- Skip to main content
- Skip to FDA Search
- Skip to in this section menu
- Skip to footer links

The .gov means it’s official. Federal government websites often end in .gov or .mil. Before sharing sensitive information, make sure you're on a federal government site.
The site is secure. The https:// ensures that you are connecting to the official website and that any information you provide is encrypted and transmitted securely.
On Oct. 1, 2024, the FDA began implementing a reorganization impacting many parts of the agency. We are in the process of updating FDA.gov content to reflect these changes.

U.S. Food and Drug Administration
- Search
- Menu
- Vaccines, Blood & Biologics
- Science & Research (Biologics)
Vaccines Research
Bacterial polysaccharides.
- Characterization of Bacterial Glycoconjugates and Development of Associated Vaccine Technologies Wille Vann, PhD
- Evaluating the Host and Microbial Factors That Impact the Efficacy of Polysaccharide Vaccines Mustafa Akkoyunlu, MD, PhD
- Biomarkers of Vaccine Safety and Effectiveness for Encapsulated Bacterial Pathogens Margaret C. Bash, MD, MPH
- Studying the Structure of Carbohydrates in Order to Better Understand Bacterial Vaccines and Pathogens John F. Cipollo, PhD
- Improvement of Biological Product Quality by Application of New Technologies to Characterize of Vaccines and Blood Products: NMR Spectroscopy and Light Scattering Darón I. Freedberg, PhD
Mucosal Pathogens and Cellular Immunology
- Novel Methods to Detect Malaria Biomarkers for Evaluation of Vaccine Safety and Efficacy Heather J. Painter, PhD
- Methods to Predict and Improve Efficacy of Vaccines Against Bacterial Pathogens Siobhan C. Cowley, PhD
- New Ways to Predict How Well Vaccines Protect Against Tuberculosis, Tularemia and Other Bacteria That Live Inside Cells Karen Elkins, PhD
Respiratory & Special Pathogens
- Improving Evaluation of Bacterial Vaccines Drusilla Burns, PhD
- Developing and Evaluating Animal Models for Studying the Safety and Efficacy of Vaccines Against Anthrax, Staphylococcal Infections, and Pertussis Todd Merkel, PhD
- Biomarkers of Vaccine Safety and Efficacy for Diphtheria Vaccines Michael Schmitt, PhD
DNA and Hepatitis Viruses
- Gastrointestinal Viruses: Understanding Diversity and Immune Responses to Inform Vaccine Design Gabriel I. Parra, PhD
- Development of Quantitative Assays to Evaluate the Safety of Cell Substrates and Vaccines Keith Peden, PhD
- Development of Assays of Defined Sensitivity for the Regulatory Management of Novel Cell Substrates Andrew Lewis, MD
- New Approaches to the Development and Evaluation of Vaccines Against Hepatitis Viruses Marian Major, PhD
- DNA Virus Vaccines and Vectors Jerry Weir, PhD
Methods Development
- Evaluation of the Quality of Viral Vaccines Based on Molecular Consistency Konstantin M. Chumakov, PhD
Pediatric & Respiratory Viral Diseases
- Influenza neuraminidase antigenicity and efficacy in vaccines Robert Daniels, PhD
- Supporting the Production of Safe and Effective Influenza Vaccines Zhiping Ye, MD, PhD
- Developing Tests to Detect Evidence of Protective Immune Responses Generated by Vaccines for Respiratory Viruses That Infect Infants, Children and the Elderly Judy Beeler, MD
Retrovirus Research
- HIV Influenza And Smallpox Vaccines: Development of New Assays and Animal Models for Evaluation of Vaccine Safety and Efficacy Hana Golding, PhD
- Investigating Viruses in Cells Used to Make Vaccines; and Evaluating the Potential Threat Posed by Transmission of Viruses to Humans Arifa Khan, PhD
Vector Borne Viral Diseases
- Understanding the Interplay Between Host Immunity and Viral Factors for Rational Design of Vaccines Against Vector-Borne Viral Pathogens Tony Wang, PhD
- Evaluating New Approaches to Developing AIDS Vaccines by Creating Virus-like Particles that Display HIV Antigens or by Using Live, Attenuated (Weakened), non-HIV Viruses to Express HIV Proteins Ira Berkower, MD
- Virus Entry and Its Inhibition by Antibodies: Studies to Aid the Development and Evaluation of Vaccines that Protect against Viral Infectious Diseases Carol Weiss, MD
- U.S. Department of Health & Human Services

- Virtual Tour
- Staff Directory
- En Español
You are here
Nih research matters.
July 25, 2023
Research in Context: Progress toward universal vaccines
Preparing for the next pandemic before it starts.
This article, about the concept of universal vaccines, inaugurates a new periodic feature of NIH Research Matters called Research in Context . These in-depth articles will focus on cutting-edge NIH biomedical research topics, describing the current state of research in a field and where it may be heading.

In March 2020, the world changed in the blink of an eye as countries around the world locked down to slow the spread of COVID-19. This often deadly disease, caused by a previously unknown coronavirus called SARS-CoV-2, seeded outbreaks across the globe in record time. By the end of 2022, it had killed more than 6 million people worldwide.
