying proportions or percentages.
- Data Tables: Present numerical data in an organized format.
- Cross-tabulations: Show relationships between variables.
Diagrams and Maps
- Flowcharts: Visualize processes or workflows.
- Concept Maps: Illustrate connections between concepts.
- Geographic Maps: Display spatial data and patterns.
When creating visualizations:
- Choose the correct type of visualization for your data and research questions.
- Ensure that visualizations are labeled, clear, and easy to understand.
- Provide context and explanations to help readers interpret the visuals.
Presenting Your Research
Presenting your research to an audience is an opportunity to engage, educate, and inspire. Whether it's through a conference presentation, seminar, or webinar, effective communication is vital.
- Know Your Audience: Tailor your presentation to the interests and expertise of your audience.
- Practice: Rehearse your presentation to ensure a smooth delivery and confident demeanor.
- Use Visual Aids: Enhance your presentation with visual aids such as slides, images, or videos.
- Engage with Questions: Encourage questions and discussions to foster interaction and clarify points.
- Stay within Time Limits: Respect time constraints and stay on schedule.
Peer Review Process
Before your research is published, it typically undergoes a peer review process. This involves experts in your field evaluating the quality, validity, and significance of your work. The peer review process aims to ensure the integrity and credibility of your research.
- Submission: Submit your research manuscript to a journal or conference for review.
- Editorial Review: The editorial team assesses your submission's fit with the journal's scope and may conduct an initial review for quality and compliance.
- Peer Review: Your manuscript is sent to peer reviewers who evaluate it for methodology, validity, significance, and adherence to ethical standards.
- Feedback and Revision: Based on reviewers' feedback, you may be asked to revise and improve your research.
- Acceptance or Rejection: After revisions, the manuscript is reevaluated, and a decision is made regarding publication.
Publishing Your Research
Publishing your research is the final step in sharing your findings with the broader scientific community. It allows others to access and build upon your work. Consider the following when choosing where to publish:
- Journal Selection: Choose a reputable journal that aligns with your research field and target audience.
- Review Process: Understand the journal's peer review process and requirements for submission.
- Open Access: Consider whether you want your research to be open access, freely accessible to all.
Once published, actively promote your research through academic networks, conferences, and social media to maximize its reach and impact.
By effectively reporting and communicating your research findings, you contribute to the advancement of knowledge, inspire others, and ensure that your hard work has a meaningful impact on your field and beyond.
Applied Research Examples
To provide a deeper understanding of applied research's impact and relevance, let's delve into specific real-world examples that demonstrate how this type of research has addressed pressing challenges and improved our lives in tangible ways.
Applied Medical Research: mRNA Vaccines
Example: mRNA (messenger RNA) vaccine technology, exemplified by the COVID-19 vaccines developed by Pfizer-BioNTech and Moderna, is a remarkable achievement in the field of applied medical research.
Applied researchers in this domain utilized mRNA technology to create vaccines that provide immunity against the SARS-CoV-2 virus. Unlike traditional vaccines, which use weakened or inactivated viruses, mRNA vaccines instruct cells to produce a harmless spike protein found on the virus's surface. The immune system then recognizes this protein and mounts a defense, preparing the body to combat the actual virus.
Impact: The rapid development and deployment of mRNA vaccines during the COVID-19 pandemic have been groundbreaking. They've played a crucial role in controlling the spread of the virus and saving countless lives worldwide. This example underscores how applied research can revolutionize healthcare and respond swiftly to global health crises.
Environmental Science and Applied Research: Ocean Cleanup
Example: The Ocean Cleanup Project, founded by Boyan Slat, is an ambitious endeavor rooted in applied research to combat plastic pollution in the world's oceans.
This project employs innovative technology, such as large-scale floating barriers and autonomous systems, to collect and remove plastic debris from the ocean. Applied researchers have played a pivotal role in designing, testing, and optimizing these systems to make them efficient and environmentally friendly.
Impact: The Ocean Cleanup Project is a testament to the power of applied research in addressing pressing environmental challenges. By removing plastic waste from the oceans, it mitigates harm to marine ecosystems and raises awareness about the urgent need for sustainable waste management.
Business and Applied Research: E-commerce Personalization
Example: E-commerce giants like Amazon and Netflix use applied research to develop sophisticated personalization algorithms that tailor product recommendations and content to individual users.
Applied researchers in data science and machine learning analyze user behavior, preferences, and historical data to create recommendation systems. These algorithms utilize predictive analytics to suggest products, movies, or shows that align with a user's interests.
Impact: The application of research-driven personalization has transformed the e-commerce and streaming industries. It enhances user experiences, increases customer engagement, and drives sales by presenting customers with products or content they are more likely to enjoy.
Education and Applied Research: Flipped Classroom Model
Example: The Flipped Classroom Model is an applied research-based teaching approach that has gained popularity in education.
In this model, instructors leverage technology to deliver instructional content (such as video lectures) outside of class, allowing in-class time for active learning, discussions, and problem-solving. Applied research has informed the design and implementation of this pedagogical approach.
Impact: The Flipped Classroom Model has shown promise in enhancing student engagement and learning outcomes. It capitalizes on research findings about how students learn best, emphasizing active participation and collaborative learning.
Agriculture and Applied Research: Precision Agriculture
Example: Precision agriculture employs data-driven technology and applied research to optimize farming practices.
Farmers utilize satellite imagery, sensors, and data analytics to monitor crop conditions, soil health, and weather patterns. Applied research guides the development of precision farming techniques, enabling more efficient resource allocation and reducing environmental impact.
Impact: Precision agriculture increases crop yields, conserves resources (such as water and fertilizer), and minimizes the ecological footprint of farming. This approach contributes to sustainable and economically viable agriculture.
These real-world examples underscore the versatility and impact of applied research across diverse domains. From healthcare and environmental conservation to business, education, and agriculture, applied research continually drives innovation, addresses critical challenges, and enhances the quality of life for individuals and communities worldwide.
Conclusion for Applied Research
Applied research is a powerful force for solving real-world problems and driving progress. By applying existing knowledge and innovative thinking, we can address healthcare challenges, protect our environment, improve businesses, enhance education, and revolutionize agriculture. Through this guide, you've gained valuable insights into the what, why, and how of applied research, unlocking the potential to make a positive impact in your field. So, go forth, conduct meaningful research, and be part of the solution to the world's most pressing issues. Remember, applied research is not just a concept; it's a practical approach that empowers individuals and teams to create solutions that matter. As you embark on your own applied research endeavors, keep the spirit of inquiry alive, remain open to new ideas, and never underestimate the transformative power of knowledge put into action.
How to Conduct Applied Research in Minutes?
Appinio , a real-time market research platform, is here to revolutionize your approach to applied research. Imagine having the power to get real-time consumer insights at your fingertips, enabling you to make swift, data-driven decisions for your business. Appinio takes care of all the heavy lifting in research and tech, so you can focus on what truly matters.
- Lightning-Speed Insights: From posing questions to gaining insights, it takes mere minutes. When you need answers fast, Appinio delivers.
- User-Friendly: No need for a PhD in research; our platform is so intuitive that anyone can use it effectively.
- Global Reach: Access a diverse pool of respondents from over 90 countries, with the ability to define the perfect target group using 1200+ characteristics.
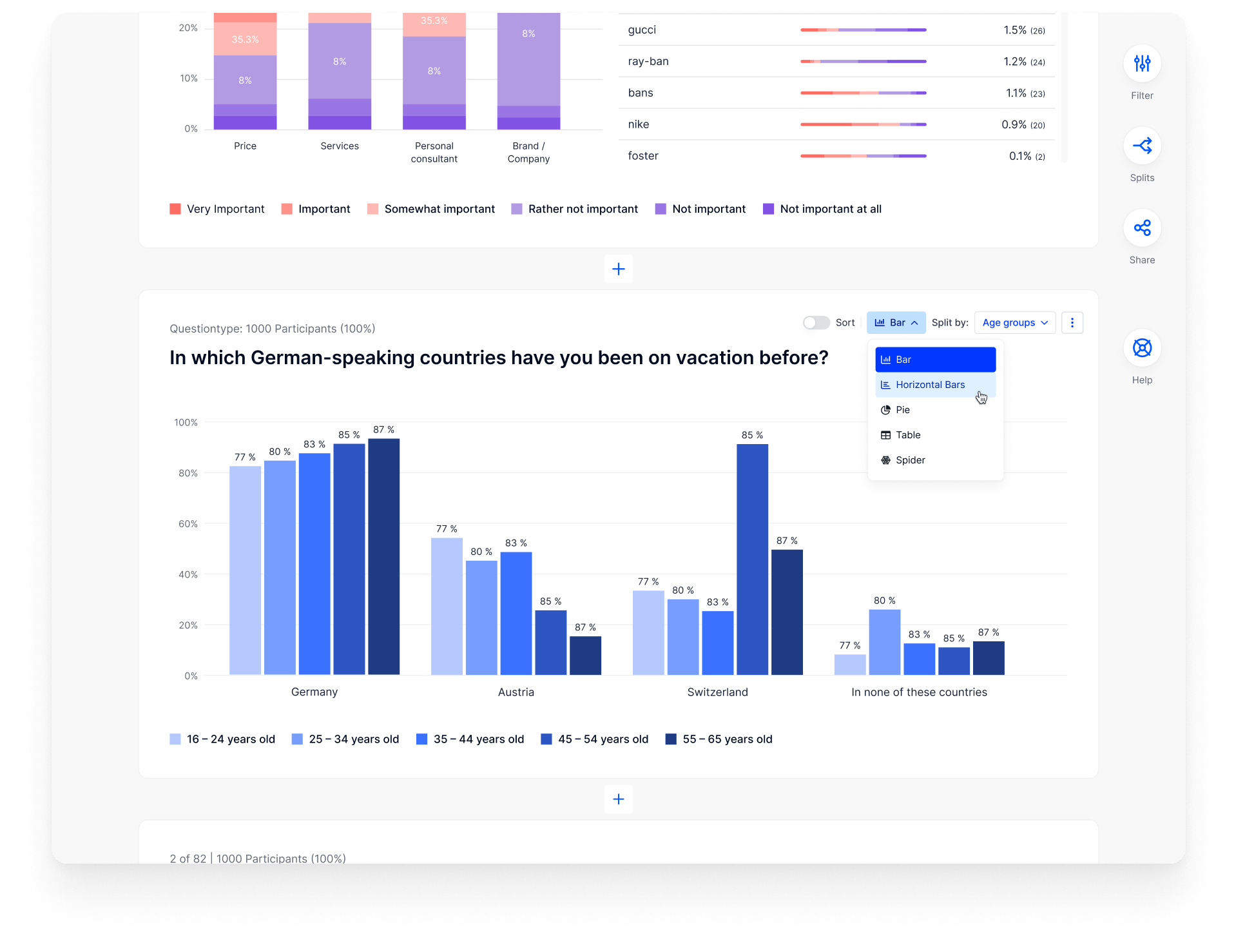
Get free access to the platform!
Join the loop 💌
Be the first to hear about new updates, product news, and data insights. We'll send it all straight to your inbox.
Get the latest market research news straight to your inbox! 💌
Wait, there's more
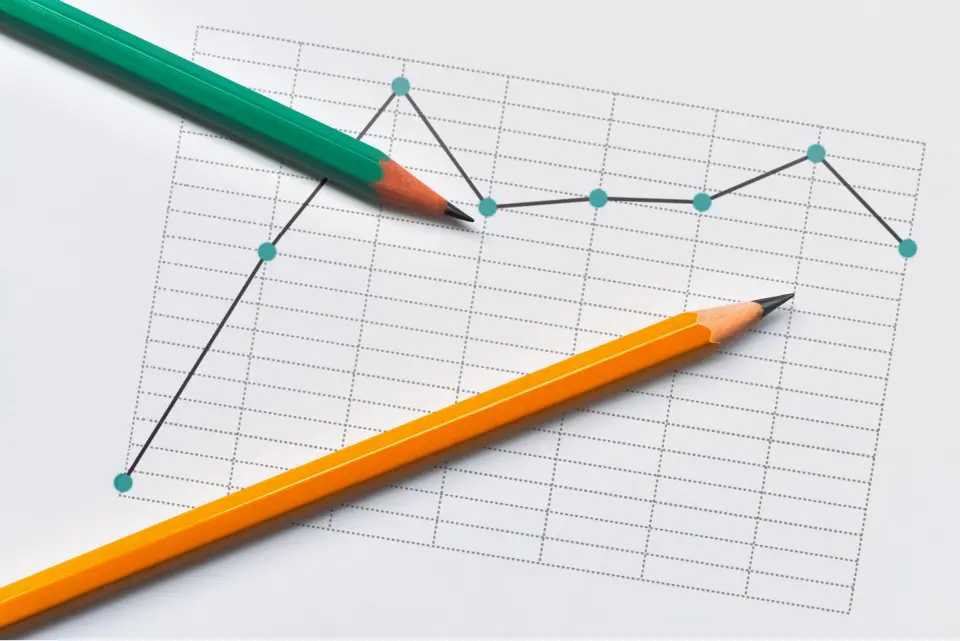
16.05.2024 | 30min read
Time Series Analysis: Definition, Types, Techniques, Examples
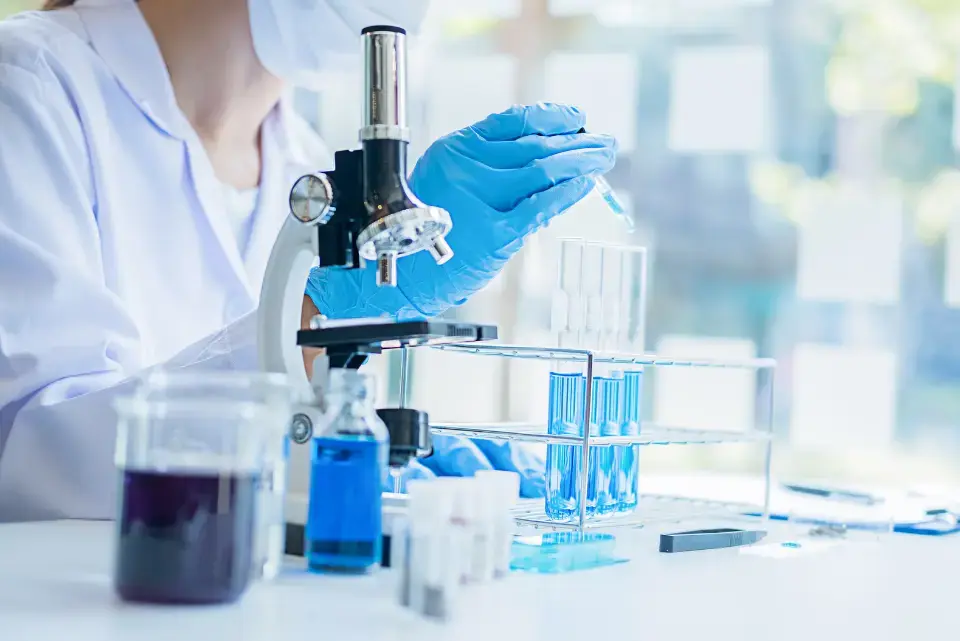
14.05.2024 | 31min read
Experimental Research: Definition, Types, Design, Examples
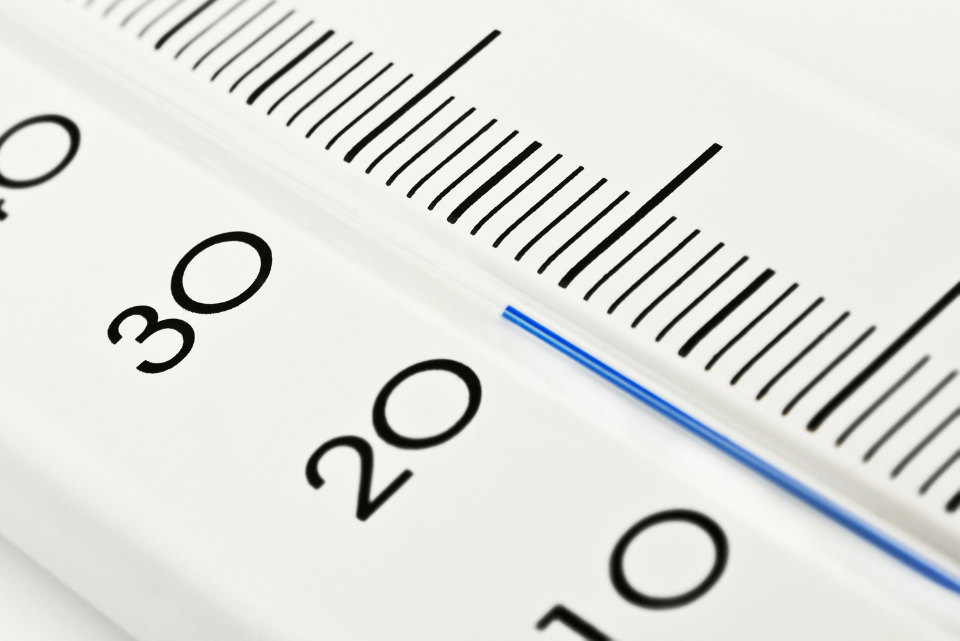
07.05.2024 | 29min read
Interval Scale: Definition, Characteristics, Examples
- What is Applied Research? + [Types, Examples & Method]

Every research begins with a clear delineation of the purpose of the investigation as this goes a long way to determine the research process or methodology adopted. In this sense, a researcher may choose to carry out basic research or applied research.
Applied research is set on providing answers to specific questions in a bid to provide a solution to a defined problem. In this article, we will outline the features of this method of systematic investigation as well as how it differs from other approaches to research.
What is Applied Research?
Applied research is a type of research design that seeks to solve a specific problem or provide innovative solutions to issues affecting an individual, group or society. It is often referred to as a scientific method of inquiry or contractual research because it involves the practical application of scientific methods to everyday problems.
When conducting applied research, the researcher takes extra care to identify a problem, develop a research hypothesis and goes ahead to test these hypotheses via an experiment. In many cases, this research approach employs empirical methods in order to solve practical problems.
Applied research is sometimes considered to be a non-systematic inquiry because of its direct approach in seeking a solution to a problem. It is typically a follow-up research design that further investigates the findings of pure or basic research in order to validate these findings and apply them to create innovative solutions.
Types of Applied Research
There are 3 types of applied research. These are evaluation research, research and development, and action research.
- Evaluation Research
Evaluation research is a type of applied research that analyses existing information about a research subject to arrive at objective research outcomes or reach informed decisions. This type of applied research is mostly applied in business contexts, for example, an organisation may adopt evaluation research to determine how to cut down overhead costs.
- Research and Development
Research and development is a type of applied research that is focused on developing new products and services based on the needs of target markets. It focuses on gathering information about marketing needs and finding ways to improve on an existing product or create new products that satisfy the identified needs.
- Action Research
Action research is a type of applied research that is set on providing practical solutions to specific business problems by pointing the business in the right directions. Typically, action research is a process of reflective inquiry that is limited to specific contexts and situational in nature.
Examples of Applied Research
Applied research is relevant in different fields of study; especially science and social science-related fields. Examples of applied research can be seen in medicine, education, business, engineering, psychology and health, and these would be further explicated below.
Applied Research Example in Business
Applied research is used in business to build knowledge and develop product solutions. It enables organisations to identify the peculiar needs of target markets and this would help them to create different business strategies that would allow them to satisfy these needs.
In addition, conducting contractual research would help business owners to get insightful feedback on product gaps that may have, otherwise, been ignored. This is a great way to get first-hand information on target market reactions which can inform brand decisions.
Applied research also helps employers of labour to identify and address the productivity needs of their workforce. For instance, an organization may carry out applied research in order to measure the effectiveness of its recruitment practices or of its organisational structure.
- Applied research to improve an organization’s hiring process.
- Applied research to improve workplace efficiency and organizational policies.
- Applied research to bridge skill gaps in the workplace.
Applied Research Examples in Education
In education, applied research is used to test pedagogic processes in order to discover the best teaching and learning methods. It is also used to test educational policies before implementation and to address different issues associated with teaching paradigms and classroom dynamics for a better learning experience.
Educational applied research attempts solving a problem by gathering data from primary sources using a combination of qualitative and quantitative data collection methods. This data serves as empirical evidence which is then subjected to rigorous analysis and description in order to arrive at valid conclusions.
The goal of this research methodology is to determine the applicability of educational theory and principles by way of subjecting hypotheses to experimentation within specific settings. Applied research in education is also more utilitarian as it gathers practical evidence that can inform pragmatic solutions to problems.
Characteristics of Applied Research in Education
- It clearly highlights generalizations and hypotheses that inform the research findings.
- It relies on empirical evidence.
- It is set at providing solutions to a defined problem.
- It requires accurate observation and description.
- A study into the way to improve teacher-learner classroom engagements.
- A study into the way to improve a school’s readiness for its students.
- A study to build students’ interests in Mathematics.
Applied Research Example in Science
In itself, applied research is a scientific method of investigation because it applies existing scientific knowledge to practical situations. It is useful in different fields including thermodynamics, physics, material sciences and microbiology.
Examples of applied research in science include the following:
- Applied research to improve agricultural crop production
- Applied research to treat or cure a specific disease.
Applied Research Examples in Psychology
There are different reasons psychologists would make use of applied research in the course of their work. In many cases, industrial-psychologists concerned with workplace behavior, human resources and organisational development combine psychological principles with applied research to proffer solutions.
Examples of applied research in psychology include:
- Applied research to improve workplace commitment by arriving at practical worker-motivation strategies.
- Investigating treatment and management options for anxiety and panic attacks.
- Investigating factors that improve worker’s productivity.
Applied Research Example in Health
In health and medical sciences, applied research serves as the background to evidence-based and solution-oriented medicine. It effectively merges scientific knowledge and methods with health experiences in order to arrive at accurate and verifiable results; using empirical research data or evidence.
The adaptation of applied research to medicine is referred to as applied clinical research . Many health and medical practitioners use applied research to measure the extent to which the findings of basic or pure research can be adopted or modified into a solution-oriented approach.
Examples of applied research in health include:
- An investigation to identify the healing properties of a specific herb.
- An investigation to identify the side effects of using a particular drug.
APPLIED RESEARCH METHODS
Qualitative and quantitative data collection methods are used in applied research to gather empirical evidence that is further subjected to experimentation in order to arrive at valid research outcomes. The following are data collection methods in applied research:
An interview is a qualitative method of data collection that involves having a one-on-one interaction or discussion with the research subjects in order to gather relevant information that can serve as empirical data. It can be conducted with the use of an audio recorder, digital camera or camcorder.
Even though it is time-consuming and expensive, interviews allow the researcher to gather the most relevant data which gives him or her in-depth knowledge about the research subjects. An interview may be structured, semi-structured or unstructured; depending on the research purpose.
- Surveys/Questionnaires
A questionnaire is an instrument that is typically used for quantitative data gathering . It outlines a series of questions relating to the research context and requires the research subjects to choose or provide responses that reflect their knowledge and experiences.
There are different types of questions that can be contained in a questionnaire including rating scale question s, close and open-ended questions and fixed alternatives. You can create and administer your applied research survey using data-collection platforms like Formplus .
You can also start choosing from our over 200 online survey/questionnaire templates.
Here is a step-by-step guide on how to create and administer questionnaires for applied research using Formplus
Sign in to Formplus
In the Formplus builder, you can easily create different questionnaires for applied research by dragging and dropping preferred fields into your form. To access the Formplus builder, you will need to create an account on Formplus.
Once you do this, sign in to your account and click on “Create Form ” to begin.
Edit Form Title
Click on the field provided to input your form title, for example, “Applied Research Questionnaire”.
Click on the edit button to edit the form.
i. Add Fields: Drag and drop preferred form fields into your form in the Formplus builder inputs column. There are several field input options for questionnaires in the Formplus builder.
ii. Edit fields
iii. Click on “Save”
iv. Preview form.
Form Customization
With the form customization options in the form builder, you can easily improve on the appearance of your questionnaire and make it more unique and personalized. Formplus allows you to change your form theme, add background images and even change the font according to your needs.
Multiple Sharing Options
Formplus also provides multiple form sharing options which enables you to easily share your questionnaire with respondents. With the direct social media sharing buttons, you can swiftly share your applied research questionnaire link to your organization’s social media pages.
You can send out your questionnaire as email invitations to your research subjects too. Formplus also allows you to share your form’s QR code or embed it in your organization’s website for easy access.
- Data Reporting
The process of gathering useful information about a research subject which can be used for further research. This can be done through not-for-profit reports, newspapers, website articles and hospital records.
It helps you gather relevant data that results in more insightful decisions. However, it is susceptible to bias because the information can easily be exaggerated by the individual or group collecting the data.
- Observation
A type of data gathering method in applied research that requires the researcher to pay close attention to a subject (s) in order to gather useful information about it. Although bias may arise with this method, observation is widely considered as a universally accepted research practice.
Observation helps the researcher to gather empirical data and thus, it is the starting point for the formulation of a hypothesis. There are different techniques for observation including complete observer, complete participant, participant as observer and observer as participant.
- Focus Groups
A focus group is a type of qualitative data collection process that allows the researcher to gather information about the disposition, feelings and opinions of the research subjects about a specific issue.
Here, the researcher engages a group comprising 6-10 individuals with a range of open-ended questions with the aim of gathering feedback about their emotional disposition to the issue at hand. This method is cost-effective compared to one-on-one interviews, and the information obtained is insightful and detailed.
How is Applied Research Different from Basic Research?
Applied research and basic research are common methods of inquiry, based on purpose or utility. However, there are key differences between these 2 research approaches and these would be clearly outlined below:
Applied research is a type of research that is aimed at the practical application of science in order to solve practical problems. On the other hand, basic research is a type of research that is aimed at expanding knowledge rather than solving problems.
Basic research is theoretical in nature while applied research is practical and descriptive in nature. Basic research explores and generates theories that may be abstract while applied research tests these theories in order to solve a problem.
Basic research is universal while applied research is limited. Basic research can focus on diverse or multiple contexts while applied research focuses on specific contexts with the aim of providing a solution to an identified problem.
Applied research is focused on providing answers or solutions to a specific research question while basic research focuses on multiple concepts at the same time in its quest to expand knowledge.
- Applied research pays attention to external validity while basic research is more focused on internal validity .
Characteristics of Applied Research
- Applied research is solution-specific and it addresses practical problems. Unlike basic research that is aimed at theorizing and expanding knowledge, applied research focuses on addressing a particular problem using a range of science-based approaches.
- Applied research is descriptive in nature as it arrives at solutions by experimenting on empirical evidence and describing research outcomes.
- Usually, applied research tests theories arrived at by pure research in order to determine the usefulness of these theories in solving practical problems.
- It describes the relationship between research variables by measuring the characteristics of dependent and independent variables.
- Applied research relies on empirical evidence in order to arrive at valid research outcomes.
- It is not theoretical and it is not directly concerned with the expansion of knowledge.
- Applied research is synthetic in nature.
- It is aimed at the cost-effective reduction of social problems.
- Applied research is action-oriented.
Advantages of Applied Research
- Validity: Applied research is unbiased in nature because it tests empirical evidence in order to arrive at valid research outcomes. It employs carefully mapped-out procedures, and this makes it a more valid research approach.
- It is useful in solving specific problems. It helps individuals and organizations to find solutions to specific problems.
Disadvantages of Applied Research
- It is not flexible in nature as it is restricted to a stipulated deadline.
- Applied research is limited in nature and it cannot be generalized. In other words, the findings from applied research cannot be generalized.
Conclusion
Applied research is an important research approach because it helps organisations to arrive at practical solutions to specific problems while improving their productivity and output. Unlike basic research that focuses on generating theories that explain phenomena, applied research pays attention to describing empirical evidence with the aim of providing solutions.
In carrying out applied research, the researcher combines a number of qualitative and quantitative data-gathering methods including questionnaires, observation methods, and interviews. This helps the researcher to gather empirical evidence that is then subjected to experimentation depending on the type of applied research and the overall focus.
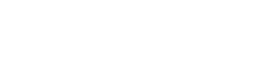
Connect to Formplus, Get Started Now - It's Free!
- applied basic research differences
- Applied research
- applied research characteristics
- Applied research methods
- applied research types
- examples of applied research
- types of applied research
- busayo.longe
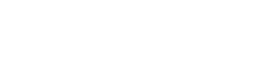
You may also like:
What is Pure or Basic Research? + [Examples & Method]
Simple guide on pure or basic research, its methods, characteristics, advantages, and examples in science, medicine, education and psychology

Basic vs Applied Research: 15 Key Differences
Differences between basic and applied research in definition, advantages, methods, types and examples
How to Write a Problem Statement for your Research
Learn how to write problem statements before commencing any research effort. Learn about its structure and explore examples
21 Chrome Extensions for Academic Researchers in 2022
In this article, we will discuss a number of chrome extensions you can use to make your research process even seamless
Formplus - For Seamless Data Collection
Collect data the right way with a versatile data collection tool. try formplus and transform your work productivity today..
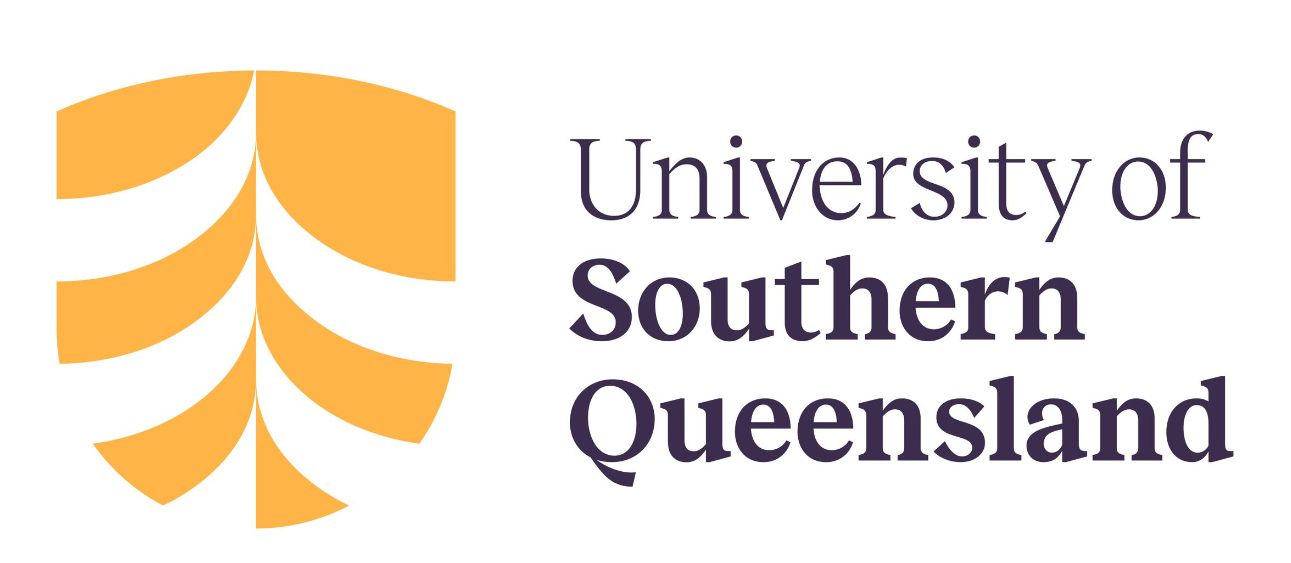
Want to create or adapt books like this? Learn more about how Pressbooks supports open publishing practices.
1 Science and scientific research
What is research? Depending on who you ask, you will likely get very different answers to this seemingly innocuous question. Some people will say that they routinely research different online websites to find the best place to buy the goods or services they want. Television news channels supposedly conduct research in the form of viewer polls on topics of public interest such as forthcoming elections or government-funded projects. Undergraduate students research on the Internet to find the information they need to complete assigned projects or term papers. Postgraduate students working on research projects for a professor may see research as collecting or analysing data related to their project. Businesses and consultants research different potential solutions to remedy organisational problems such as a supply chain bottleneck or to identify customer purchase patterns. However, none of the above can be considered ‘scientific research’ unless: it contributes to a body of science, and it follows the scientific method. This chapter will examine what these terms mean.
What is science? To some, science refers to difficult high school or university-level courses such as physics, chemistry, and biology meant only for the brightest students. To others, science is a craft practiced by scientists in white coats using specialised equipment in their laboratories. Etymologically, the word ‘science’ is derived from the Latin word scientia meaning knowledge. Science refers to a systematic and organised body of knowledge in any area of inquiry that is acquired using ‘the scientific method’ (the scientific method is described further below). Science can be grouped into two broad categories: natural science and social science. Natural science is the science of naturally occurring objects or phenomena, such as light, objects, matter, earth, celestial bodies, or the human body. Natural sciences can be further classified into physical sciences, earth sciences, life sciences, and others. Physical sciences consist of disciplines such as physics (the science of physical objects), chemistry (the science of matter), and astronomy (the science of celestial objects). Earth sciences consist of disciplines such as geology (the science of the earth). Life sciences include disciplines such as biology (the science of human bodies) and botany (the science of plants). In contrast, social science is the science of people or collections of people, such as groups, firms, societies, or economies, and their individual or collective behaviours. Social sciences can be classified into disciplines such as psychology (the science of human behaviours), sociology (the science of social groups), and economics (the science of firms, markets, and economies).
The natural sciences are different from the social sciences in several respects. The natural sciences are very precise, accurate, deterministic, and independent of the person making the scientific observations. For instance, a scientific experiment in physics, such as measuring the speed of sound through a certain media or the refractive index of water, should always yield the exact same results, irrespective of the time or place of the experiment, or the person conducting the experiment. If two students conducting the same physics experiment obtain two different values of these physical properties, then it generally means that one or both of those students must be in error. However, the same cannot be said for the social sciences, which tend to be less accurate, deterministic, or unambiguous. For instance, if you measure a person’s happiness using a hypothetical instrument, you may find that the same person is more happy or less happy (or sad) on different days and sometimes, at different times on the same day. One’s happiness may vary depending on the news that person received that day or on the events that transpired earlier during that day. Furthermore, there is not a single instrument or metric that can accurately measure a person’s happiness. Hence, one instrument may calibrate a person as being ‘more happy’ while a second instrument may find that the same person is ‘less happy’ at the same instant in time. In other words, there is a high degree of measurement error in the social sciences and there is considerable uncertainty and little agreement on social science policy decisions. For instance, you will not find many disagreements among natural scientists on the speed of light or the speed of the earth around the sun, but you will find numerous disagreements among social scientists on how to solve a social problem such as reduce global terrorism or rescue an economy from a recession. Any student studying the social sciences must be cognisant of and comfortable with handling higher levels of ambiguity, uncertainty, and error that come with such sciences, which merely reflects the high variability of social objects.
Sciences can also be classified based on their purpose. Basic sciences , also called pure sciences, are those that explain the most basic objects and forces, relationships between them, and laws governing them. Examples include physics, mathematics, and biology. Applied sciences , also called practical sciences, are sciences that apply scientific knowledge from basic sciences in a physical environment. For instance, engineering is an applied science that applies the laws of physics and chemistry for practical applications such as building stronger bridges or fuel efficient combustion engines, while medicine is an applied science that applies the laws of biology to solving human ailments. Both basic and applied sciences are required for human development. However, applied science cannot stand on its own right, but instead relies on basic sciences for its progress. Of course, industry and private enterprises tend to focus more on applied sciences given their practical value, while universities study both basic and applied sciences.