But COVID-19 was far from humanity’s first pandemic. As recently as 15 years ago, an outbreak of pandemic flu sickened 60 million people globally. In 2003, a new disease called severe acute respiratory syndrome, or SARS, sickened more than 8,000 people worldwide. It was caused by a virus called SARS-associated coronavirus (SARS-CoV). No one can predict when the next pandemic will happen—only that one eventually will.
These recent pandemics have brought into stark relief the need to be prepared for the next emerging disease, whenever it arrives. To this end, NIH-funded research teams have been working to develop universal vaccines against diseases with pandemic potential. Unlike current vaccines, which confer immunity to one or several strains of a disease, universal vaccines are designed to teach the immune system to defend against all versions of a pathogen—even versions that don’t exist yet. They do this by targeting an element of the pathogen that remains the same across all strains and types.
Such targets are usually those that are least accessible to the immune system. This has posed a significant challenge to vaccine researchers. But with recent progress in vaccine technology, researchers believe that universal vaccines are closer to reality than ever before.
Moving beyond educated guesses
For some viruses, the only constant is change. Locked in a continuous battle with the human immune system, many common viruses change, or mutate, rapidly. This means that even if you’ve been infected with a previous version of a virus, your immune system may not recognize an altered version the next time around.
A well-known example of the arms race between viruses and humans is the influenza virus, commonly known as the flu. More than 20 types of the virus—each of which, in turn, contains many different strains—circulate among people and animals, changing almost constantly.
The flu vaccine you get every year targets four strains that the scientific community predicts are most likely to predominate that season. “We have a well-established system to collect [information] on which strains are circulating all over the world,” says NIH vaccine researcher Dr. Karin Bok. “But it takes at least six months from the decision of which [strains] to include to the vaccine being available to the public.” And which flu strains circulate during that time can change unpredictably.
As a result, seasonal flu vaccines vary in their effectiveness. Their ability to prevent severe disease ranges from as high as 60% to as low as 10%.
All widely used flu vaccines to date teach the immune system to recognize a protein called hemagglutinin, which is found on the surface of the influenza virus. The virus uses hemagglutinin to enter human cells.
In a recent NIH-funded study, researchers designed a flu vaccine to provide broad protection against different influenza viruses. To create the vaccine, the researchers fused hemagglutinin to building blocks that assemble into nanometer-sized particles, or nanoparticles. The nanoparticles included hemagglutinin from four different flu strains. The researchers reasoned that this would encourage the immune system to respond to parts of the protein that were more similar, or conserved, between influenza strains.
“What these nanoparticles do is repetitively display the antigen—the protein from the virus—that you’re trying to mount an immune response to,” explains Dr. Neil King of UW Medicine, who helped lead the study along with researchers from NIH’s Vaccine Research Center (VRC). “And repetition…tells the immune system that this is something dangerous.” This approach can create a strong immune memory of the conserved part of these viral proteins.
In studies in mice, ferrets, and monkeys, the nanoparticle vaccines induced antibody responses against the included strains that were as good as or better than those elicited by a commercial vaccine. Notably, the nanoparticle vaccines also provided near-complete protection against several related flu strains that were not included in the nanoparticles. In contrast, the commercial vaccine did not protect against those other strains.
“These nanoparticle vaccines may be what we call a “supra-seasonal vaccine”—a vaccine that protects for more than one year,” King says.
The vaccine, called FluMos-v1, is now in phase 1 clinical trials .
VRC researchers have also been working on another vaccine that may provoke an even broader immune response to influenza. The team based their approach on the structure of hemagglutinin, which consists of a stem and a head. Flu vaccines to date have targeted the head of the protein, which is most accessible to immune cells. But this is also the part of the protein that mutates fastest.
The new VRC vaccine, which has completed an early-phase human trial, uses a nanoparticle to display the hemagglutinin stem without the head. The hemagglutinin stem tends to remain relatively unchanged, even as the head rapidly changes. The trial found that immunization with this vaccine was safe and elicited immune responses to a range of hemagglutinins that lasted more than a year after vaccination.
Showing the immune system the stem has an added advantage, Bok explains: it’s not something the immune system is used to seeing. This novelty provokes a stronger immune response. That, plus the conserved nature of the stem, “may make it so you would be able to mount an immune response to any hemagglutinin you’re exposed to [after vaccination],” Bok says.
A pressing need for universal protection
The nanoparticle technologies pioneered to develop universal flu vaccines are now being tested to create vaccines that could protect against multiple current and future coronaviruses, including SARS-CoV-2.
“SARS-CoV-2 has proven itself capable of making new variants that are prolonging the global COVID-19 pandemic,” says Dr. Pamela Bjorkman, who leads an NIH-funded research team at the California Institute of Technology. And SARS-CoV-2 wasn’t the first virus of its kind—a type called a betacoronavirus—to jump from animals to people. SARS-CoV came before, and so did MERS-CoV, which causes the deadly Middle East Respiratory Syndrome (MERS).

“The fact that three betacoronaviruses—SARS-CoV, MERS-CoV, and SARS-CoV-2—have spilled over into humans from animal hosts in the last 20 years illustrates the need for making broadly protective vaccines,” she says.
In a recent study, Bjorkman and her team combined pieces of the spike proteins from eight different coronaviruses into a new nanoparticle vaccine. The portion of the spike protein they used is called the receptor binding domain, or RBD. Coronaviruses use the RBD to enter human cells.
Each nanoparticle included 60 RBDs, so that any two adjacent ones were rarely from the same coronavirus. As with flu vaccines, this arrangement encourages antibody-producing immune cells to target areas that are similar across the proteins.
The team tested the new vaccine in mice engineered to be vulnerable to SARS-CoV-2. Following vaccination, the mice produced antibodies that recognized a range of different coronaviruses. And as expected, the antibodies recognized parts of the spike protein that remained similar between coronaviruses.
Promising results were also seen when the vaccine was tested in monkeys. The animals were protected not only against a SARS-CoV-2 variant that wasn’t included in the vaccine but also against SARS-CoV.
“We can’t predict which virus or viruses among the vast numbers in animals will evolve in the future to infect humans to cause another epidemic or pandemic,” Bjorkman explains. “What we’re trying to do is make an all-in-one vaccine protective against SARS-like coronaviruses. This sort of vaccine would also protect against current and future SARS-CoV-2 variants without the need for updating.”
The arrival of mRNA vaccines
Another tool being tested to create more broadly effective vaccines is mRNA technology. This technology enabled COVID-19 vaccines to be developed and brought to the clinic within less than a year after the genome of SARS-CoV-2 was sequenced.
Traditionally, vaccines used weakened or killed versions of an actual pathogen, Bok explains. As technology improved, more refined vaccines were made that included only the pathogen proteins that interact with human cells. mRNA vaccines are very similar, Bok says.
“You’re still getting the same protein,” she explains. “It’s just the delivery mechanism that’s different. Instead of giving you the protein, it’s giving [your body] the source code—the software—so you can make [that protein] yourself.”
This approach allows the immune system to be exposed to substantially greater quantities of a protein than with a traditional vaccine. That, in turn, can produce a stronger immune response. Another advantage of mRNA vaccines is that they can be much cheaper to produce and easier to modify quickly. These vaccines are now being tested to prevent a variety of illnesses beyond SARS-CoV-2, including influenza.
An NIH-funded research team led by Dr. Scott Hensley from the University of Pennsylvania designed a vaccine that includes mRNAs for hemagglutinin from all 20 influenza types known to infect people. It hadn’t been possible to include so much variation with traditional vaccine production methods. But the researchers thought it might work with mRNA technology.
In animal tests, mice that received the experimental mRNA vaccine produced antibodies against both similar and unique regions of all 20 different types of hemagglutinin. Levels of these antibodies remained unchanged for months after vaccination. This robust antibody production occurred whether or not the mice had previously been exposed to one of the flu strains.
Further experiments showed that vaccination protected both mice and ferrets from a dangerous flu strain similar to one of those in the vaccine.
“For a conventional vaccine, immunizing against all these types would be a major challenge, but with mRNA technology it’s relatively easy,” Hensley says. “The idea here is to have a vaccine that will give people a baseline level of immune memory to diverse flu strains, so that there will be far less disease and death when the next flu pandemic occurs.”
“When we think about pandemic preparedness,” Bok says, “what we’re most worried about is the first few months, before vaccines can be prepared. Universal vaccines, along with antivirals and other treatments, could provide [vital] protection against severe disease from the next pandemic.”
—by Sharon Reynolds
Related Links
- Bivalent Boosters Provide Better Protection Against Severe COVID-19
- Using mRNA Technology for a Universal Flu Vaccine
- Fighting Current and Future Coronaviruses with a Single Vaccine
- Experimental Vaccine Protects against Multiple Coronaviruses
- Nanoparticle Vaccine against Various Coronaviruses
- Nanoparticle-Based Flu Vaccine
- Universal Mosquito Vaccine Tested
- Newly Identified Flu Antibodies Could Improve Vaccines and Treatments
- Universal Influenza Vaccine Research
- Pandemic Preparedness
References: Quadrivalent influenza nanoparticle vaccines induce broad protection. Boyoglu-Barnum S, Ellis D, Gillespie RA, Hutchinson GB, Park YJ, Moin SM, Acton OJ, Ravichandran R, Murphy M, Pettie D, Matheson N, Carter L, Creanga A, Watson MJ, Kephart S, Ataca S, Vaile JR, Ueda G, Crank MC, Stewart L, Lee KK, Guttman M, Baker D, Mascola JR, Veesler D, Graham BS, King NP, Kanekiyo M. Nature . 2021 Apr;592(7855):623-628. doi: 10.1038/s41586-021-03365-x. Epub 2021 Mar 24. PMID: 33762730. A multivalent nucleoside-modified mRNA vaccine against all known influenza virus subtypes. Arevalo CP, Bolton MJ, Le Sage V, Ye N, Furey C, Muramatsu H, Alameh MG, Pardi N, Drapeau EM, Parkhouse K, Garretson T, Morris JS, Moncla LH, Tam YK, Fan SHY, Lakdawala SS, Weissman D, Hensley SE. Science . 2022 Nov 25;378(6622):899-904. doi: 10.1126/science.abm0271. Epub 2022 Nov 24. PMID: 36423275. Mosaic RBD nanoparticles protect against challenge by diverse sarbecoviruses in animal models. Cohen AA, van Doremalen N, Greaney AJ, Andersen H, Sharma A, Starr TN, Keeffe JR, Fan C, Schulz JE, Gnanapragasam PNP, Kakutani LM, West AP Jr, Saturday G, Lee YE, Gao H, Jette CA, Lewis MG, Tan TK, Townsend AR, Bloom JD, Munster VJ, Bjorkman PJ. Science . 