Scientific knowledge
The purpose of science is to create scientific knowledge. Scientific knowledge refers to a generalised body of laws and theories for explaining a phenomenon or behaviour of interest that is acquired using the scientific method. Laws are observed patterns of phenomena or behaviours, while theories are systematic explanations of the underlying phenomenon or behaviour. For instance, in physics, the Newtonian Laws of Motion describe what happens when an object is in a state of rest or motion (Newton’s First Law), what force is needed to move a stationary object or stop a moving object (Newton’s Second Law), and what happens when two objects collide (Newton’s Third Law). Collectively, the three laws constitute the basis of classical mechanics—a theory of moving objects. Likewise, the theory of optics explains the properties of light and how it behaves in different media, electromagnetic theory explains the properties of electricity and how to generate it, quantum mechanics explains the properties of subatomic particles, and thermodynamics explains the properties of energy and mechanical work. An introductory university level textbook in physics will likely contain separate chapters devoted to each of these theories. Similar theories are also available in social sciences. For instance, cognitive dissonance theory in psychology explains how people react when their observations of an event are different from what they expected of that event, general deterrence theory explains why some people engage in improper or criminal behaviours, such as to illegally download music or commit software piracy, and the theory of planned behaviour explains how people make conscious reasoned choices in their everyday lives.
The goal of scientific research is to discover laws and postulate theories that can explain natural or social phenomena, or in other words, build scientific knowledge. It is important to understand that this knowledge may be imperfect or even quite far from the truth. Sometimes, there may not be a single universal truth, but rather an equilibrium of ‘multiple truths.’ We must understand that the theories upon which scientific knowledge is based are only explanations of a particular phenomenon as suggested by a scientist. As such, there may be good or poor explanations depending on the extent to which those explanations fit well with reality, and consequently, there may be good or poor theories. The progress of science is marked by our progression over time from poorer theories to better theories, through better observations using more accurate instruments and more informed logical reasoning.
We arrive at scientific laws or theories through a process of logic and evidence. Logic (theory) and evidence (observations) are the two, and only two, pillars upon which scientific knowledge is based. In science, theories and observations are inter-related and cannot exist without each other. Theories provide meaning and significance to what we observe, and observations help validate or refine existing theory or construct new theory. Any other means of knowledge acquisition, such as faith or authority cannot be considered science.
Scientific research
Given that theories and observations are the two pillars of science, scientific research operates at two levels: a theoretical level and an empirical level. The theoretical level is concerned with developing abstract concepts about a natural or social phenomenon and relationships between those concepts (i.e., build ‘theories’), while the empirical level is concerned with testing the theoretical concepts and relationships to see how well they reflect our observations of reality, with the goal of ultimately building better theories. Over time, a theory becomes more and more refined (i.e., fits the observed reality better), and the science gains maturity. Scientific research involves continually moving back and forth between theory and observations. Both theory and observations are essential components of scientific research. For instance, relying solely on observations for making inferences and ignoring theory is not considered valid scientific research.
Depending on a researcher’s training and interest, scientific inquiry may take one of two possible forms: inductive or deductive. In inductive research , the goal of a researcher is to infer theoretical concepts and patterns from observed data. In deductive research , the goal of the researcher is to test concepts and patterns known from theory using new empirical data. Hence, inductive research is also called theory-building research, and deductive research is theory-testing research. Note here that the goal of theory testing is not just to test a theory, but possibly to refine, improve, and extend it. Figure 1.1 depicts the complementary nature of inductive and deductive research. Note that inductive and deductive research are two halves of the research cycle that constantly iterates between theory and observations. You cannot do inductive or deductive research if you are not familiar with both the theory and data components of research. Naturally, a complete researcher is one who can traverse the entire research cycle and can handle both inductive and deductive research.
It is important to understand that theory-building (inductive research) and theory-testing (deductive research) are both critical for the advancement of science. Elegant theories are not valuable if they do not match with reality. Likewise, mountains of data are also useless until they can contribute to the construction of meaningful theories. Rather than viewing these two processes in a circular relationship, as shown in Figure 1.1, perhaps they can be better viewed as a helix, with each iteration between theory and data contributing to better explanations of the phenomenon of interest and better theories. Though both inductive and deductive research are important for the advancement of science, it appears that inductive (theory-building) research is more valuable when there are few prior theories or explanations, while deductive (theory-testing) research is more productive when there are many competing theories of the same phenomenon and researchers are interested in knowing which theory works best and under what circumstances.
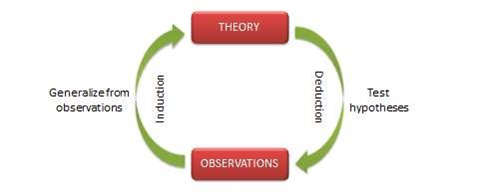
Theory building and theory testing are particularly difficult in the social sciences, given the imprecise nature of the theoretical concepts, inadequate tools to measure them, and the presence of many unaccounted for factors that can also influence the phenomenon of interest. It is also very difficult to refute theories that do not work. For instance, Karl Marx’s theory of communism as an effective means of economic production withstood for decades, before it was finally discredited as being inferior to capitalism in promoting economic growth and social welfare. Erstwhile communist economies like the Soviet Union and China eventually moved toward more capitalistic economies characterised by profit-maximising private enterprises. However, the recent collapse of the mortgage and financial industries in the United States demonstrates that capitalism also has its flaws and is not as effective in fostering economic growth and social welfare as previously presumed. Unlike theories in the natural sciences, social science theories are rarely perfect, which provides numerous opportunities for researchers to improve those theories or build their own alternative theories.
Conducting scientific research, therefore, requires two sets of skills—theoretical and methodological—needed to operate in the theoretical and empirical levels respectively. Methodological skills (‘know-how’) are relatively standard, invariant across disciplines, and easily acquired through doctoral programs. However, theoretical skills (‘know-what’) are considerably harder to master, require years of observation and reflection, and are tacit skills that cannot be ‘taught’ but rather learned though experience. All of the greatest scientists in the history of mankind, such as Galileo, Newton, Einstein, Niels Bohr, Adam Smith, Charles Darwin, and Herbert Simon, were master theoreticians, and they are remembered for the theories they postulated that transformed the course of science. Methodological skills are needed to be an ordinary researcher, but theoretical skills are needed to be an extraordinary researcher!
Scientific method
In the preceding sections, we described science as knowledge acquired through a scientific method. So what exactly is the ‘scientific method’? Scientific method refers to a standardised set of techniques for building scientific knowledge, such as how to make valid observations, how to interpret results, and how to generalise those results. The scientific method allows researchers to independently and impartially test pre-existing theories and prior findings, and subject them to open debate, modifications, or enhancements. The scientific method must satisfy four key characteristics:
Replicability : Others should be able to independently replicate or repeat a scientific study and obtain similar, if not identical, results. Precision : Theoretical concepts, which are often hard to measure, must be defined with such precision that others can use those definitions to measure those concepts and test that theory. Falsifiability : A theory must be stated in such a way that it can be disproven. Theories that cannot be tested or falsified are not scientific theories and any such knowledge is not scientific knowledge. A theory that is specified in imprecise terms or whose concepts are not accurately measureable cannot be tested, and is therefore not scientific. Sigmund Freud’s ideas on psychoanalysis fall into this category and are therefore not considered a ‘theory’, even though psychoanalysis may have practical utility in treating certain types of ailments. Parsimony: When there are multiple different explanations of a phenomenon, scientists must always accept the simplest or logically most economical explanation. This concept is called parsimony or ‘Occam’s razor’. Parsimony prevents scientists from pursuing overly complex or outlandish theories with an endless number of concepts and relationships that may explain a little bit of everything but nothing in particular. Any branch of inquiry that does not allow the scientific method to test its basic laws or theories cannot be called ‘science’. For instance, theology (the study of religion) is not science because theological ideas—such as the presence of God—cannot be tested by independent observers using a logical, confirmable, repeatable, and scrutinisable. Similarly, arts, music, literature, humanities, and law are also not considered science, even though they are creative and worthwhile endeavours in their own right.
The scientific method, as applied to social sciences, includes a variety of research approaches, tools, and techniques for collecting and analysing qualitative or quantitative data. These methods include laboratory experiments, field surveys, case research, ethnographic research, action research, and so forth. Much of this book is devoted to learning about these different methods. However, recognise that the scientific method operates primarily at the empirical level of research, i.e., how to make observations and analyse these observations. Very little of this method is directly pertinent to the theoretical level, which is really the more challenging part of scientific research.
Types of scientific research
Depending on the purpose of research, scientific research projects can be grouped into three types: exploratory, descriptive, and explanatory. Exploratory research is often conducted in new areas of inquiry, where the goals of the research are: to scope out the magnitude or extent of a particular phenomenon, problem, or behaviour, to generate some initial ideas (or ‘hunches’) about that phenomenon, or to test the feasibility of undertaking a more extensive study regarding that phenomenon. For instance, if the citizens of a country are generally dissatisfied with governmental policies during an economic recession, exploratory research may be directed at measuring the extent of citizens’ dissatisfaction, understanding how such dissatisfaction is manifested, such as the frequency of public protests, and the presumed causes of such dissatisfaction, such as ineffective government policies in dealing with inflation, interest rates, unemployment, or higher taxes. Such research may include examination of publicly reported figures, such as estimates of economic indicators, such as gross domestic product (GDP), unemployment, and consumer price index (CPI), as archived by third-party sources, obtained through interviews of experts, eminent economists, or key government officials, and/or derived from studying historical examples of dealing with similar problems. This research may not lead to a very accurate understanding of the target problem, but may be worthwhile in scoping out the nature and extent of the problem and serve as a useful precursor to more in-depth research.
Descriptive research is directed at making careful observations and detailed documentation of a phenomenon of interest. These observations must be based on the scientific method (i.e., must be replicable, precise, etc.), and therefore, are more reliable than casual observations by untrained people. Examples of descriptive research are tabulation of demographic statistics by the United States Census Bureau or employment statistics by the Bureau of Labor, who use the same or similar instruments for estimating employment by sector or population growth by ethnicity over multiple employment surveys or censuses. If any changes are made to the measuring instruments, estimates are provided with and without the changed instrumentation to allow the readers to make a fair before-and-after comparison regarding population or employment trends. Other descriptive research may include chronicling ethnographic reports of gang activities among adolescent youth in urban populations, the persistence or evolution of religious, cultural, or ethnic practices in select communities, and the role of technologies such as Twitter and instant messaging in the spread of democracy movements in Middle Eastern countries.
Explanatory research seeks explanations of observed phenomena, problems, or behaviours. While descriptive research examines the what, where, and when of a phenomenon, explanatory research seeks answers to questions of why and how. It attempts to ‘connect the dots’ in research, by identifying causal factors and outcomes of the target phenomenon. Examples include understanding the reasons behind adolescent crime or gang violence, with the goal of prescribing strategies to overcome such societal ailments. Most academic or doctoral research belongs to the explanation category, though some amount of exploratory and/or descriptive research may also be needed during initial phases of academic research. Seeking explanations for observed events requires strong theoretical and interpretation skills, along with intuition, insights, and personal experience. Those who can do it well are also the most prized scientists in their disciplines.
History of scientific thought
Before closing this chapter, it may be interesting to go back in history and see how science has evolved over time and identify the key scientific minds in this evolution. Although instances of scientific progress have been documented over many centuries, the terms ‘science’, ’scientists’, and the ‘scientific method’ were coined only in the nineteenth century. Prior to this time, science was viewed as a part of philosophy, and coexisted with other branches of philosophy such as logic, metaphysics, ethics, and aesthetics, although the boundaries between some of these branches were blurred.
In the earliest days of human inquiry, knowledge was usually recognised in terms of theological precepts based on faith. This was challenged by Greek philosophers such as Plato, Aristotle, and Socrates during the third century BC, who suggested that the fundamental nature of being and the world can be understood more accurately through a process of systematic logical reasoning called rationalism . In particular, Aristotle’s classic work Metaphysics (literally meaning ‘beyond physical [existence]’) separated theology (the study of Gods) from ontology (the study of being and existence) and universal science (the study of first principles, upon which logic is based). Rationalism (not to be confused with ‘rationality’) views reason as the source of knowledge or justification, and suggests that the criterion of truth is not sensory but rather intellectual and deductive, often derived from a set of first principles or axioms (such as Aristotle’s ‘law of non-contradiction’).
The next major shift in scientific thought occurred during the sixteenth century, when British philosopher Francis Bacon (1561–1626) suggested that knowledge can only be derived from observations in the real world. Based on this premise, Bacon emphasised knowledge acquisition as an empirical activity (rather than as a reasoning activity), and developed empiricism as an influential branch of philosophy. Bacon’s works led to the popularisation of inductive methods of scientific inquiry, the development of the ‘scientific method’ (originally called the ‘Baconian method’), consisting of systematic observation, measurement, and experimentation, and may have even sowed the seeds of atheism or the rejection of theological precepts as ‘unobservable’.
Empiricism continued to clash with rationalism throughout the Middle Ages, as philosophers sought the most effective way of gaining valid knowledge. French philosopher Rene Descartes sided with the rationalists, while British philosophers John Locke and David Hume sided with the empiricists. Other scientists, such as Galileo Galilei and Sir Isaac Newton, attempted to fuse the two ideas into natural philosophy (the philosophy of nature), to focus specifically on understanding nature and the physical universe, which is considered to be the precursor of the natural sciences. Galileo (1564–1642) was perhaps the first to state that the laws of nature are mathematical, and contributed to the field of astronomy through an innovative combination of experimentation and mathematics.
In the eighteenth century, German philosopher Immanuel Kant sought to resolve the dispute between empiricism and rationalism in his book Critique of pure r eason by arguing that experiences are purely subjective and processing them using pure reason without first delving into the subjective nature of experiences will lead to theoretical illusions. Kant’s ideas led to the development of German idealism , which inspired later development of interpretive techniques such as phenomenology, hermeneutics, and critical social theory.
At about the same time, French philosopher Auguste Comte (1798–1857), founder of the discipline of sociology, attempted to blend rationalism and empiricism in a new doctrine called positivism . He suggested that theory and observations have circular dependence on each other. While theories may be created via reasoning, they are only authentic if they can be verified through observations. The emphasis on verification started the separation of modern science from philosophy and metaphysics and further development of the ‘scientific method’ as the primary means of validating scientific claims. Comte’s ideas were expanded by Emile Durkheim in his development of sociological positivism (positivism as a foundation for social research) and Ludwig Wittgenstein in logical positivism.
In the early twentieth century, strong accounts of positivism were rejected by interpretive sociologists (antipositivists) belonging to the German idealism school of thought. Positivism was typically equated with quantitative research methods such as experiments and surveys and without any explicit philosophical commitments, while antipositivism employed qualitative methods such as unstructured interviews and participant observation. Even practitioners of positivism, such as American sociologist Paul Lazarsfield who pioneered large-scale survey research and statistical techniques for analysing survey data, acknowledged potential problems of observer bias and structural limitations in positivist inquiry. In response, antipositivists emphasised that social actions must be studied though interpretive means based upon understanding the meaning and purpose that individuals attach to their personal actions, which inspired Georg Simmel’s work on symbolic interactionism, Max Weber’s work on ideal types, and Edmund Husserl’s work on phenomenology.
In the mid-to-late twentieth century, both positivist and antipositivist schools of thought were subjected to criticisms and modifications. British philosopher Sir Karl Popper suggested that human knowledge is based not on unchallengeable, rock solid foundations, but rather on a set of tentative conjectures that can never be proven conclusively, but only disproven. Empirical evidence is the basis for disproving these conjectures or ‘theories’. This metatheoretical stance, called postpositivism (or postempiricism), amends positivism by suggesting that it is impossible to verify the truth although it is possible to reject false beliefs, though it retains the positivist notion of an objective truth and its emphasis on the scientific method.
Likewise, antipositivists have also been criticised for trying only to understand society but not critiquing and changing society for the better. The roots of this thought lie in Das k apital , written by German philosophers Karl Marx and Friedrich Engels, which critiqued capitalistic societies as being socially inequitable and inefficient, and recommended resolving this inequity through class conflict and proletarian revolutions. Marxism inspired social revolutions in countries such as Germany, Italy, Russia, and China, but generally failed to accomplish the social equality that it aspired. Critical research (also called critical theory) propounded by Max Horkheimer and Jürgen Habermas in the twentieth century, retains similar ideas of critiquing and resolving social inequality, and adds that people can and should consciously act to change their social and economic circumstances, although their ability to do so is constrained by various forms of social, cultural and political domination. Critical research attempts to uncover and critique the restrictive and alienating conditions of the status quo by analysing the oppositions, conflicts and contradictions in contemporary society, and seeks to eliminate the causes of alienation and domination (i.e., emancipate the oppressed class). More on these different research philosophies and approaches will be covered in future chapters of this book.
Social Science Research: Principles, Methods and Practices (Revised edition) Copyright © 2019 by Anol Bhattacherjee is licensed under a Creative Commons Attribution-NonCommercial-ShareAlike 4.0 International License , except where otherwise noted.