2022 Aug 5;377(6606):eabq0839. doi: 10.1126/science.abq0839. Epub 2022 Aug 5. PMID: 35857620. An influenza hemagglutinin stem nanoparticle vaccine induces cross-group 1 neutralizing antibodies in healthy adults. Widge AT, Hofstetter AR, Houser KV, Awan SF, Chen GL, Burgos Florez MC, Berkowitz NM, Mendoza F, Hendel CS, Holman LA, Gordon IJ, Apte P, Liang CJ, Gaudinski MR, Coates EE, Strom L, Wycuff D, Vazquez S, Stein JA, Gall JG, Adams WC, Carlton K, Gillespie RA, Creanga A, Crank MC, Andrews SF, Castro M, Serebryannyy LA, Narpala SR, Hatcher C, Lin BC, O'Connell S, Freyn AW, Rosado VC, Nachbagauer R, Palese P, Kanekiyo M, McDermott AB, Koup RA, Dropulic LK, Graham BS, Mascola JR, Ledgerwood JE; VRC 321 study team. Sci Transl Med . 2023 Apr 19;15(692):eade4790. doi: 10.1126/scitranslmed.ade4790. Epub 2023 Apr 19. PMID: 37075129. An influenza H1 hemagglutinin stem-only immunogen elicits a broadly cross-reactive B cell response in humans. Andrews SF, Cominsky LY, Shimberg GD, Gillespie RA, Gorman J, Raab JE, Brand J, Creanga A, Gajjala SR, Narpala S, Cheung CSF, Harris DR, Zhou T, Gordon I, Holman L, Mendoza F, Houser KV, Chen GL, Mascola JR, Graham BS, Kwong PD, Widge A, Dropulic LK, Ledgerwood JE, Kanekiyo M, McDermott AB. Sci Transl Med . 2023 Apr 19;15(692):eade4976. doi: 10.1126/scitranslmed.ade4976. Epub 2023 Apr 19. PMID: 37075126.
Connect with Us
- More Social Media from NIH
Thank you for visiting nature.com. You are using a browser version with limited support for CSS. To obtain the best experience, we recommend you use a more up to date browser (or turn off compatibility mode in Internet Explorer). In the meantime, to ensure continued support, we are displaying the site without styles and JavaScript.
- View all journals
- Explore content
- About the journal
- Publish with us
- Sign up for alerts
- Review Article
- Published: 12 April 2021
Vaccine development for emerging infectious diseases
- Jean-Louis Excler ORCID: orcid.org/0000-0002-6462-5101 1 ,
- Melanie Saville 2 ,
- Seth Berkley 3 &
- Jerome H. Kim ORCID: orcid.org/0000-0003-0461-6438 1
Nature Medicine volume 27 , pages 591–600 ( 2021 ) Cite this article
76k Accesses
237 Citations
93 Altmetric
Metrics details
- Viral infection
Examination of the vaccine strategies and technical platforms used for the COVID-19 pandemic in the context of those used for previous emerging and reemerging infectious diseases and pandemics may offer some mutually beneficial lessons. The unprecedented scale and rapidity of dissemination of recent emerging infectious diseases pose new challenges for vaccine developers, regulators, health authorities and political constituencies. Vaccine manufacturing and distribution are complex and challenging. While speed is essential, clinical development to emergency use authorization and licensure, pharmacovigilance of vaccine safety and surveillance of virus variants are also critical. Access to vaccines and vaccination needs to be prioritized in low- and middle-income countries. The combination of these factors will weigh heavily on the ultimate success of efforts to bring the current and any future emerging infectious disease pandemics to a close.
Similar content being viewed by others
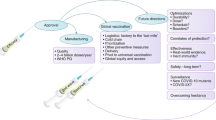
Looking beyond COVID-19 vaccine phase 3 trials
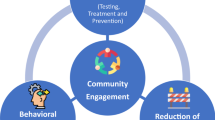
Leveraging lessons learned from the COVID-19 pandemic for HIV
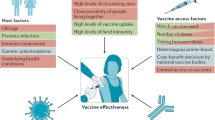
Progress of the COVID-19 vaccine effort: viruses, vaccines and variants versus efficacy, effectiveness and escape
Newly emerging and reemerging infectious viral diseases have threatened humanity throughout history. Several interlaced and synergistic factors including demographic trends and high-density urbanization, modernization favoring high mobility of people by all modes of transportation, large gatherings, altered human behaviors, environmental changes with modification of ecosystems and inadequate global public health mechanisms have accelerated both the emergence and spread of animal viruses as existential human threats. In 1918, at the time of the ‘Spanish flu’, the world population was estimated at 1.8 billion. It is projected to reach 9.9 billion by 2050, an increase of more than 25% from the current 2020 population of 7.8 billion ( https://www.worldometers.info ). The novel severe acute respiratory syndrome coronavirus 2 (SARS-CoV-2) responsible for the coronavirus disease 2019 (COVID-19) pandemic 1 , 2 , 3 engulfed the entire world in less than 6 months, with high mortality in the elderly and those with associated comorbidities. The pandemic has severely disrupted the world economy. Short of lockdowns, the only means of control have been limited to series of mitigation measures such as self-distancing, wearing masks, travel restrictions and avoiding gatherings, all imperfect and constraining. Now with more than 100 million people infected and more than 2 million deaths, it seems that the addition of vaccine(s) to existing countermeasures holds the best hope for pandemic control. Taken together, these reasons compel researchers and policymakers to be vigilant, reexamine the approach to surveillance and management of emerging infectious disease threats, and revisit global mechanisms for the control of pandemic disease 4 , 5 .
Emerging and reemerging infectious diseases
The appearance of new infectious diseases has been recognized for millennia, well before the discovery of causative infectious agents. Despite advances in development of countermeasures (diagnostics, therapeutics and vaccines), world travel and increased global interdependence have added layers of complexity to containing these infectious diseases. Emerging infectious diseases (EIDs) are threats to human health and global stability 6 , 7 . A review of emerging pandemic diseases throughout history offers a perspective on the emergence and characteristics of coronavirus epidemics, with emphasis on the SARS-CoV-2 pandemic 8 , 9 . As human societies grow in size and complexity, an endless variety of opportunities is created for infectious agents to emerge into the unfilled ecologic niches we continue to create. To illustrate this constant vulnerability of populations to emerging and reemerging pathogens and their respective risks to rapidly evolve into devastating outbreaks and pandemics, a partial list of emerging viral infectious diseases that occurred between 1900 and 2020 is shown in Table 1 .
Although nonemerging infectious diseases (not listed in Table 1 ), two other major mosquito-borne viral infections are yellow fever and dengue. Yellow fever, known for centuries and an Aedes mosquito-borne disease, is endemic in more than 40 countries across Africa and South America. Since 2016, several yellow fever outbreaks have occurred in Angola, Democratic Republic of Congo, Nigeria and Brazil to cite a few 10 , raising major concerns about the adequacy of yellow fever vaccine supply. Four live attenuated vaccines derived from the live attenuated yellow fever strain (17D) 11 and prequalified by the WHO (World Health Organization) are available 12 .
Dengue is an increasing global public health threat with the four dengue virus types (DENV1–4) now cocirculating in most dengue endemic areas. Population growth, an expansion of areas hospitable for Aedes mosquito species and the ease of travel have all contributed to a steady rise in dengue infections and disease. Dengue is common in more than 100 countries around the world. Each year, up to 400 million people acquire dengue. Approximately 100 million people get sick from infection, and 22,000 die from severe dengue. Most seriously affected by outbreaks are the Americas, South/Southeast Asia and the Western Pacific; Asia represents ~70% of the global burden of disease ( https://www.cdc.gov/dengue ). Several vaccines have been developed 13 . A single dengue vaccine, Sanofi Pasteur’s Dengvaxia based on the yellow fever 17D backbone, has been licensed in 20 countries, but uptake has been poor. A safety signal in dengue-seronegative vaccine recipients stimulated an international review of the vaccine performance profile, new WHO recommendations for use and controversy in the Philippines involving the government, regulatory agencies, Sanofi Pasteur, clinicians responsible for testing and administering the vaccine, and the parents of vaccinated children 14 .
Two bacterial diseases, old scourges of humanity, are endemic and responsible for recurrent outbreaks and are increasingly antimicrobial resistant. Cholera, caused by pathogenic strains of Vibrio cholerae , is currently in its seventh global pandemic since 1817; notably, the seventh pandemic started in 1961 15 . Global mortality due to cholera infection remains high, mainly due to delay in rehydrating patients. The global burden of cholera is estimated to be between 1.4 and 4.3 million cases with about 21,000–143,000 deaths per year, mostly in Asia and Africa. Tragic outbreaks have occurred in Yemen and Haiti. Adding to rehydration therapy, antibiotics have been used in the treatment of cholera to shorten the duration of diarrhea and to limit bacterial spread. Over the years, antimicrobial resistance developed in Asia and Africa to many useful antibiotics including chloramphenicol, furazolidone, trimethoprim-sulfamethoxazole, nalidixic acid, tetracycline and fluoroquinolones. Several vaccines have been developed and WHO prequalified; these vaccines constitute a Gavi-supported global stockpile for rapid deployment during outbreaks 16 .
Typhoid fever is a severe disease caused by the Gram-negative bacterium Salmonella enterica subsp. enterica serovar Typhi ( S . Typhi). Antimicrobial-resistant S . Typhi strains have become increasingly common. The first large-scale emergence and spread of a novel extensively drug-resistant (XDR) S . Typhi clone was first reported in Sindh, Pakistan 17 , 18 , and has subsequently been reported in India, Bangladesh, Nepal, the Philippines, Iraq and Guatemala 19 , 20 . The world is in a critical period as XDR S . Typhi has appeared in densely populated areas. The successful development of improved typhoid vaccines (conjugation of the Vi polysaccharide with a carrier protein) with increased immunogenicity and efficacy including in children less than 2 years of age will facilitate the control of typhoid, in particular in XDR areas by decreasing the incidence of typhoid fever cases needing antibiotic treatment 21 , 22 .
A model of vaccine development for emerging infectious diseases
The understanding of emerging infectious diseases has evolved over the past two decades. A look back at the SARS-CoV outbreak in 2002 shows that—despite a small number of deaths and infections—its high mortality and transmissibility caused significant global disruption (see Table 1 ). The epidemic ended as work on vaccines was initiated. Since then, the disease has not reappeared—wet markets were closed and transmission to humans from civets ceased. Consequently, work on vaccines against SARS-CoV ended and its funding was cut. Only a whole inactivated vaccine 23 and a DNA vaccine 24 were tested in phase 1 clinical trials.