Share This Book
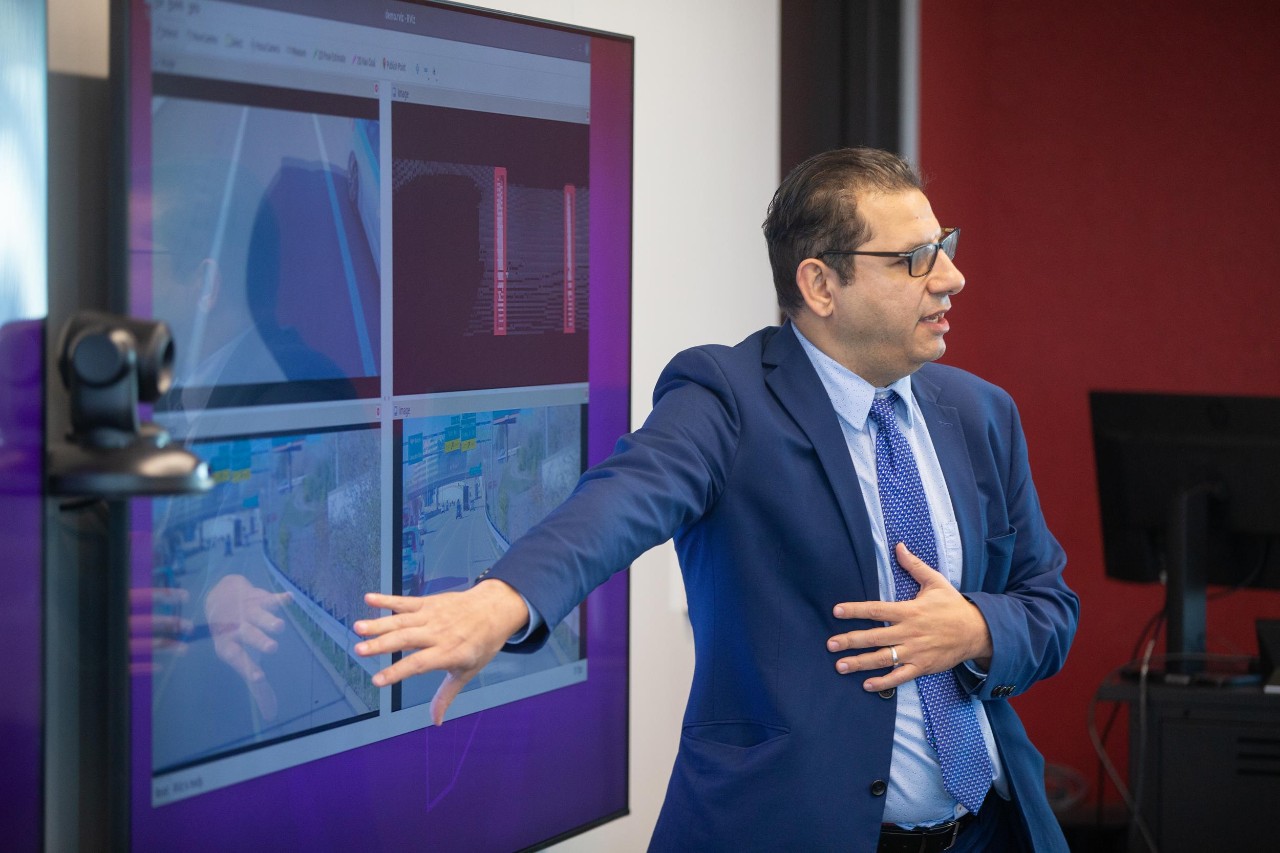
Engineering professor honored for distinguished scientific research
Civil engineer directs center for smart, sustainable & resilient infrastructure.
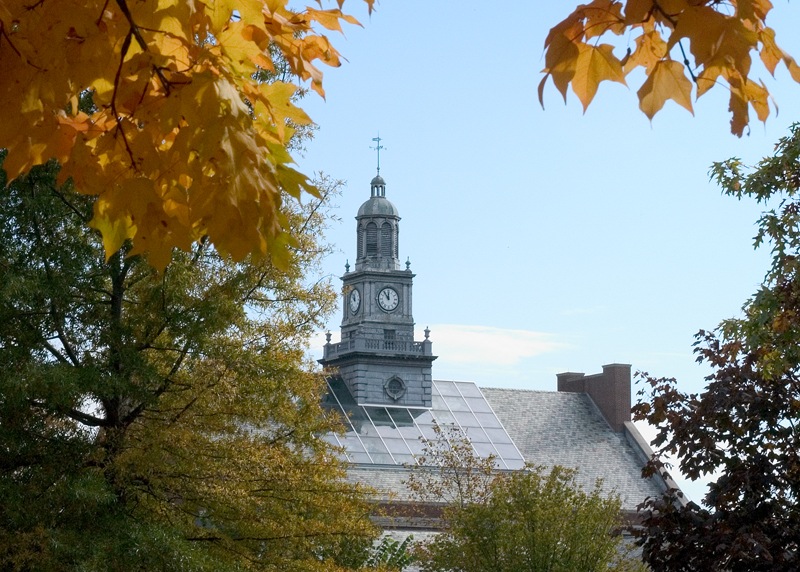
In 2019, Munir Nazzal , Ph.D., joined the University of Cincinnati College of Engineering and Applied Science as a professor in the Department of Civil and Architectural Engineering and Construction Management .
At UC, he has received various accolades for his advancements in transportation research, including the UC George Rieveschl Jr. Award for Distinguished Scientific Research for 2024.
Munir Nazzal, Civil Engineering Professor and Director of the Center for Smart, Sustainable, and Resilient Infrastructure. Photo/Andrew Higley/UC Marketing + Brand
In addition to teaching, Nazzal is director of UC's Center for Smart, Sustainable & Resilient Infrastructure .
He is co-author of more than 160 peer-reviewed publications supported by more than $14 million in research funding. His work focuses on novel methods and technologies to advance sustainable transportation-related infrastructure.
In 2023 the Association of State Highway and Transportation Officials selected his project for the High Value Research Supplemental Award.
“Dr. Nazzal’s work has a tremendous impact,” said Richard Miller , professor and head of UC's Department of Civil and Architectural Engineering and Construction Management.
“His work on studying infrastructure materials has also resulted in better, higher quality paving materials which is saving money, providing longer service life and improving the infrastructure construction industry. This work has the potential to have a huge impact on the ability of state departments of transportation to manage infrastructure.”
Nazzal and his team focus their research on the use of connected and autonomous (self-driving) vehicles for road assessments, with preparation of infrastructure for next-generation transportation. Recently, Nazzal developed an artificial intelligence system that uses connected and autonomous vehicle sensor data to assess damage in infrastructure assets, such as potholes.
Nazzal is partnering with Honda, the infrastructure engineering firm Parsons Corp., the consulting firm i-Probe and the Ohio Department of Transportation on the first project in the world to use sensors in production-level vehicles to examine assets of transportation infrastructure. The results of this project will have major impacts in the transportation sector, aiming to reduce costs, improve public safety by reducing accidents, and facilitate the safe, widespread deployment of connected and autonomous vehicles. The project improves roads by sensing things like where pavement markers are needed, where intersections need to be refined, and if signs are missing or obscured by the environment. To additionally support his research, Nazzal is also partnered with the Jurgensen Co., a local infrastructure construction firm.
Munir Nazzal with his family after receiving the 2024 George Rieveschl Jr. Award for Distinguished Scientific Research. Photo/Joseph Fuqua II/UC
Alongside his acclaimed research work, Nazzal is a member of Ohio State Transportation Invocation Council and is an editor for ASCE Journal of Materials in Civil Engineering and Buildings Journal. He continues to teach as a professor of civil engineering, and has mentored 35 graduate students.
“Dr. Nazzal’s work is of the highest quality, and it has a tremendous impact beyond Cincinnati,” said UC College of Engineering and Applied Science Dean John Weidner.
- Next Lives Here
The University of Cincinnati is classified as a Research 1 institution by the Carnegie Commission and is ranked in the National Science Foundation's Top-35 public research universities. UC's graduate students and faculty investigate problems and innovate solutions with real-world impact. Next Lives Here .
Featured image at top: Munir Nazzal presents his transporation research. Photo/Andrew Higley/UC Marketing + Brand
- Faculty Staff
- Office of Research
- College of Engineering and Applied Science
- Civil and Architectural Engineering and Construction Management
Related Stories
May 21, 2024
In 2019, Munir Nazzal joined the University of Cincinnati College of Engineering and Applied Science as a professor in the Department of Civil and Architectural Engineering and Construction Management. Throughout his time at UC, he has received various accolades for his advancements in transportation research, including the UC George Rieveschl Jr. Award for Distinguished Scientific Research for 2024.
How can your car make roads safer?
November 20, 2023
The University of Cincinnati will work with Honda Motor Co., infrastructure engineering firm Parsons Corp., consulting firm i-Probe and the Ohio Department of Transportation to demonstrate that new cars can help evaluate roads.
UC students take on net-zero building challenge
October 10, 2022
Students in architecture and engineering programs at the University of Cincinnati are learning about the latest materials and strategies to create more energy-efficient indoor spaces. They will take part in the Solar Decathlon to design the next generation of efficient buildings.
Gear-obsessed editors choose every product we review. We may earn commission if you buy from a link. Why Trust Us?
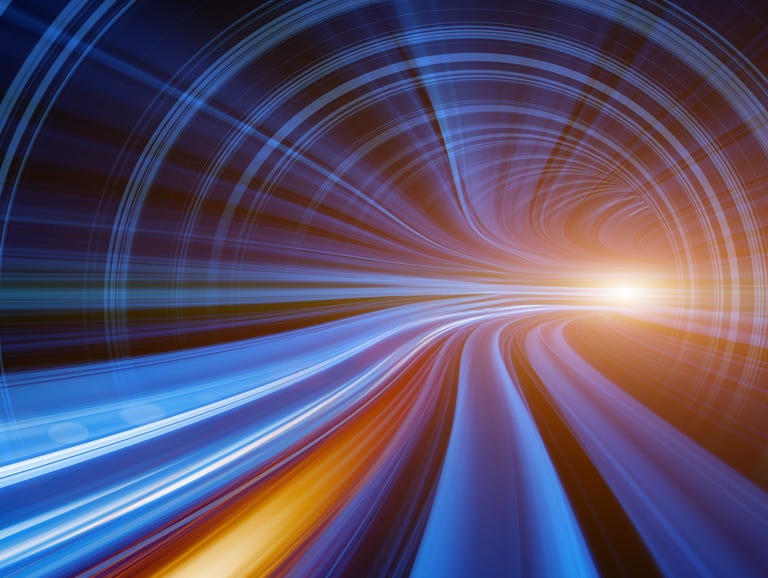
A Groundbreaking Scientific Discovery Just Created the Instruction Manual for Light-Speed Travel
In a first for warp drives, this research actually obeys the laws of physics.
If a superluminal—meaning faster than the speed of light—warp drive like Alcubierre’s worked, it would revolutionize humanity’s endeavors across the universe , allowing us, perhaps, to reach Alpha Centauri, our closest star system, in days or weeks even though it’s four light years away.
The clip above from the 2016 film Star Trek Beyond showcases the effect of a starship zipping through space inside a faster-than-light warp bubble. You can see the imagined but hypothetically accurate warping of spacetime.
However, the Alcubierre drive has a glaring problem: the force behind its operation, called “negative energy,” involves exotic particles—hypothetical matter that, as far as we know, doesn’t exist in our universe. Described only in mathematical terms, exotic particles act in unexpected ways, like having negative mass and working in opposition to gravity (in fact, it has “anti-gravity”). For the past 30 years, scientists have been publishing research that chips away at the inherent hurdles to light speed revealed in Alcubierre’s foundational 1994 article published in the peer-reviewed journal Classical and Quantum Gravity .
Now, researchers at the New York City-based think tank Applied Physics believe they’ve found a creative new approach to solving the warp drive’s fundamental roadblock. Along with colleagues from other institutions, the team envisioned a “positive energy” system that doesn’t violate the known laws of physics . It’s a game-changer, say two of the study’s authors: Gianni Martire, CEO of Applied Physics, and Jared Fuchs, Ph.D., a senior scientist there. Their work, also published in Classical and Quantum Gravity in late April, could be the first chapter in the manual for interstellar spaceflight.
Positive energy makes all the difference. Imagine you are an astronaut in space, pushing a tennis ball away from you. Instead of moving away, the ball pushes back, to the point that it would “take your hand off” if you applied enough pushing force, Martire tells Popular Mechanics . That’s a sign of negative energy, and, though the Alcubierre drive design requires it, there’s no way to harness it.
Instead, regular old positive energy is more feasible for constructing the “ warp bubble .” As its name suggests, it’s a spherical structure that surrounds and encloses space for a passenger ship using a shell of regular—but incredibly dense—matter. The bubble propels the spaceship using the powerful gravity of the shell, but without causing the passengers to feel any acceleration. “An elevator ride would be more eventful,” Martire says.
That’s because the density of the shell, as well as the pressure it exerts on the interior, is controlled carefully, Fuchs tells Popular Mechanics . Nothing can travel faster than the speed of light, according to the gravity-bound principles of Albert Einstein’s theory of general relativity . So the bubble is designed such that observers within their local spacetime environment—inside the bubble—experience normal movement in time. Simultaneously, the bubble itself compresses the spacetime in front of the ship and expands it behind the ship, ferrying itself and the contained craft incredibly fast. The walls of the bubble generate the necessary momentum, akin to the momentum of balls rolling, Fuchs explains. “It’s the movement of the matter in the walls that actually creates the effect for passengers on the inside.”
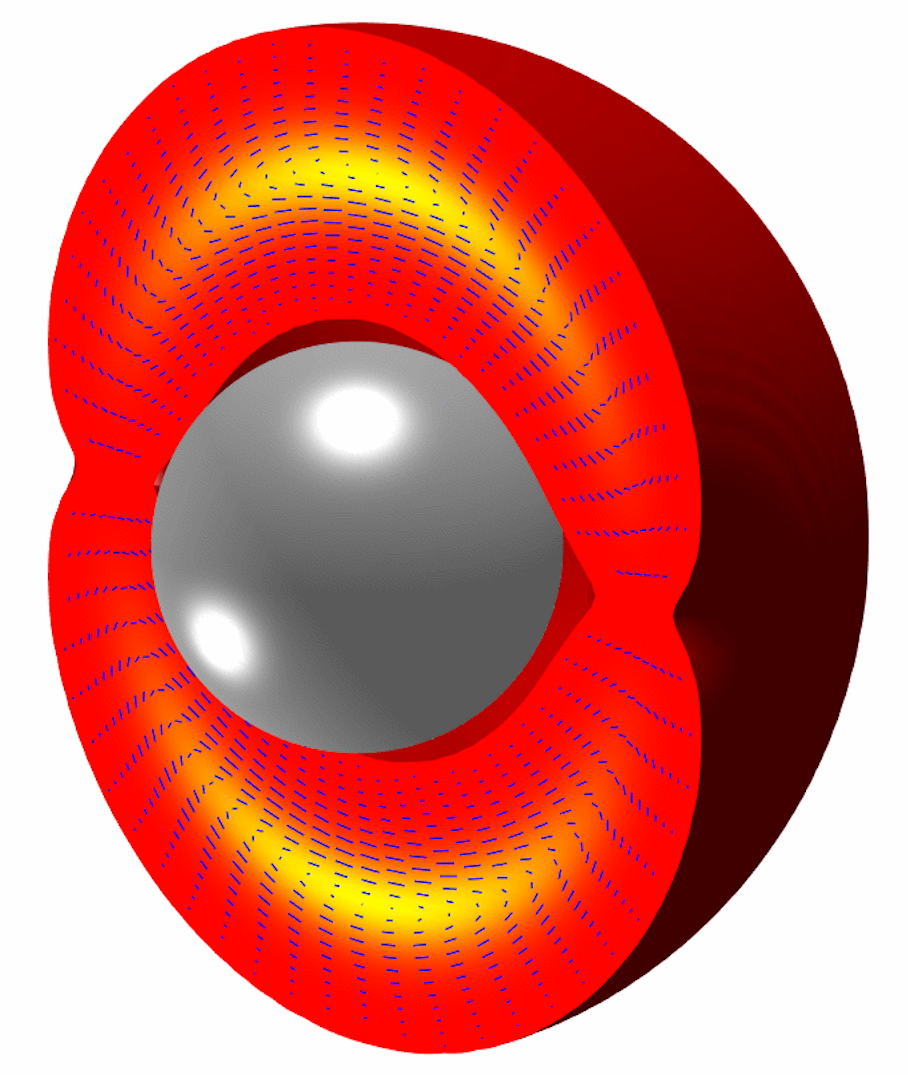
Building on its 2021 paper published in Classical and Quantum Gravity —which details the same researchers’ earlier work on physical warp drives—the team was able to model the complexity of the system using its own computational program, Warp Factory. This toolkit for modeling warp drive spacetimes allows researchers to evaluate Einstein’s field equations and compute the energy conditions required for various warp drive geometries. Anyone can download and use it for free . These experiments led to what Fuchs calls a mini model, the first general model of a positive-energy warp drive. Their past work also demonstrated that the amount of energy a warp bubble requires depends on the shape of the bubble; for example, the flatter the bubble in the direction of travel, the less energy it needs.
☄️ DID YOU KNOW? People have been imagining traveling as fast as light for nearly a century, if not longer. The 1931 novel Islands of Space by John W. Campbell mentions a “warp” method in the context of superluminal space travel.
This latest advancement suggests fresh possibilities for studying warp travel design, Erik Lentz, Ph.D., tells Popular Mechanics . In his current position as a staff physicist at Pacific Northwest National Laboratory in Richland, Washington, Lentz contributes to research on dark matter detection and quantum information science research. His independent research in warp drive theory also aims to be grounded in conventional physics while reimagining the shape of warped space. The topic needs to overcome many practical hurdles, he says.
Controlling warp bubbles requires a great deal of coordination because they involve enormous amounts of matter and energy to keep the passengers safe and with a similar passage of time as the destination. “We could just as well engineer spacetime where time passes much differently inside [the passenger compartment] than outside. We could miss our appointment at Proxima Centauri if we aren’t careful,” Lentz says. “That is still a risk if we are traveling less than the speed of light.” Communication between people inside the bubble and outside could also become distorted as it passes through the curvature of warped space, he adds.
While Applied Physics’ current solution requires a warp drive that travels below the speed of light, the model still needs to plug in a mass equivalent to about two Jupiters. Otherwise, it will never achieve the gravitational force and momentum high enough to cause a meaningful warp effect. But no one knows what the source of this mass could be—not yet, at least. Some research suggests that if we could somehow harness dark matter , we could use it for light-speed travel, but Fuchs and Martire are doubtful, since it’s currently a big mystery (and an exotic particle).