Following a traditional research and development pipeline, it takes between 5 and 10 years to develop a vaccine for an infectious agent. This approach is not well suited for the needs imposed by the emergence of a new pathogen during an epidemic. Figure 1 shows a comparison of the epidemic curves and vaccine development timelines between the 2014 West African Ebola outbreak and COVID-19. The 2014 Ebola epidemic lasted more than 24 months with 11,325 deaths and was sufficiently prolonged to enable the development and testing of vaccines for Ebola, with efficacy being shown for one vaccine (of several) toward the end of the epidemic 25 , 26 . What makes the COVID-19 pandemic remarkable is that the whole research and development pipeline, from the first SARS-CoV-2 viral sequenced to interim analyses of vaccine efficacy trials, was accomplished in just under 300 days 27 . Amid increasing concerns about unmitigated transmission during the 2013–2016 Western African Ebola outbreak in mid-2014, WHO urged acceleration of the development and evaluation of candidate vaccines 25 . To ensure that manufacturers would take the Ebola vaccine to full development and deployment, Gavi, the Vaccine Alliance, publicly announced support of up to US$300 million for vaccine purchase and followed that announcement with an advance purchase agreement. Ironically, there had been Ebola vaccines previously developed and tested for biodefense purposes in nonhuman primates, but this previous work was neither ‘ready’ for clinical trials during the epidemic nor considered commercially attractive enough to finish development 28 .
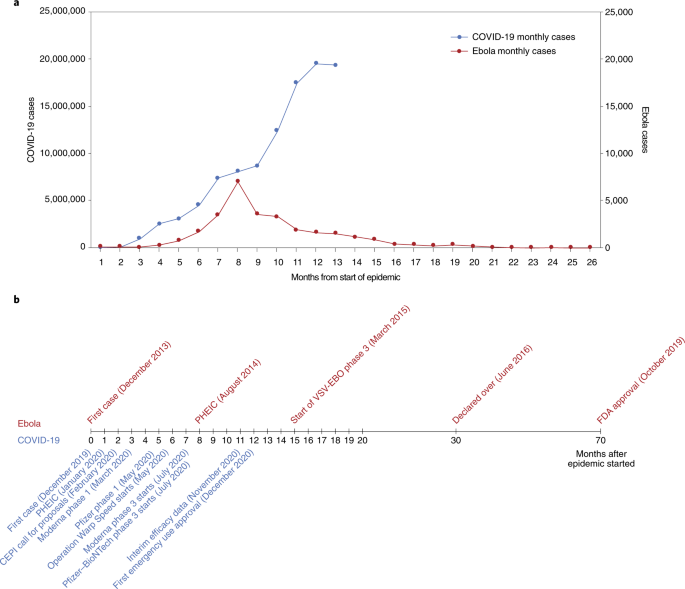
a , The number of months from the onset of the epidemic is shown against the number of reported cases per day. Note that the COVID-19 (left) and Ebola (right) axes are scaled differently. b , Vaccine development timelines for COVID-19 versus Ebola in the context of particular events during the respective outbreaks. PHEIC, public health emergency of international concern.
From these perceived shortcomings in vaccine development during public health emergencies arose the Coalition for Epidemic Preparedness Innovations (CEPI), a not-for-profit organization dedicated to timely vaccine development capabilities in anticipation of epidemics 29 , 30 . CEPI initially focused on diseases chosen from a list of WHO priority pathogens for EIDs—Middle East respiratory syndrome (MERS), Lassa fever, Nipah, Rift Valley fever (RVF) and chikungunya. The goal of CEPI was to advance candidate vaccines through phase 2 and to prepare stockpiles of vaccine against eventual use/testing under epidemic circumstances. CEPI had also prepared for ‘disease X’ by investing in innovative rapid response platforms that could move from sequence to clinical trials in weeks rather than months or years, such as mRNA and DNA technology, platforms that were useful when COVID-19 was declared a global health emergency in January 2020, and a pandemic in March 2020 31 , 32 .
CEPI has been able to fund several vaccine development efforts, among them product development by Moderna, Inovio, Oxford–AstraZeneca and Novavax. Providing upfront funding helped these groups to advance vaccine candidates to clinical trials and develop scaled manufacturing processes in parallel, minimizing financial risk to vaccine developers. The launch of the larger US-funded Operation Warp Speed 33 further provided companies with funding—reducing risks associated with rapid vaccine development and securing initial commitments in vaccine doses.
Vaccine platforms and vaccines for emerging infectious diseases
Vaccines are the cornerstone of the management of infectious disease outbreaks and are the surest means to defuse pandemic and epidemic risk. The faster a vaccine is deployed, the faster an outbreak can be controlled. As discussed in the previous section, the standard vaccine development cycle is not suited to the needs of explosive pandemics. New vaccine platform technologies however may shorten that cycle and make it possible for multiple vaccines to be more rapidly developed, tested and produced 34 . Table 2 provides examples of the most important technical vaccine platforms for vaccines developed or under development for emerging viral infectious diseases. Two COVID-19 vaccines were developed using mRNA technology (Pfizer–BioNTech 35 and Moderna 36 ), both showing safety and high efficacy, and now with US Food and Drug Administration (FDA) emergency use authorization (EUA) 37 , 38 and European Medicines Agency (EMA) conditional marketing authorization 39 , 40 . While innovative and encouraging for other EIDs, it is too early to assert that mRNA vaccines represent a universal vaccine approach that could be broadly applied to other EIDs (such as bacterial or enteric pathogens). While COVID-19 mRNA vaccines are a useful proof of concept, gathering lessons from their large-scale deployment and effectiveness studies still requires more work and time.
While several DNA vaccines are licensed for veterinary applications, and DNA vaccines have shown safety and immunogenicity in human clinical trials, no DNA vaccine has reached licensure for use in humans 41 . Recombinant proteins vary greatly in design for the same pathogen (for example, subunit, virus-like particles) and are often formulated with adjuvants but have longer development times. Virus-like particle-based vaccines used for hepatitis B and human papillomavirus are safe, highly immunogenic, efficacious and easy to manufacture in large quantity. The technology is also easily transferable. Whole inactivated pathogens (for example, SARS-CoV-2, polio, cholera) or live attenuated vaccines (for example, SARS-CoV-2, polio, chikungunya) are unique to each pathogen. Depending on the pathogen, these vaccines also may require biosafety level 3 manufacturing (at least for COVID-19 and polio), which may limit the possibility of technology transfer for increasing the global manufacturing capacity.
Other vaccines are based on recombinant vector platforms, subdivided into nonreplicating vectors (for example, adenovirus 5 (Ad5), Ad26, chimpanzee adenovirus-derived ChAdOx, highly attenuated vectors like modified vaccinia Ankara (MVA)) and live attenuated vectors such as the measles-based vector or the vesicular stomatitis virus (VSV) vector. Either each vector is designed with specific inserts for the pathogen targeted, or the same vector can be designed with different inserts for the same disease. The development of the Merck Ebola vaccine is an example. ERVEBO is a live attenuated, recombinant VSV-based, chimeric-vector vaccine, where the VSV envelope G protein was deleted and replaced by the envelope glycoprotein of Zaire ebolavirus . ERVEBO is safe and highly efficacious, now approved by the US FDA and the EMA, and WHO prequalified, making VSV an attractive ‘platform’ for COVID-19 and perhaps for other EID vaccines 26 although the −70 °C ultracold chain storage requirement still presents a challenge.
Other equally important considerations are speed of development, ease of manufacture and scale-up, ease of logistics (presentation, storage conditions and administration), technology transfer to other manufacturers to ensure worldwide supply, and cost of goods. Viral vectors such as Ad5, Ad26 and MVA have been used in HIV as well as in Ebola vaccines 42 . Finally, regulatory authorities do not approve platforms but vaccines. Each vaccine is different. However, with each use of a specific technology, regulatory agencies may, over time, become more comfortable with underlying technology and the overall safety and efficacy of the vaccine platform, allowing expedited review and approvals in the context of a pandemic 43 . With COVID-19, it meant that the regulatory authorities could permit expedited review of ‘platform’ technologies, such as RNA and DNA, that had been used (for other conditions) and had safety profiles in hundreds of people.
A heterologous prime–boost (HPB) vaccine approach has been extensively explored for HIV 44 and Ebola vaccines 42 . It is being investigated for COVID-19 vaccines with the Oxford–AstraZeneca AZD1222 and Gamaleya Sputnik V COVID-19 vaccines 45 or with the Pfizer–BioNTech vaccine ( https://www.comcovstudy.org.uk ). Other HPB combinations might be considered involving mRNA, DNA, viral vector-based and protein-based vaccines. This may offer the potential benefit of improving the immune response and avoiding mutlidose reactogenicity or anti-vector immune responses. Additionally, people previously vaccinated with the standard regimen (for example, single or two dose) could be offered a booster immunization with a different vaccine. This might mitigate current shortages in vaccines, particularly in low- and middle-income countries (LMICs). Such a matrix of HPB possibilities deserves further consideration by manufacturers, funders and regulators supported by clinical trial studies and assessment of implementation challenges.
Important improvements could speed up availability. Standardized labeling of vaccines so that they can be interchanged across countries and regions, date of production rather than expiration so that shelf life can be tracked, three-dimensional bar coding to allow critical information to be updated, standard indemnification and liability language that would allow agreement with all manufacturers, a no-fault compensation mechanism for serious adverse events related to vaccine administration, and regulatory harmonization are all critical and being worked on as part of the COVID-19 vaccine response and must be optimized for future outbreaks.
The pathway to EUA, licensure and beyond
Big pharmaceutical or biotechnology companies supported by organizations such as CEPI or efforts such as Operation Warp Speed have conducted efficacy trials in countries or regions with the highest SARS-CoV-2 incidence rates. The same groups have also committed funding for large-scale manufacturing at risk. With more than 60 vaccine candidates in clinical trials and another 170 in preclinical development (WHO COVID-19 vaccine landscape) 46 , it is uncertain whether vaccine candidates not in the first wave of testing/approvals will be able to progress to EUA and licensure based solely on results of randomized clinical efficacy trials with clinical endpoints. Regulators and ethics committees may decide that noninferiority clinical trials against comparator vaccines with proven clinical efficacy will be needed for further approvals. Would the demonstration of equivalence between immune responses generated by a new vaccine and those of a clinically proven efficacious vaccine (bridging studies) 47 be accepted by regulatory authorities and replace the need for noninferiority clinical endpoint studies? For that to occur there must be agreement on what are immune correlates of protection (ICP) to COVID-19, and these have yet to be identified. Moreover, it is not yet clear that ICP will translate equally between different vaccine platforms; for example, are immune responses generated by chimpanzee adenovirus the same as those generated by proteins or whole inactivated virus? As incidence rates of a disease decrease over time due to sustained mitigation measures and implementation of vaccination, larger sample sizes in multicountry trials, additional participant accrual time and complex logistics will likely be required for future approvals, compromising the speed of clinical development and increasing cost. Early deployment of scarce doses of still-investigational vaccines (under emergency use listing (EUL) or similar regulatory mechanisms) could bring additional public health benefits if accompanied by firm commitment to maintaining blinded follow-up of participants in ongoing or future placebo-controlled trials until a licensed vaccine is fully deployed in the population 48 .