Despite the many problems scientists still need to solve to build a working warp drive, the Applied Physics team claims its model should eventually get closer to light speed. And even if a feasible model remains below the speed of light, it’s a vast improvement over today’s technology. For example, traveling at even half the speed of light to Alpha Centauri would take nine years. In stark contrast, our fastest spacecraft, Voyager 1—currently traveling at 38,000 miles per hour—would take 75,000 years to reach our closest neighboring star system.
Of course, as you approach the actual speed of light, things get truly weird, according to the principles of Einstein’s special relativity . The mass of an object moving faster and faster would increase infinitely, eventually requiring an infinite amount of energy to maintain its speed.
“That’s the chief limitation and key challenge we have to overcome—how can we have all this matter in our [bubble], but not at such a scale that we can never even put it together?” Martire says. It’s possible the answer lies in condensed matter physics, he adds. This branch of physics deals particularly with the forces between atoms and electrons in matter. It has already proven fundamental to several of our current technologies, such as transistors, solid-state lasers, and magnetic storage media.
The other big issue is that current models allow a stable warp bubble, but only for a constant velocity. Scientists still need to figure out how to design an initial acceleration. On the other end of the journey, how will the ship slow down and stop? “It’s like trying to grasp the automobile for the first time,” Martire says. “We don’t have an engine just yet, but we see the light at the end of the tunnel.” Warp drive technology is at the stage of 1882 car technology, he says: when automobile travel was possible, but it still looked like a hard, hard problem.
The Applied Physics team believes future innovations in warp travel are inevitable. The general positive energy model is a first step. Besides, you don’t need to zoom at light speed to achieve distances that today are just a dream, Martire says. “Humanity is officially, mathematically, on an interstellar track.”
Before joining Popular Mechanics , Manasee Wagh worked as a newspaper reporter, a science journalist, a tech writer, and a computer engineer. She’s always looking for ways to combine the three greatest joys in her life: science, travel, and food.
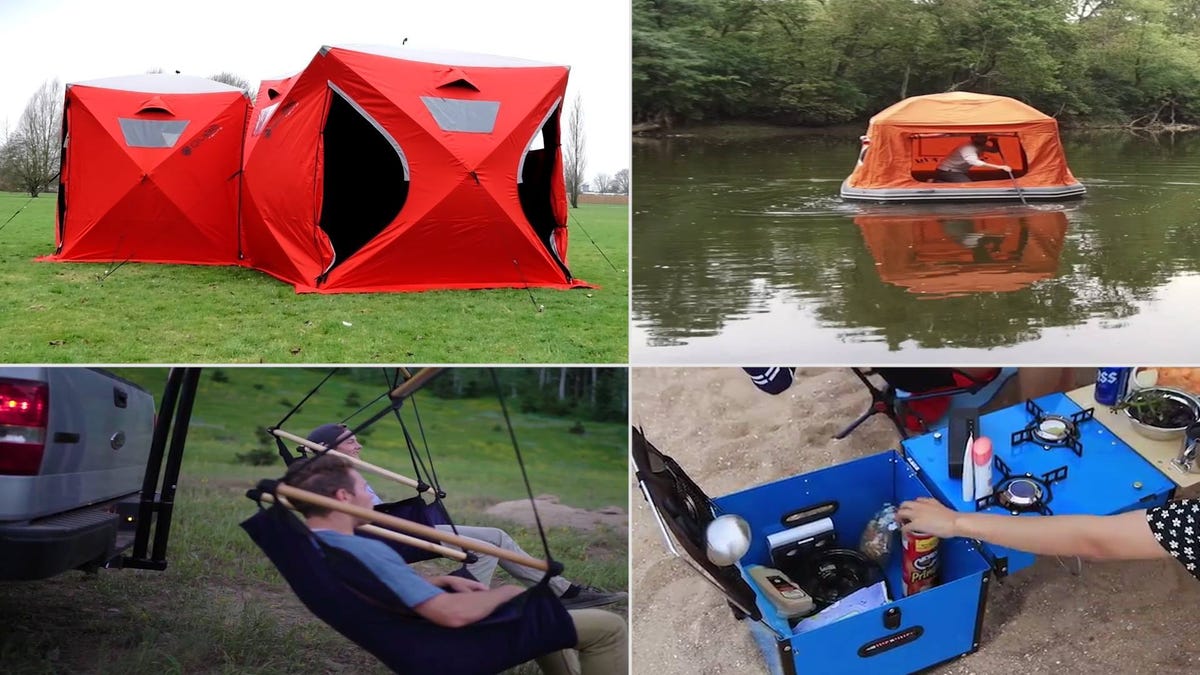
.css-cuqpxl:before{padding-right:0.3125rem;content:'//';display:inline;} Pop Mech Pro: Science .css-xtujxj:before{padding-left:0.3125rem;content:'//';display:inline;}
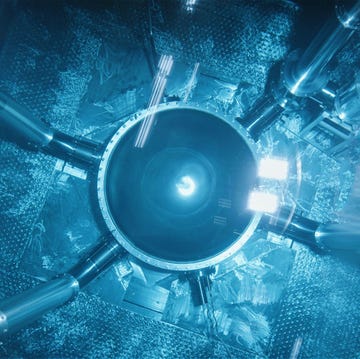
The Source of All Consciousness May Be Black Holes
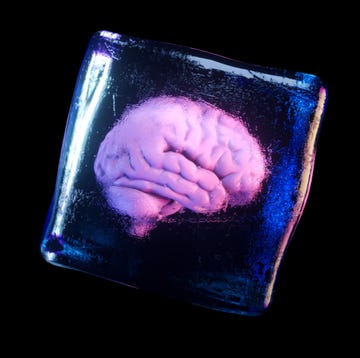
Could Freezing Your Brain Help You Live Forever?
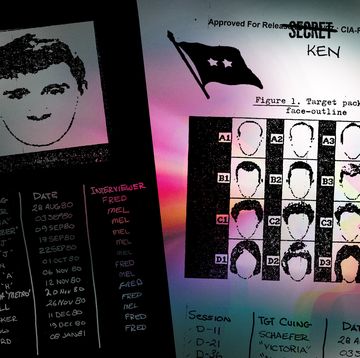
The CIA’s Secret Plan to Use Mind Control
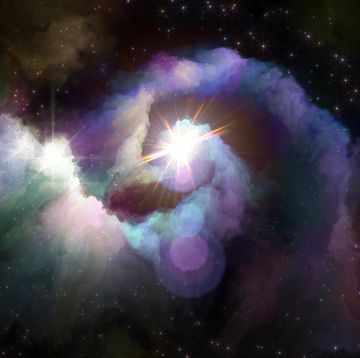
The Universe Could Be Eternal, This Theory Says
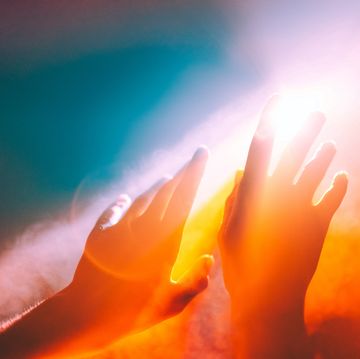
Immortality Is Impossible Until We Beat Physics
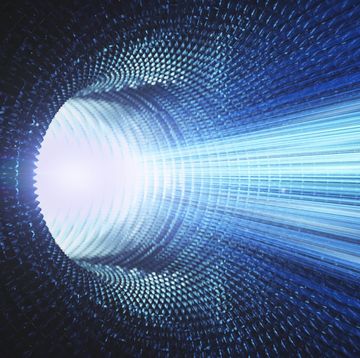
How Vacuum Energy Could Help Us Reach Light Speed
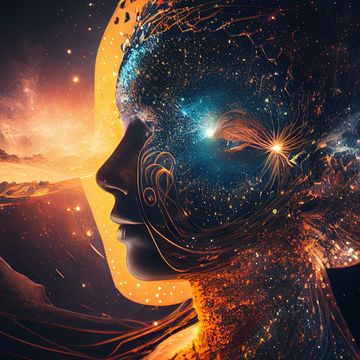
Could the Chair You Sit on Have a Soul?
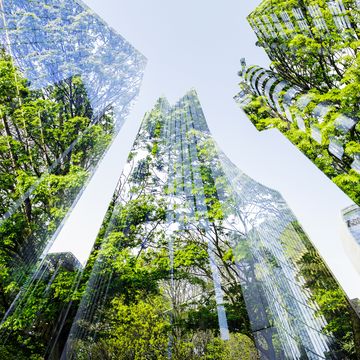
Here’s How We Could Live in Trees
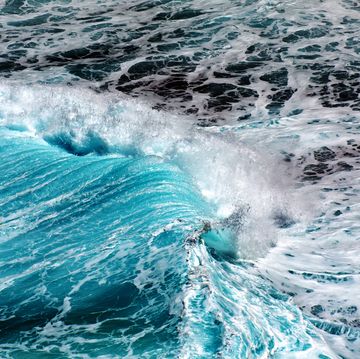
The Engine Driving Our Oceans Could Die by 2100
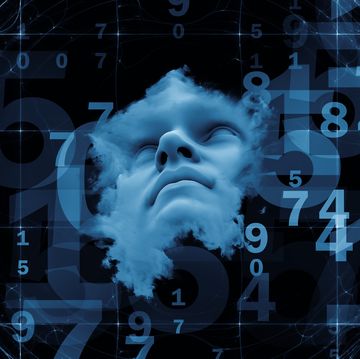
Can AI Help Solve Math’s Thorniest Mysteries?
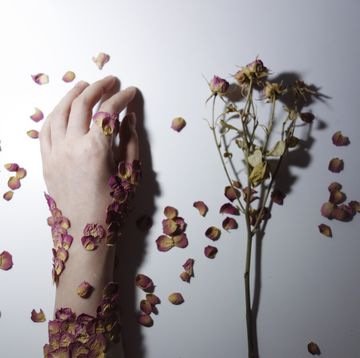
You Can Give Your Body Back to Nature When You Die
Bachelor's in Materials Science and Mechanical Engineering
Why pursue a bachelor’s degree in materials science and mechanical engineering.
Mechanical Engineering is critical to the success of many human enterprises. It plays a central role in the generation and distribution of energy, transportation, manufacturing, and infrastructure development. Nearly every product or service in modern life has been touched in some way by a mechanical engineer.
At Harvard College, students choose a "concentration," which is what we call a major. All prospective undergraduate students, including those intending to study engineering and applied sciences, apply directly to Harvard College . During your sophomore spring you’ll declare a concentration, or field of study. You may choose from 50 concentrations and 49 secondary field (from Harvard DSO website ).
Students can pursue a degree with an emphasis in mechanical engineering through the following concentrations:
- A.B. in Engineering Sciences - Mechanical and Materials Science and Engineering Track
- S.B. in Mechanical Engineering
Learn about some of our Mechanical Engineering Concentrators >
Apply to Harvard College >
Bachelor of Arts (A.B.)
Materials Science and Mechanical Engineering is a discipline of engineering that uses the principles of physics and materials science for the analysis and design of mechanical and thermal systems. The objectives of the Mechanical and Materials Science and Engineering Track of the Engineering Sciences A.B. program are to provide students a solid foundation in mechanical engineering study within the setting of a liberal arts college for preparation for a diverse range of careers in industry and government or for advanced work in engineering, business, law, or medicine.
Bachelor of Science (S.B.)
Mechanical Engineering concentrators receive a foundational education in a discipline central to challenges in energy, transportation, manufacturing, robotics, and the development of public infrastructure. Mechanical Engineering deals with the study and application of mechanical and thermal systems and covers a range of subtopics including mechatronics and robotics, structural analysis, thermodynamics and engineering design including the analysis of mechanical systems using finite element methods, the science of new materials, and devices for micro electromechanical systems (MEMS) and biological and nanotechnology applications.
AB/SM Materials Science and Mechanical Engineering Degree Program
Our AB/SM degree program is for currently enrolled Harvard College students only.
Prerequisites
Learn about the prerequisites for the concentration and the differences between the S.B. and A.B. tracks on on our First-Year Exploration page . Students interested in concentrating in Mechanical Engineering can be matched with a Peer Concentration Advisor. PCAs serve as peer advisors for pre-concentrators (and current concentrators), providing a valuable perspective and helping students to discover additional resources and opportunities. Learn more about the Peer Concentration Advisor program .
AB/SM Program
Our AB/SM degree program is for currently enrolled Harvard College students only.
Requirements
Learn more about the Mechanical Engineering requirements .
Materials Science and Mechanical Engineering Degree Courses
View current Mechanical Engineering courses .
Research Opportunities in Mechanical Engineering
As part of your Mechanical Engineering coursework, or perhaps as part of individual research opportunities working with professors, you will have the chance to take part in or participate in some extraordinary projects. Learn more about research opportunities at Harvard SEAS .
Learn about the research interests of our Materials Science & Mechanical Engineering faculty .
Mechanical Engineering Career Paths
Read about some of our Mechanical Engineering Alumni .
Clubs and Organizations
SEAS-affiliated student organizations are critical to the overall growth of our concentrators as engineering and applied science professionals. These organizations enable our students to pursue passion projects and events in areas of interest that are complementary to the current formal academic curriculum. Learn more about SEAS-affiliated student clubs and organizations .
In Materials Science & Mechanical Engineering
- Undergraduate Engineering at Harvard
- Concentration Requirements
- How to Declare
- Who are my Advisors?
- Sophomore Forum
- ABET Information
- Senior Thesis
- Research for Course Credit (ES 91R)
- AB/SM Information
- Peer Concentration Advisors (PCA) Program
- Student Organizations
- How to Apply
- PhD Timeline
- PhD Model Program (Course Guidelines)
- Qualifying Exam
- Committee Meetings
- Committee on Higher Degrees
- Research Interest Comparison
- Collaborations
- Cross-Harvard Engagement
- Clubs & Organizations
- Centers & Initiatives
- Alumni Stories
- History of Engineering Mechanics
Share Options
- Share to Facebook
- Share to Linkedin
AI Research at UVA Engineering
UVA Engineering has strength in artificial intelligence research and education: both core AI development and advanced AI applications. AI research areas are connected to all programs, involving more than 50 faculty researchers and offering student research opportunities.
UVA Professor Nikolaos Sidiropoulos Receives Lifetime Achievement Award in Electrical Engineering
The professor behind 5G wireless innovations, data-driven recommendations and MRIs.
CAREER Award: Fixing AI’s Blind Spot in Image Analysis
Miaomiao Zhang is improving AI image processing and analysis for real-world applications such as medical diagnoses and environmental monitoring.
Beyond the Finish Line: The Intersection of Speed, Safety and AI Innovation
UVA’s autonomous race car is reshaping not just the racetrack but the future of AI and robotics.
A Cognitive Assistant on Your Doctor’s Smartwatch?
Reimagining Alexa and Siri’s sensors for helping with patient communication.
UVA Engineering’s New Machine Learning Research Aims to Make Bank Financing More Inclusive
Fairness-aware AI research can explain how a policy affects different users and inform policies and consumer education programs.
New Artificial Intelligence Risk-Assessment Tool To Improve Heart-Failure Care
UVA Health and UVA Engineering researchers have developed a powerful new risk assessment tool for predicting outcomes in heart failure patients.
Engineering Professor Earns Grant To Improve Trust Between Humans and Robots
This UVA researcher is laying the foundation for better trust between humans and robots, with help from an Air Force Office of Scientific Research grant.
Using Machine Learning for Better Human Understanding of Disease Risk Factors and Treatments
Machine learning changed health care by making sense of biological data, but for UVA Engineering’s Aidong Zhang, that’s only part of understanding disease risk and treatments.
UVA Chemical and Biomedical Engineering Collaboration Offers Hope for Pulmonary Fibrosis Patients
A UVA Engineering team has developed a new analytical tool that uses AI to explore genes that could be targets for new treatments.
University of Virginia Joins the BrainChip University AI Accelerator Program
Program provides platforms and guidance to students at higher education institutions with artificial intelligence engineering programs training.
How Artificial Intelligence May Benefit Heart Patients
With machine learning and human learning, scientists uncover the biological cause and effect of fibroblast behaviors after heart attack and advanced the development of new treatments.
In the Blink of An Eye
Lee's vision for biomimicking sensor systems presents a complete story from materials to integration to application.
Trailblazer Award Will Use Advanced AI to Improve Outcomes for Heart Patients
Zhang's goal is to develop advanced machine learning and artificial intelligence techniques to analyze cine magnetic resonance imaging, an MRI procedure that visualizes the heart muscle in action.
Alzheimer's, Digital Twins and the Future of Medicine in the Age of AI
How digital twins and other AI developments are impacting access to diagnosis and treatments in Alzheimer's diseases.
CAREER Award: Designing the Next Generation of Wireless Communications Systems
Working in partnership with industry, Shen's machine-learning models can be prototyped, standardized and integrated into real systems.
CAREER Award: Teaching Machines the Art of Human Decision-Making
Li’s models will generate deeper insights into observational data to help people make better decisions.
Understanding Security and Privacy Risks Associated with Machine Learning
David Evans, professor of computer science in the University of Virginia School of Engineering and Applied Science, is leading research to understand how machine learning models can be compromised.
A Messenger for Medicine
Yanjun Qi builds artificial intelligence tools that have the power to turn biomedical data into better medicine.
UVA Biocomplexity Institute Developing Artificial Intelligence for Agriculture
Researchers at UVA Biocomplexity Institute are founding partners of a national research institute that will develop artificial intelligence-driven solutions for some of agriculture’s biggest problems.
The Race Is On
Cavalier Autonomous Racing built an artificial intelligence-driven vehicle for the world's first high-speed autonomous vehicle race.
FerroCoDE Platform Will Efficiently Compute Solutions for Complex Problems
A $1.5 million grant from the National Science Foundation’s ASCENT program supports UVA and Penn State researchers pursuing a new hardware platform.
UVA Computer Science Artificial Intelligence Research
Our department is advancing the state of the art in both fundamental algorithms as well as new algorithms to help users benefit from this revolution and provide new capabilities for the benefit of society.
Questions? Comments?
Office of communications.
The Office of Communications is charged with keeping all stakeholders well informed about the School's mission, vision, activities, progress and achievements. Web Issue? SUBMIT A REQUEST
The relationship between basic and applied research in universities
- Open access
- Published: 25 March 2015
- Volume 70 , pages 689–709, ( 2015 )
Cite this article
You have full access to this open access article
- Peter James Bentley 1 ,
- Magnus Gulbrandsen 2 &
- Svein Kyvik 3
57k Accesses
69 Citations
9 Altmetric
Explore all metrics
What is the central research activity in modern universities? This paper uses a comprehensive survey among individuals from 15 countries to map differences in orientation towards basic/fundamental research, applied/practical research and a combination of the two. Despite some claims in the literature that basic research is no longer a preoccupation of universities, our findings point at a continued strong presence of basic research in universities but with large variations between countries and academic disciplines. At the individual level, most academics engage in a combination of basic and applied research, rather than specialising, with applied orientations generally more common. Academics specialising in basic research tend to receive less external funding, work in environments where applied research is less emphasised and hold weaker professional obligations to apply their knowledge to problems in society.
Similar content being viewed by others
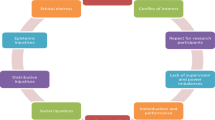
Ethical Issues in Research: Perceptions of Researchers, Research Ethics Board Members and Research Ethics Experts
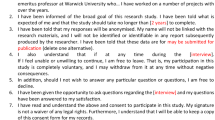
'Qualitative' and 'quantitative' methods and approaches across subject fields: implications for research values, assumptions, and practices
Research design: the methodology for interdisciplinary research framework.
Avoid common mistakes on your manuscript.
Introduction
The higher education literature is bursting with examples of how universities are changing in response to societal transformations and new demands. Many of these changes are described in rather pessimistic terms because they imply reduced funding, different activities and numerous tensions and challenges (Enders 2013 ). Only by protecting their so-called academic heartland can universities retain a degree of autonomy and a connection with widely shared values among their employees (Clark 1998 ). Long-term scientific work is at the centre of this heartland.
Basic research, or research undertaken with a primary purpose of the advancement of knowledge for its own sake, has traditionally been fundamental to university missions and their public funding. The “linear model” of innovation, popular during the post-1945 era, saw basic research as the primary contributor to innovation and economic growth, with universities as the institutional locus for public funding (Mowery and Sampat 2005 ). However, the centrality of basic research has come under question. The Mode 2 thesis, first outlined in The New Production of Knowledge by Gibbons et al. ( 1994 ), argued that disinterested, academic-oriented, disciplinary and autonomous research conducted exclusively within universities (Mode 1) is no longer the core mode of knowledge production. Mode 2 knowledge, produced within the context of application, has become the dominant form. Revisiting the Mode 2 discourse, Nowotny et al. ( 2003 ) summarised: “… the research that is variously described as ‘pure’, ‘blue-skies’, fundamental, or disinterested, is now a minority preoccupation—even in universities” (p. 184).
The Mode 2 diagnosis is broader than just the decline of fundamental research, and it also includes shifts towards collaborative and transdisciplinary research, greater heterogeneity in the sites of knowledge production, deeper social accountability and broader forms of quality control (beyond discipline-based peer review). The exceptionally wide scope of the Mode 2 discourse has been criticised for its lack of coherence and empirical validity (Hessels and Van Lente 2008 ). To date, there has been little evidence to support or refute Nowotny et al. ( 2003 ) claims, yet the Mode 2 discourse has influenced higher education and research policy makers and has been cited in almost ten thousand documents, with the vast majority of scientific papers treating it as an accepted or background concept (Hessels and Van Lente 2008 ).
Mode 2 is not necessarily new or unique, and it competes with other popular characterisations of the changing research agenda, some of which predate it. In the 1980s, Irvine and Martin ( 1984 ) identified the emergence of “strategic research”, combining academic excellence with an orientation towards future practical utility. According to Rip ( 2004 ), strategic research is the basic way of carrying out research in the regime of “strategic science”. However, strategic research retains a linearity between basic research and practical utility, including basic research as a cornerstone of its definition: “Strategic research [is] basic research carried out with the expectation that it will produce a broad base of knowledge likely to form the background to the solution of recognized current or future practical problems” (Irvine and Martin 1984 ). A similar conceptualisation, “translational research”, shares many of these characteristics, focussing on the harnessing of basic research for practical uses, particularly within the health sciences (Woolf 2008 ). Other conceptualisations, such as “finalised science” and “post-normal science”, also emphasise strong practice orientation, but these concepts are much more nuanced in their interpretation, limiting their implications to certain disciplines or policy-relevant fields (Weingart 1997 ).
More recently, the triple helix model of university–industry–government relations has also had considerable impact on research policy (Etzkowitz and Leydesdorff 2000 ). Like Mode 2, it contends that the future legitimation of science depends upon application orientation and contribution to economic development. However, it distinguishes itself from Mode 2 because universities retain distinctiveness as the core knowledge institutions and the application orientation process is emergent, rather than complete (Etzkowitz and Leydesdorff 2000 ). Academic capitalism also stresses the growing engagement of academics in application-oriented and commercially funded research, but the extent of such engagement is limited based on the closeness of one’s discipline to the market (Slaughter and Leslie 1997 ). Overall, the Mode 2 claims regarding the shifting research agenda away from fundamental research is unique only in its comprehensiveness, particularly with reference to country and disciplinary context.
This study is in particular interested in the claim that universities are no longer preoccupied with basic or theoretical research. Our decision to limit ourselves to this component follows Hessels and van Lente’s ( 2008 ) recommendation that Mode 2’s impact necessitates empirical research on each of the constitutive parts separately. We do so by drawing on data from an international survey among academic staff in research universities in 15 countries and across 10 academic fields. The lack of international comparable indicators has been a crucial limitation for understanding how universities operate in national innovation systems (Mowery and Sampat 2005 , p. 234). The comparative dimension allows us to test whether the assumptions surrounding Mode 2 apply to different contexts. Firstly, we investigate the extent to which individual academics worldwide consider basic or theoretical research a core part of their current research. Secondly, we examine the extent to which academics specialise only in basic or applied research, or combine these activities. Thirdly, we investigate variations in the extent to which academics specialise only in basic or applied research. Why might some academics within certain countries and academic fields choose to engage in both types of research, while others choose not to? We investigate three possible explanations. We expect engagement in basic and applied research to relate to: access to external commercial research funding; institutional expectations/policies; and individual normative/social behaviour.
Question 1: To what extent is basic research still a defining characteristic of universities across the world?
Nowotny et al. ( 2003 ) are clear regarding three generalisable causes for the decline in fundamental research: increased steering of research priorities; increased commercialisation of research (resulting from decreased public funding and increased attention to intellectual property ownership); and broader accountability of science.
The limited evidence of how individual academics view their primary research points to the contrary that basic research continues to be sustained as a major activity. A large-scale Finnish investigation found that an “academic orientation” with strong emphasis on basic research remains central across disciplinary fields (Ylijoki et al. 2011 ). The authors question the thesis that a significant transformation is taking place in universities, arguing instead that academics find different ways to combine an academic orientation with various forms of societal engagement. A UK interview study found the same, although it also emphasised how the term “basic research” is flexible and may be adapted to varying circumstances (Calvert 2006 ). Gulbrandsen and Kyvik ( 2010 ) also found that 90 % of Norwegian academics undertook basic research, with slightly less than half declaring their research “very much” basic (maximum value on a five-point scale), confirming other findings that basic research remains a strong identity marker for university staff (Gulbrandsen and Langfeldt 2004 ). These results are indicative of the resilience of basic research within universities, but may not be generalisable to other countries. Public funding of university research retains a strong position in Norway (Kyvik 2007 ).
National context is potentially of greater importance to understanding changes in knowledge production than implied by the Mode 2 thesis. Even if one acknowledges that universities across the world have experienced increased steering of research priorities and accountability pressures, it is unlikely that the effects would be uniform across countries. Shinn ( 2002 ) argues there is little evidence that science has become de-nationalised and it is inappropriate to generalise broad changes across national settings. According to Shinn, despite globalisation trends, universities, business and government still function predominantly within the national settings. The interaction between these institutions has implications for university governance and research behaviours. European countries have stronger traditions of academic self-determination, whereas market coordination has stronger traditions in most English-speaking countries and state control in parts of Asia (Clark 1983 ). Even within regions of similar academic traditions, convergences in higher education policies have not diluted the national character of academic labour markets and their internal regulation of research (Musselin 2005 ). This makes a data set from different countries particularly valuable.
National governments remain the main funder of university research in almost all OECD countries, but this varies from over 80 % of total funding of R&D in higher education institutions in Argentina, Australia, Italy, Finland, Germany, Norway and the Netherlands to closer to two-thirds in the USA, UK and Canada, and just over half of all funding in China (OECD 2013 ). Countries also differ in how this public funding is allocated to universities for research. According to Auranen and Nieminen ( 2010 ), core public research funding is determined predominantly via input-orientated measures in many European countries (e.g. Norway, Finland, Netherlands and Germany), whereas output-oriented funding models are more dominant in Australia and the UK. Although many European countries have been moving towards output-oriented funding models, there has been stronger resistance from universities and their implementation has been weaker. This likely reflects the traditionally stronger academic oligarchy within these countries (Clark 1983 ).
The implications for engagement in basic research is not directly clear, but one would reasonably assume that country-level differences in how universities are governed and funded would lead to greater diversity in research behaviours. For example, systems with greater levels of core funding based on input measures have higher levels of stability compared to output-oriented and externally funded systems (Auranen and Nieminen 2010 ). By implication, risky, fundamental and disinterested basic research may be more likely to flourish in the countries with more predictable funding. Although we cannot test empirically why country-level differences in basic research engagement may exist (countries differ across a range of idiosyncratic characteristics, not just governance or funding), it is appropriate to test the robustness of Nowotny and colleague’s general claims.
Question 2: To what extent do academics specialise only in basic or applied research, or combine these activities?
If universities are no longer preoccupied with pure, fundamental and disinterested research, what have become the dominant research preoccupations? Nowotny et al. ( 2003 ) discuss how Mode 2 knowledge production is characterised by diversity, reflexivity and a shift away from the stable, clear and unchallengeable taxonomy of disciplinary research. Further, the original Mode 2 argument stated that Mode 2 did not replace Mode 1; it supplemented and grew alongside it (Gibbons et al. 1994 ). Accordingly, individual academics engaged in Mode 2 knowledge production may continue to be involved in basic research alongside application-oriented research. Adding further complexity, application orientation (and the four other attributes characterising Mode 2 knowledge) is “not present in every instance of Mode 2” (Gibbons et al. 1994 , p. 8). Thus, application orientation is neither a prerequisite, nor a sufficient, characterisation of Mode 2. This is similar to Stokes’ ( 1997 ) argument that considerations of use often go hand in hand with a quest for fundamental understanding. Despite these challenges, one may reasonably understand Nowotny et al. ( 2003 ) argument to imply a shift in the balance between basic and applied research, towards more applied research specialists.
The few studies that have reported on this issue indicate, however, that a traditional academic orientation is resilient rather than new combinations. Albert ( 2003 ) found no evidence that Canadian academics in sociology and economics departments had shifted their research attention away from disciplinary research intended for their peers and begun specialising in interdisciplinary and practical research. Studies of scientists in the Netherlands (van Rijnsoever and Hessels 2011 ) and Finnish academics in technology, social science and humanities departments (Hakala and Ylijoki 2001 ) also indicate high commitment to Mode 1-type research over purely applied research. Finally, Gulbrandsen and Kyvik ( 2010 ) found that few Norwegian academics in 2000 were engaged exclusively in basic research, but this was still greater than the proportion engaged exclusively in applied research, although a combination was most common for all university staff members. Each of these aforementioned studies is somewhat limited by their national context. Our international data provide us with a useful opportunity to examine this relationship.
Another contextual limitation of the Mode 2 thesis is academic field (Hessels and Van Lente 2008 ). Disciplinary cultures span international boundaries and influence what types of research are considered legitimate within the scientific community (Becher 1994 ). Weingart ( 1997 ) argues that Mode 2 attributes, such as application-oriented research, make little sense in scientific disciplines distanced from policy makers. Likewise, Godin ( 1998 ) notes the difficulty certain disciplines face when modifying their research towards applied and practical purposes. According to Becher ( 1994 ), academic disciplines differ in their cognitive knowledge structure from pure (theoretical) to applied (pragmatic). Applied disciplines within the technological sciences (e.g. engineering) are purposive and pragmatic in their knowledge, oriented towards mastery of the physical environment, resulting in products/techniques. In the pure sciences (e.g. physics), knowledge is cumulative, atomistic, well organised and results in discovery or explanation, rather than directly practical outputs. Social sciences and humanities may also be pure or applied, depending on whether they are oriented towards practical, utilitarian and functional improvements to professional practice (e.g. education), or understanding and interpretation (e.g. humanities).
Based on Becher’s typology, we may expect specialisation in basic research to be more common in the hard-pure disciplines (e.g. life sciences and physical sciences) and soft-pure disciplines (humanities and social sciences). By contrast, specialisation in applied research may be more common in the science-based professions within hard-applied disciplines (e.g. agriculture, engineering, medical sciences) and social professions within the soft-applied disciplines (e.g. education, law). Our data will allow us to extend disciplinary-based findings from specific countries to an international context, controlling for other individual-level factors.
Question 3: What might explain individual differences in specialisation versus combining basic and applied research?
Few universities are completely insulated from the increased steering of research priorities, commercialisation and accountability pressures, but this does not imply that individual academics will respond in consistent and uniform ways. Despite the decline in autonomy, academics still retain a relatively high degree of discretion over their non-teaching hours and have the freedom to seek research activities of professional and personal engagement, although the latter may not always be compatible with a secure academic career (Davies 2013 ). Why might some academics in comparable disciplines and countries engage predominantly in basic research, while others do not? We put forward three possible explanations: commercial research funding, institutional expectations and professional norms.
Nowotny et al. ( 2003 ) argue that inadequacy of public funding has caused universities to shift their research preoccupation away from basic research. Government core funding of research has declined in a range of OECD countries, and the mechanisms for disbursing public funds have shifted from stable block grants towards results-based models
(Auranen and Nieminen 2010 ). Given that basic research leads to unpredictable results and tends to attract little private sector funding, we may expect that access to business and industry funding will also be a differentiator between individual academics for basic and applied research engagement within countries. Academics able to source business and industry funding for research are less reliant on dwindling institutional funding and may be more likely to be engaged in applied research.
A second important factor is institutional expectations for research. Universities across the world have seen stakeholder expectations widen and deepen, including the expectations for producing practical knowledge and training. Clark ( 1998 ) describes the situation in terms of a demand-response imbalance: “demands on universities outrun their capacity to respond” (p. 6). In terms of functional differentiation, modern universities are characterised by “overcomplexity and underdifferentiation” (Enders 2013 ). However, universities are not entirely victims of past history and stakeholder demands. Universities are capable of choosing which stakeholder demands to respond to, where within the organisation these responses will occur and how. For example, entrepreneurial responses may occur at the “developmental periphery”, where traditional disciplinary research meets the outside world through interdisciplinary research, contract research, contract education and consultancy (Clark 1998 ). Such engagement with external stakeholders can diversify the funding base, promote an entrepreneurial culture throughout the university, as well as stimulate the “academic heartland” where traditional disciplinary and theoretical research is maintained. In other words, universities have the capability to respond to stakeholder needs by promoting commercial and interdisciplinary research. Therefore, we may expect that academics who work in such environments will be more likely to engage in applied research and not necessarily to the detriment of their engagement in basic research.
A third factor explaining diversity is professional norms. One of the defining characteristics of academia is autonomy and protection from external influence. The freedom of professors to teach without external control was a core principle of the mediaeval university and has influenced the development of universities, not just in Central and Western Europe, but also in the USA (Altbach 2001 ). The emergence of privately funded, practically oriented research for commercial or technology transfer purposes in the mid- to late twentieth century challenged established scientific norms, particularly the norms of disinterested research and public ownership of knowledge (Stuart and Ding 2006 ). The changing funding patterns have been described as placing the future of basic research in jeopardy (Altbach 2001 ). However, scientific norms are not static. Etzkowitz ( 1998 ) argues that scientific norms have progressively accepted commercial research and academic entrepreneurialism with profit-making motives. These normative changes may be considered the “second revolution” in academia, following the first revolution which made research a core function of universities. Given the challenges that applied research poses to traditional scientific norms, we expect that engagement in applied research will reflect how individuals view applied research. Those who view applied research as a professional obligation will be more likely to be more engaged in applied research.
Data and methodology
The data come from the changing academic profession (CAP) international survey of the academic profession across 19 countries during 2007–2008: Argentina, Australia, Brazil, Canada, China and Hong Kong, Finland, Germany, Italy, Japan, Malaysia, Mexico, the Netherlands, Norway, Portugal, South Africa, Republic of Korea, UK and the USA. The CAP project used a common random sampling protocol and survey instrument. The target population of the CAP survey was professionals in universities or other higher education institutions offering a baccalaureate degree or higher. Given that this article addresses research orientation, we restrict ourselves to academics employed in universities with research and teaching missions, generally those universities awarding doctorate degrees (i.e. excluding polytechnics, teaching-focused colleges, research institutes). We exclude four of the 19 countries from our analysis. This is due to an inability to differentiate between teaching-focused and research-focused universities or small sample sizes (Japan and Korea), low response rate (Portugal) or limited information on the sampling framework (South Africa).
The response rates, including partially completed responses, were mostly below 40 per cent. Therefore, there is a potential risk of non-response bias in some countries. However, national samples were found broadly representative of the respective populations on strata such as gender, academic rank and institutional type (Cummings and Finkelstein 2012 ; RIHE 2008 ; Vabø and Ramberg 2009 ). Further, there are few reasons to expect that non-respondents would differ substantially in terms of self-declared research orientation. After removing non-respondents to the core questions regarding basic and applied research orientation and respondents not employed in academic ranks, we were left with a sample of 12,379 academics (Table 1 ).
Dependent variable: measuring basic and applied research
We consider Nowotny et al. ( 2003 ) references to “pure”, “blue skies”, “fundamental” or “disinterested” research to be analogous to basic or theoretical research and context of application to be analogous to applied or practically oriented research. Our data for measuring research orientation come directly from the CAP survey. The relevant question asked: “How would you characterize the emphasis of your primary research this (or for the previous) academic year?” Respondents provided a score ranging from “very much” (1) to “not at all” (5) on seven separate criteria. This study examines the results for two of these criteria: “basic/theoretical” and “applied/practically oriented”. The explicit reference to theory and practical orientation is consistent with the extended definitions of basic and applied research in the OECD’s Frascati Manual ( 2002 ). Given that respondents provided scores on each criterion separately, it was up to individual to determine whether the concepts of basic and applied research were mutually exclusive or inclusive.
We are interested both in the extent to which basic research is a defining characteristic of academic work, as well as its relationship with applied research. We distinguish between five distinct research orientations: pure basic; leaning towards basic; equally, basic and applied; leaning towards applied; and pure applied. A sixth category for respondents with neither basic nor applied research orientation is a residual category for those not declaring a clear research orientation. The definition of each research orientation group and number of respondents in each group is presented as a cross-tabulated matrix in Table 2 .
There are certain limitations which may affect how the research results are interpreted. Firstly, there is a risk that the concepts of “basic”, “theoretical”, “applied” and “practically oriented” may be understood differently across countries, particularly after translation into local language. In countries where English was not the language of the survey, most used a combination of professional translators, academic translators, survey piloting and peer review (e.g. regions with common languages), in addition to internal review by the project team.
Although we are confident the concepts of basic and applied research were translated accurately, there is a risk the concepts are interpreted differently, particularly in countries where research has not traditionally been conducted within universities. Secondly, one assumes that differences in response to Likert-type questions reflect differences in substantive engagement and that engagement is related to contextual factors such as research norms of the discipline or institution. However, academics may exaggerate or define their research in accordance with norms, rather than contextual factors influencing substantive engagement.
Independent variables: factors associated with research orientation
The operationalisation of independent variables is outlined below. Means on each variable are listed for each country in Table 4 of “ Appendix ”.
Country is a dichotomous variable based on country of employment: Argentina; Australia; Brazil; Canada; China; Finland; Germany; Hong Kong; Italy; Malaysia; Mexico; Netherlands; Norway; UK; and USA.
Academic discipline is a dichotomous variable based on discipline of one’s current academic unit: education (teacher training and education science); humanities (humanities and arts); social sciences (social and behavioural sciences); commerce (business and administration, economics); law; life sciences; physical sciences (physical sciences, mathematics, computer sciences); engineering (engineering, manufacturing and construction, architecture); agriculture; medicine (medical sciences, health-related sciences, social services); and other (personal services, transport services, security services or other). Based on Becher’s ( 1994 ) disciplinary typology, we expect basic research to continue to be a core preoccupation of academics within hard-pure sciences and soft-pure social sciences and humanities.
Commercial research funding is the percentage of one’s research funding (in the current or previous academic year) which came from business firms or industry. We expect that engagement in applied research will be greater among those academics with greater access to external commercial research funding.
Individual normative/social behaviour includes one scale variable based on the ordinal responses (from “strongly agree” [5] to “strongly disagree” [1]) to the following statement: “Faculty in my discipline have a professional obligation to apply their knowledge to problems in society”. We expect that engagement in applied research will reflect the extent to which individuals view applied research as a professional obligation.
Institutional norms and expectations/policy/strategy includes one scale variable based on the ordinal responses (from “strongly agree” [5] to “strongly disagree” [1]) to the following statement: “Your institution emphasizes commercially-oriented or applied research”. We expect that academics working in environments emphasising commercially oriented and applied research will tend to engage more in applied research.
Basic research as a pre-occupation
Our first research question addressed the extent to which basic research is still a defining characteristic of universities. When asked to characterise the emphasis of their primary research in the previous year on a scale of 1 (“very much”) to 5 (“not at all”), 61 % reported significant engagement in basic research (values of 1 or 2). Very few academics (7 %) reported being “not at all” engaged in basic research, with the remainder engaged partly in basic research (32 %). Based on these results, Nowotny et al. ( 2003 ) claim that fundamental research is a minority preoccupation in universities received little empirical support, with 93 % reporting at least some engagement with basic research. At the very least, claims that fundamental research is a minority preoccupation should be qualified by country. Levels of significant engagement in basic research ranged from 78 % in China to half of all academics in Argentina (50 %). Universities in Argentina, and to a lesser extent, Brazil, tend to employ a large number of part-time and teaching-only staff, but this does not account for their relatively weak engagement in basic research. Part-time staff in these countries did not differ from their full-time colleagues in their engagement in basic research.
Although most academics engage in basic research, a greater proportion report engagement in applied research. To this extent, fundamental research may not be the primary preoccupation of universities, even though the majority engage in it. More than two-thirds (69 %) of academics characterised their research as applied to a large extent, one quarter (25 %) were partly engaged in applied research, and 6 % not at all. China was again the country where academics were most likely to report strong engagement in applied research (86 %). Norway and the Netherlands were the only countries where more academics reported significant engagement in basic research compared to applied research. The proportion of academics in each country reporting significant engagement in basic research (values of 1 or 2) is shown in Fig. 1 on the left-hand bar, while the proportion reporting significant engagement in applied research is shown on the right-hand bar. Note that the basic and applied characterisations were derived from separate questions. Therefore, it was up to the respondent to decide whether the two categories (basic and applied) were mutually exclusive characterisations. The fact that sum of both bars exceeds 100 % in all countries is evidence that many academics do not consider these characterisations as mutually exclusive.
Proportion of academics whose primary research was to a considerable extent basic/theoretical ( left-hand bar ) and applied/practical ( right-hand bar ), by country
Specialisation versus the coexistence of basic and applied research
In order to investigate the relationship between basic and applied research, and tendency towards specialisation, we exclude respondents who were not sufficiently engaged in basic or applied research. Rather than being engaged in research outside the basic/applied typologies, academics with no clear research orientation are better understood as insufficiently research active. For example, they published little, spent very few hours on research and were also unlikely to characterise their research in other ways (e.g. commercially oriented, single disciplinary or interdisciplinary). These 998 respondents were classified as “neither basic nor applied” in Table 2 (8 % of the total sample) and are subsequently excluded from the valid cases for further analysis.
Among academics with clear research orientations, 14 % specialised as “pure basic” researchers. On this basis, only a small minority of academics may be understood to be preoccupied entirely with basic research. However, the lack of specialisation was not primarily due to a tendency to specialise in applied research, and only 17 % could be considered “pure applied” researchers. The bulk of the sample engaged in both basic and applied research. The most frequent categorisation was “equally, basic and applied” (27 %), followed by “leaning towards applied” (24 %) and “leaning towards basic” (18 %). In other words, basic research is not the primary preoccupation of most academics, but this is because it is integrated with applied research in most cases.
Specialisation varied considerably across countries. Pure basic researchers were most common in Italy, Netherlands and Norway, comprising roughly one quarter of all academics and outnumbering those in the pure-applied category. At the other extreme, almost no academics were specialised in basic research in China (2 %) and Malaysia (5 %). However, this reflected a greater tendency to combine basic and applied research in these countries, rather than an aversion to basic research. The balance between basic and applied research was most skewed in Australia, where pure applied researchers were more than twice as common as pure basic researchers. The proportion of academics specialising in basic or applied research by country is shown in Fig. 2 . The full breakdown across the five research orientation categories is shown for each country in Table 5 of “ Appendix ”.
Proportion of academics engaged purely in basic/theoretical research ( left-hand bar ) and purely applied/practical research ( right-hand bar ), by country
Research specialisation was closely aligned with Becher’s ( 1994 ) disciplinary typology of pure/theoretical and applied/pragmatic knowledge structures. Pure basic researchers were most common in the humanities (28 %), life sciences (22 %), social sciences (19 %) and physical sciences (18 %). These discipline-based differences were broadly consistent across country samples, with humanities ranked in the top 3 basic research disciplines in all countries other than Australia. Comparably, few pure basic researchers were located in Becher’s applied disciplines within the science-based and social professions, such as medicine (9 %), business studies (8 %), education (7 %), agriculture (4 %) and engineering (3 %). The balance between specialisation in basic or applied research also aligns with Becher’s typology, with few disciplines having a roughly equal number of pure applied and pure basic researchers. One possible exception may be law, where pure applied research was rare (10 %) compared to pure basic (18 %). This appears to contradict the practice orientation of the discipline. However, it may be that purely “practically oriented” research is defined differently by law academics, given that research within a legal practice involves direct remuneration for specific clients. The proportion of academics specialising in basic or applied research by discipline is shown in Fig. 3 (full breakdown by discipline in Table 6 of “ Appendix ”).
Proportion of academics engaged purely in basic/theoretical research ( left-hand bar ) and purely applied/practical research ( right-hand bar ), by discipline of current academic unit
Compared to the country-level results where pure applied researchers were only marginally more common than pure basic researchers, research orientation is considerably more fragmented across disciplines. This also supports Becher’s claims that disciplinary cultures often span national boundaries and embody collective norms. Taken together, the large differences across countries and disciplines suggest that claims regarding basic, theoretical or blue sky research should be nuanced with reference to national and disciplinary context.
Multinomial results
Our third research question was how we might account for individual-level differences in engagement and specialisation in basic and applied research. From the above results, the disciplinary implications are clear. Given that countries differ in their proportion of academics by discipline, cross-country comparisons need to control for these differences. However, significant variation within countries and disciplines suggests that other factors may also influence specialisation. To examine this further, we computed a multinomial logistic regression with three categorical outcomes: pure basic, pure applied and basic and applied as the reference category (merging the three categories which involved both types of research). Controlling for country and discipline, we investigated whether specialisation in basic or applied research was also associated with differences in access to commercial research funding, institutional expectations/policies and individual normative/social behaviour. We also checked whether the results differed when controlling for demographic characteristics (gender, age and age squared), but the main results did not change. The multinomial logistic regression results are presented in Table 3 .
The likelihood ratio tests from the multinomial regression results (not shown) indicated a statistically significant relationship between research specialisation and commercial research funding, organisational expectations and individual norms. However, organisational expectations for commercially oriented or applied research (institutional norms) were not a statistically significant factor differentiating between all categories of research specialisation. Compared to the reference category for those engaged in both basic and applied research, and holding other factors constant, a one unit increase in agreement (on a five-point scale) with the statement “Your institution emphasizes commercially-oriented or applied research” was only statistically significant in its negative association with being a pure basic researcher (with a decrease in the multinomial log odds of 0.15 per unit increase). Agreement with this statement was not significant in its relationship with being a pure applied researcher. In other words, institutional norms emphasising research commercialisation appear to increase engagement in applied research but not specialisation in this type of research. On the other hand, individual norms were significantly associated with both types of specialisation. A one unit increase in agreement with the statement that “Faculty in my discipline have a professional obligation to apply their knowledge to problems in society” was associated with an increased likelihood of being pure applied. Agreement was even more strongly associated with a decrease in the likelihood of being a pure basic researcher.
External commercial research funding was a significant differentiator for research specialisation, but mostly in its negative relationship with pure basic research specialisation. A one point percentage increase in the proportion of one’s research funding coming from business or government sources was associated with a small but statistically significant decrease in the likelihood of being a pure basic researcher. To a lesser extent, commercial research funding was also positively associated with pure applied research. In other words, commercial research funding tends to increase the likelihood of being engaged in applied research, but mostly in combination with basic research rather than in specialisation. This relatively minor relationship is partly due to the small share of academics for whom commercial funding is a main source of research funding. In most countries, the mean proportion of research funding from business and industry was around 5 % or less. However, in Germany and China, these proportions were considerably greater at 10 and 15 %, respectively (see Table 4 of “ Appendix ”). This is consistent with their high proportion of higher education R&D expenditure financed by industry, at 14 and 33 %, respectively, versus 6 % across all OECD countries (OECD 2013 ).
The control variables for country and discipline remained statistically significant in their relationship to research specialisation. Specialisation in pure basic or pure applied research was significant and negatively associated with being located in China and Malaysia (compared to the reference category of USA), which was to be expected given the aversion towards specialisation found in the descriptive results. The other regional patterns that remained significant were the greater likelihood of pure basic researchers to be located in the Western European countries of Italy, Norway, the Netherlands and Finland. Disciplinary differences continued to reflect Becher’s ( 1994 ) typologies. Pure basic researchers were significantly more likely to be located in the humanities, while pure applied researchers were significantly more likely to be located in engineering, agriculture, medicine and commerce.
In summary, pure basic researchers could be more clearly differentiated from others based on their research funding, professional norms and perceptions of institutional expectations, while pure applied researchers share more in common with those engaged in both basic and applied research.
Discussion and conclusion
Basic research is an activity that continues to define academic work at most research universities around the world. The majority of academics (61 %) in our data material based on more than 10,000 researchers from 15 countries report significant engagement in basic research, and very few report no engagement in basic research (7 %). Although these aggregate results mask considerable diversity across countries, there is little evidence that basic research is a minority preoccupation in any of the countries for which we have data. However, more academics are engaged in applied research than basic research. Those academics that engaged in both types did not do so equally, with more academics leaning towards applied over basic. This suggests there are some trade-offs between the two activities. Therefore, basic research may be considered to be an important part of most academics’ research, but often secondary to applied research. Our cross-sectional data do not allow us to investigate the extent to which theoretical research has declined from a dominant position, but the resilience of theoretical research lends stronger support to the more nuanced position of the triple helix model (Etzkowitz and Leydesdorff 2000 ). The shift towards Mode 2 or practical research appears to be emergent, rather than complete, with universities remaining a core producer of theoretical knowledge.
Our research generally supports Stokes ( 1997 ) since a main result is that combining basic and applied work is the norm for the majority of academics, regardless of country and discipline. It also supports earlier work claiming that traditional academic orientations remain strong in universities, even in periods of decreased core funding and increased pressure for various forms of societal engagement (Ylijoki et al. 2011 ). We still do not have sufficient evidence to conclude whether the frequent “hybrid profiles” of academics are productive and meaningful or whether they to a greater extent represent incommensurable activities leading to stress and insecurity (c.f. Davies 2013 ).
Disciplinary-based analyses showed basic research to be relatively weaker in the professional social science disciplines (e.g. business and education) and the applied sciences (engineering, agriculture and medicine). Academics within these disciplines were rarely primarily focused on theoretical research, but these patterns probably reflect the cognitive knowledge structures of these disciplines and their orientation towards practical outputs (Becher and Trowler 2001 ), rather than a weakening importance of theoretical research. The diversity within these disciplines also suggests that theoretical research can be an important, and even dominant, motivation. Highly practical results may be the starting point for conducting research in some applied disciplines, but academic prestige has a strong appeal for academics across various disciplines (Hakala and Ylijoki 2001 ). Nevertheless, with up to around one-third of academics in the applied sciences engaging purely in applied research, compared to less than one-fifth in other fields, a complete devotion towards practice orientated research is clearly more relevant in certain fields. This lends stronger support to the field-dependent interpretations offered by Weingart ( 1997 ) and Woolf ( 2008 ) in their competing frameworks for understanding changes to the science system.
Our cross-country results are less easy to explain and should be treated with caution due to the limitations of the data. The strong position of basic research in Finnish and Norwegian universities has been noted previously (see Ylijoki et al. 2011 ; Gulbrandsen and Kyvik 2010 ), but our international results from other countries suggest a more nuanced interpretation. Although more academics were significantly engaged in basic research than applied research in Norway and the Netherlands (with an equal division in Italy), these countries were the exceptions. Applied research dominated in most countries, particularly in Australia where academics were roughly twice as likely to specialise or lean towards applied research compared to basic research. This tendency was also noticeable in the USA and Hong Kong. One possibility is that applied research engagement reflects academic governance systems, with a stronger academic oligarchy protecting the place of basic research compared to market-driven systems (Clark 1983 ), but this was not consistent with results in all countries.
Institutional norms emphasising research commercialisation were weakest in Mexico, Argentina and Brazil, and relatively weaker in most European countries (see Table 4 of “ Appendix ”). This was consistent with traditional systems of bureaucratised governance within Latin American universities, which have been criticised for creating few incentives to engage in research commercialisation and research productivity more generally (Thorn and Soo 2006 ). However, although each of these institutional aspects differentiated between individuals within countries in consistent ways, the differences across countries remained. For example, strong institutional-level norms towards research commercialisation in the UK were not consistent with the rather moderate engagement in applied research in this country. Nor were weak commercialisation norms in Brazil and Argentina consistent with the moderate engagement in applied research and weak engagement in basic research. In Latin America, it has been claimed that university discourse has shifted enthusiastically towards Mode 2, but the academic evaluation system remains rooted in Mode 1 outputs, leading to an “evaluation system schizophrenia” (Arocena and Sutz 2001 , p. 1231). Engagement in basic and applied research clearly has strong country-level features, implying that generalised statements about basic or applied research must be qualified for local context.
China and Malaysia are particularly interesting countries because both applied research and basic research were strong self-characterisations. Neither country has strong university research traditions, but their respective governments have recently increased their expectations for basic research as part of innovation and development plans (Zhu and Gong 2008 ; Lee et al. 2013 ). Up until the mid-1980s, Chinese universities were predominantly teaching focused, receiving no specific funding for research. Basic research was the responsibility of the Chinese Academy of Science and other national research institutes, while applied research was conducted by research institutes in industrial sectors (Zhu and Gong 2008 ). Malaysian universities have also been largely teaching focused and lacked an academic research culture (Lee 2003 ). Strong engagement in both basic and applied research may reflect an emerging research culture which supports both basic and applied research. According to Mohrman et al. ( 2008 ), at the same time that Chinese institutions are encouraging basic research, universities are also responding to demands for applied research as a service to society. This appeared to translate into individual-level norms. Academics in these two countries were most likely to report stronger agreement that academics had a professional obligation to apply their knowledge to problems in society.
However, the results for China and Malaysia also highlight a broader difficulty in defining basic and applied research engagement. Even though we are confident that concepts of basic/theoretical research and applied/practically oriented research were understood in each country and the investigation teams went to great lengths to ensure translations were accurate and comparable, there is a risk that subtle differences in understanding might be responsible for parts of the differences across countries in responses. Academics in some countries may declare a greater engagement in basic research due to, for example, a broader definition of what “theoretical” or “practical” research means. This also goes beyond linguistic differences to general understanding, acceptance and reward structures for basic and applied research. The strong relationship between individual norms, institutional expectations and self-reported engagement in applied research could indicate that academics are responsive to institutional policies supporting applied research. Unfortunately, individuals may also simply exaggerate behaviours in accordance with what they perceive to be the norms of their discipline and institution. This has implications for the growing expectations of research “relevance” from higher education stakeholders around the world (Enders and Musselin 2008 ). If most academics genuinely believe they are already deeply engaged in practical research, it may indicate they hold a different understanding of applied and practically oriented research compared to the policy makers articulating the need for greater engagement.
We have shown that the emphasis on applied research is not only related to funding and university strategies; it is even more strongly related to individual norms about academics obligations. National and institutional policies for more applied and practically related activities are therefore not necessarily out of touch with core academic values. Our main finding is that different types of research activities are mixed and combined at the individual level, despite variations between countries and between academic disciplines. This does not imply that the balance between basic and applied research is equal, or that basic research has not lost its pre-eminence, but it does imply that basic research retains a core position within the research mind sets of most academics. As such, our results can be interpreted as a warning against policies striving for clear division in the higher education landscape between institutions primarily doing basic research and others applied. This seems out of touch with widely shared norms and practices among the researchers themselves, who overwhelming combine these activities.
Albert, M. (2003). Universities and the market economy: The differential impact on knowledge production in sociology and economics. Higher Education, 45 (2), 147–182.
Article Google Scholar
Altbach, P. G. (2001). Academic freedom: International realities and challenges. Higher Education, 41 (1–2), 205–219.
Arocena, R., & Sutz, J. (2001). Changing knowledge production and Latin American universities. Research Policy, 30 , 1221–1234.
Auranen, O., & Nieminen, M. (2010). University research funding and publication performance—An international comparison. Research Policy, 39 (6), 822–834. doi: 10.1016/j.respol.2010.03.003 .
Becher, T. (1994). The significance of disciplinary differences. Studies in Higher Education, 19 (2), 151–161.
Becher, T., & Trowler, P. (2001). Academic tribes and territories: Intellectual enquiry and the culture of disciplines . Philadelphia, PA: Open University Press.
Google Scholar
Calvert, J. (2006). What’s special about basic research. Science, Technology and Human Values, 31 , 199–220.
Clark, B. R. (1983). The higher education system . Berkeley: University of California Press.
Clark, B. R. (1998). The entrepreneurial university: Demand and response. Tertiary Education and Management, 4 (1), 5–16.
Cummings, W. K., & Finkelstein, M. J. (2012). 2. Concepts and methods. In W. K. Cummings & M. J. Finkelstein (Eds.), Scholars in the changing American Academy: New contexts, new rules, and new roles (pp. 15–26). Dordrecht: Springer.
Davies, S. (2013). Research staff and public engagement: A UK study. Higher Education, 66 (6), 725–739. doi: 10.1007/s10734-013-9631-y .
Enders, J. (2013). The university in the audit society: On accountability, trust and markets. In L. Engwall & P. Scott (Eds.), Trust in universities (pp. 53–62). London: Portland Press.
Enders, J., & Musselin, C. (2008). Back to the future? The academic professions in the 21st century. In Higher education to 2030: Demography (Vol. 1, pp. 125–150, Demography). Paris: OECD.
Etzkowitz, H. (1998). The norms of entrepreneurial science: Cognitive effects of the new university–industry linkages. Research Policy, 27 (8), 823–833. doi: 10.1016/S0048-7333(98)00093-6 .
Etzkowitz, H., & Leydesdorff, L. (2000). The dynamics of innovation: From National Systems and “Mode 2” to a Triple Helix of university–industry–government relations. Research Policy, 29 (2), 109–123.
Gibbons, M., Limoges, C., Nowotny, H., Schwartzman, S., Scott, P., & Trow, M. (1994). The new production of knowledge: The dynamics of science and research in contemporary societies . London: Sage.
Godin, B. (1998). Writing performative history: the new New Atlantis? Social Studies of Science, 28 (3), 465–483.
Gulbrandsen, M., & Kyvik, S. (2010). Are the concepts basic research, applied research and experimental development still useful? An empirical investigation among Norwegian academics. Science and Public Policy, 37 (5), 343–353.
Gulbrandsen, M., & Langfeldt, L. (2004). In search of Mode 2: The nature of knowledge production in Norway. Minerva, 42 , 237–250.
Hakala, J., & Ylijoki, O.-H. (2001). Research for whom? Research orientations in three academic cultures. Organization, 8 (2), 373–380. doi: 10.1177/1350508401082021 .
Hessels, L. K., & Van Lente, H. (2008). Re-thinking new knowledge production: A literature review and a research agenda. Research Policy, 37 (4), 740–760.
Irvine, J., & Martin, B. R. (1984). Foresight in science: Picking the winners . London: Pinter.
Kyvik, S. (2007). Changes in funding of university research. Consequences for problem choice and research output of academic staff. In J. Enders & B. Jongbloed (Eds.), Public–private dynamics in higher education: Expectations, developments and outcomes (pp. 387–411). Bielefeld: Transcript Verlag.
Lee, M. N. N. (2003). The academic profession in Malaysia and Singapore: Between bureaucratic and corporate cultures. In P. G. Altbach (Ed.), The decline of the guru: The academic profession in the third world (pp. 135–166). Chestnut Hill: Boston College.
Chapter Google Scholar
Lee, M. N. N., Sirat, M., Da, W. C., & Karpudewan, M. (2013). Appendix C: Effectiveness of research and innovation management at policy and institutional levels in Malaysia. In A. Olsson & L. Meek (Eds.), Effectiveness of Research and Innovation Management at Policy and Institutional Levels (pp. 90–120). Paris: OECD.
Mohrman, K., Ma, W., & Baker, D. (2008). The research university in transition: The emerging global model. Higher Education Policy, 21 (1), 5–27.
Mowery, D., & Sampat, B. (2005). The Oxford Handbook of Innovation. In J. Fagerberg, D. C. Mowery, & R. R. Nelson (Eds.), Universities in national innovation systems (pp. 209–239). Oxford: Oxford University Press.
Musselin, C. (2005). European academic labor markets in transition. Higher Education, 49 (1–2), 135–154.
Nowotny, H., Scott, P., & Gibbons, M. (2003). Introduction: ‘Mode 2’ revisited: The new production of knowledge. Minerva, 41 (3), 179–194. doi: 10.1023/a:1025505528250 .
OECD. (2002). Frascati manual 2002: Proposed standard practice for surveys on research and experimental development . Paris: OECD.
Book Google Scholar
OECD. (2013). MSTI main science and technology indicators . Paris: OECD.
RIHE (Ed.). (2008). The changing academic profession in international comparative and quantitative perspectives (Vol. 12, RIHE International Seminar Reports). Hiroshima: Research Institute for Higher Education, Hiroshima University.
Rip, A. (2004). Strategic research, post-modern universities and research training. Higher Education Policy, 17 (2), 153–166.
Shinn, T. (2002). The triple helix and new production of knowledge prepackaged thinking on science and technology. Social Studies of Science, 32 (4), 599–614.
Slaughter, S., & Leslie, L. L. (1997). Academic capitalism: Politics, policies, and the entrepreneurial university . Baltimore: The Johns Hopkins University Press.
Stokes, D. E. (1997). Pasteur’s quadrant: Basic science and technological innovation . Washington, DC: Brookings Institution Press.
Stuart, T. E., & Ding, W. W. (2006). When do scientists become entrepreneurs? The social structural antecedents of commercial activity in the academic life sciences. American Journal of Sociology, 112 (1), 97–144.
Thorn, K., & Soo, M. (2006). Latin American universities and the third mission: Trends, challenges, and policy options [Working Paper, no. 4002]. World Bank Policy Research . The World Bank.
Vabø, A., & Ramberg, I. (2009). Arbeidsvilkår i norsk forskning . Oslo: NIFU STEP.
van Rijnsoever, F. J., & Hessels, L. K. (2011). Factors associated with disciplinary and interdisciplinary research collaboration. Research Policy, 40 (3), 463–472. doi: 10.1016/j.respol.2010.11.001 .
Weingart, P. (1997). From “Finalization” to “Mode 2”: Old wine in new bottles? Social Science Information, 36 (4), 591–613.
Woolf, S. H. (2008). The meaning of translational research and why it matters. JAMA, 299 (2), 211–213.
Ylijoki, O.-H., Lyytinen, A., & Marttila, L. (2011). Different research markets: A disciplinary perspective. Higher Education, 62 (6), 721–740. doi: 10.1007/s10734-011-9414-2 .
Zhu, Z., & Gong, X. (2008). Basic research: Its impact on China’s future. Technology in Society, 30 (3–4), 293–298. doi: 10.1016/j.techsoc.2008.05.001 .
Download references
Author information
Authors and affiliations.
LH Martin Institute, The University of Melbourne, Level 1, 715 Swanston Street, Carlton, VIC, 3010, Australia
Peter James Bentley
Centre for Technology, Innovation and Culture, University of Oslo, Postboks 1108, Blindern, 0317, Oslo, Norway
Magnus Gulbrandsen
NIFU Nordic Institute for Studies in Innovation, Research and Education, P.O. Box 5183, Majorstuen, 0302, Oslo, Norway
Svein Kyvik
You can also search for this author in PubMed Google Scholar
Corresponding author
Correspondence to Peter James Bentley .
See Tables 4 , 5 and 6 .
Rights and permissions
Open Access This article is distributed under the terms of the Creative Commons Attribution License which permits any use, distribution, and reproduction in any medium, provided the original author(s) and the source are credited.
Reprints and permissions
About this article
Bentley, P.J., Gulbrandsen, M. & Kyvik, S. The relationship between basic and applied research in universities. High Educ 70 , 689–709 (2015). https://doi.org/10.1007/s10734-015-9861-2
Download citation
Published : 25 March 2015
Issue Date : October 2015
DOI : https://doi.org/10.1007/s10734-015-9861-2
Share this article
Anyone you share the following link with will be able to read this content:
Sorry, a shareable link is not currently available for this article.
Provided by the Springer Nature SharedIt content-sharing initiative
- Basic research
- Applied research
- Academic profession
- Academic work
- Find a journal
- Publish with us
- Track your research

IMAGES
VIDEO
COMMENTS
Applied Scientific Research. Section B: Electrophysics, acoustics, optics, mathematical methods. Publishing model: Subscription. Applied Scientific Research is now archived and no longer receiving submissions with this publisher. All articles published in the journal during its time with Springer will remain fully searchable through our websites.
Applied research. Applied research is the practical application of science. It accesses and uses accumulated theories, knowledge, methods, and techniques, for a specific state-, business-, or client-driven purpose. In contrast to engineering, applied research does not include analyses or optimization of business, economics, and costs.
Open. This collection highlights research and commentary in applied science. The range of topics is large, spanning all scientific disciplines, with the unifying factor being the goal to turn ...
Applied research is a type of scientific inquiry that focuses on developing practical solutions to real-world problems. It involves the use of existing knowledge, theories, and techniques to address specific problems or challenges in a particular field or industry. ... Medical research: Applied research can be used to develop new treatments or ...
An applied scientist does scientific research with a focus on applying the results of their studies to solving real-world problems. They use the scientific method to develop research questions and then conduct studies that lead to practical solutions. These scientists work in various industries and apply their skills to develop functional ...
This research is used to examine new drugs in the medical industry. It combines scientific knowledge and procedures with health experiences to produce evidence-based results. Example: Applied study in heart surgery. Applied study to determine a drug's efficacy. Applied study on a medicine's adverse effects.
Applied Scientific Research is now archived and no longer receiving submissions with this publisher. All articles published in the journal during its time with Springer will remain fully searchable through our websites. Articles. Search all Applied Scientific Research articles Sort by: Date published (new to old) ...
Real-World Applications. Applied research refers to scientific study and research that seeks to solve practical problems. This type of research plays an important role in solving everyday problems that can have an impact on life, work, health, and overall well-being. For example, it can be used to find solutions to everyday problems, cure ...
Founded in 1998, Applied Scientific Research is a consulting firm, providing engineering and advanced computational and algorithm development support for the high performance computing needs of the U.S. government and industrial clients. Applied Scientific Research offers its expertise in: Computational Fluid Dynamics - CFD ; Unsteady fluid flow and heat transfer analyses
Applied Research is a multidisciplinary journal for researchers across the physical sciences, natural sciences, life sciences and engineering fields.. Our goal is to bridge the gap between fundamental and applied research and highlight the path towards the application of ideas through experiments, protocols, software, instrumentation, and other approaches.
Prevention science broadly refers to interdisciplinary, basic, and applied research on the etiology, development, and prevention of health, mental health, and other social problems. A key feature of prevention science is the translation of scientific research into practical preventive interventions.
Research conducted for the purpose of contributing towards science by the systematic collection, interpretation and evaluation of data and that, too, in a planned manner is called scientific research: a researcher is the one who conducts this research. The results obtained from a small group through scientific studies are socialised, and new ...
Scientific research is often broadly divided into two types: basic research (also called fundamental research) and applied research. Basic research is about pushing the boundaries of our understanding and generating new knowledge. An example is researching how a physiological process works at the molecular level.
Similarly, for the development of new and improved materials, energy systems, and electronics, to name but a few, we need applied science to develop our initial discoveries into usable technologies.
The goal of the Applied Science organization at Google is to cross-fertilize these two fields. There are four main efforts in Applied Science: Quantum Computing, Google Accelerated Science, Climate and Energy, and Scientific Computing Tools. Quantum Computing uses advances in applied physics to push the state-of-the-art in computation.
Applied Scientific Research. Section B: Electrophysics, acoustics, optics, mathematical methods. Publishing model: Subscription. Applied Scientific Research is now archived and no longer receiving submissions with this publisher. All articles published in the journal during its time with Springer will remain fully searchable through our websites.
Applied research is original investigation undertaken in order to. acquire new knowledge; it is, however, directed primarily towards a specific, practical aim or. objective (OECD, 2015). It is ...
Applied research is a systematic and organized inquiry aimed at solving specific real-world problems or improving existing practices, products, or services. Unlike basic research, which focuses on expanding general knowledge, applied research is all about using existing knowledge to address practical issues.
Applied Research Example in Science. In itself, applied research is a scientific method of investigation because it applies existing scientific knowledge to practical situations. It is useful in different fields including thermodynamics, physics, material sciences and microbiology. Examples of applied research in science include the following:
The scientific method, as applied to social sciences, includes a variety of research approaches, tools, and techniques for collecting and analysing qualitative or quantitative data. These methods include laboratory experiments, field surveys, case research, ethnographic research, action research, and so forth.
Design equipment to help diagnose and treat medical conditions. Design and build artificial limbs and organs. Install, maintain, and repair various medical equipment. Act as medical science liaison to other professionals. 3. Statistician. Create polls, questionnaires, and surveys. Analyze data to help make decisions.
In research and development: Introduction and definitions. Applied research carries the findings of basic research to a point where they can be exploited to meet a specific need, while the development stage of research and development includes the steps necessary to bring a new or modified product or process into production. In….
In 2019, Munir Nazzal joined the University of Cincinnati College of Engineering and Applied Science as a professor in the Department of Civil and Architectural Engineering and Construction Management. Throughout his time at UC, he has received various accolades for his advancements in transportation research, including the UC George Rieveschl Jr. Award for Distinguished Scientific Research ...
The Applied Physics team's new research created this hypothetical model using the Warp Factory software toolkit. ... a science journalist, a tech writer, and a computer engineer. She's always ...
Support nonprofit science journalism. Sophisticated, trustworthy reporting about science has never been more important. As part of the AAAS mission, Science has built a global award-winning network of reporters and editors that independently cover the most important developments in research and policy.
Bachelor of Science (S.B.) Mechanical Engineering concentrators receive a foundational education in a discipline central to challenges in energy, transportation, manufacturing, robotics, and the development of public infrastructure. Mechanical Engineering deals with the study and application of mechanical and thermal systems and covers a range ...
Email [email protected]. Phone (434) 924-1713. UVA Engineering has strength in artificial intelligence research and education: both core AI development and advanced AI applications.
On April 23, members of the GW Engineering Environmental and Energy Management Institute (EEMI) met with delegates from the Sultanate of Oman to discuss collaborative research activities. Representing GW were Professor Scott Sklar, EEMI Director of Sustainable Energy, and Jonathan Deason, EEMI Executive Director.
Dependent variable: measuring basic and applied research. We consider Nowotny et al. references to "pure", "blue skies", "fundamental" or "disinterested" research to be analogous to basic or theoretical research and context of application to be analogous to applied or practically oriented research.Our data for measuring research orientation come directly from the CAP survey.
Compared to traditional industrial materials, composites have higher durability and compressive strength. However, some components may have flaws due to the manufacturing process. Traditional defect detection methods have low accuracy and cannot adapt to complex shooting environments. Aiming to address the issues of high computational requirements in traditional detection models and the lack ...