Randomized controlled trials might underestimate the protective effect of vaccines at the population level. This would occur if the COVID-19 vaccine, in addition to conferring direct protection to individuals, reduces transmission of COVID-19 between individuals, providing protection to unvaccinated individuals and enhanced protection of vaccinated individuals in contact with vaccinated individuals. Vaccine-induced herd protection, which might be crucial to the public health value of a vaccine, will be missed when trials are individually randomized and analyses fail to take account of the geographical distribution of individuals in the population 49 . For these reasons, other clinical trial designs have been proposed once COVID-19 vaccines have achieved licensure via current phase 3 trials to assess how useful the vaccines will be in practice and addressing vaccine effectiveness, including the level of protection of both vaccinated and nonvaccinated individuals in targeted populations 50 .
In the particular context of the COVID-19 pandemic, whether regulatory authorities would require clinical endpoints in future efficacy trials or would consider ICP remains unclear. Clinical endpoints provide increased accuracy with regard to definitive clinical outcomes where outcome-related analyses using ICP are inferential. ICP will contribute to our understanding of viral pathogenesis and immunity, be useful for future approval of vaccines, and help in our understanding of waning of protective immunity following vaccination or infection. The paradox is that the higher the efficacy, the more difficult it will be to identify these correlates because there may not be enough infected vaccine recipients to compare with uninfected vaccine recipients. The analysis of ICP may be possible only in clinical trials showing a lower vaccine efficacy 50 . They would also not provide a rigorous evaluation of long-term safety and the potential for vaccine-associated enhanced respiratory disease 51 .
Pharmacovigilance and surveillance
In May 2020, the 42nd Global Advisory Committee on Vaccine Safety addressed pharmacovigilance preparedness for the launch of the future COVID-19 vaccines 52 . One of their recommendations was that infrastructure and capacity for surveillance of the safety of COVID-19 vaccines should be in place in all countries and engaged before a vaccine is introduced. The WHO’s COVID-19 vaccine safety surveillance manual develops the monitoring and reporting of adverse events following immunization and adverse events of special interest, data management systems and safety communication, and the need for postauthorization safety surveillance studies 53 . One critical element of this surveillance is the duration of the observation period. The implementation of this surveillance will require local, national, regional and global collaboration. While countries should include preparedness plans for COVID-19 vaccine safety in their overall plans for vaccine introduction, building on WHO guidance, it is imperative that the COVID-19 Vaccines Global Access (COVAX) initiative (coordinated by CEPI, Gavi, the Vaccine Alliance, and WHO) works with partners on capacity building and the practical aspects of implementation with technical and training support tailored to the settings.
In view of the public health urgency and the extensive vaccination campaigns foreseen worldwide, the EMA and the national competent authorities in EU member states have prepared themselves for the expected high data volume by putting pharmacovigilance plans specific for COVID-19 vaccines in place. Good pharmacovigilance practices include detailed requirements and guidance on the principles of a risk management plan (RMP) and requirements for vaccines. In addition, core RMP requirements for COVID-19 vaccines have been developed to facilitate and harmonize the preparation of RMPs by companies and their evaluation by assessors. The RMP preparation addresses the planning of the postauthorization safety follow-up of COVID-19 vaccines by marketing authorization holders, while acknowledging uncertainties in the pandemic setting and recommending ways to prepare for pharmacovigilance activities 54 . Similarly, the US Advisory Committee on Immunization Practices (ACIP) initially convened the COVID-19 Vaccine Safety Technical Working Group in June 2020 to advise the ACIP COVID-19 Vaccine Workgroup and the full ACIP on the safety monitoring of COVID-19 vaccines under development and pharmacovigilance postapproval 55 .
Key lessons could be learnt from past situations where new vaccines were introduced in response to pandemic and epidemic emergencies. For the 2009 H1N1 influenza pandemic, few countries had a pandemic preparedness plan that comprehensively addressed vaccine deployment and monitoring of adverse events. The African Vaccine Regulatory Forum, a regional network of regulators and ethics committees, working closely with regulators from other parts of the world, participated in the review of clinical trial protocols and results, the joint monitoring of trials and the joint authorization and deployment of vaccines 56 . Such models can be used to guide pharmacovigilance for the deployment of COVID-19 vaccines, particularly in LMICs with limited resources. The introduction of the first licensed dengue vaccine, while not in the context of an international public health emergency, illustrated a number of lessons for the pharmacovigilance of newly introduced vaccines, particularly the vaccine-associated enhanced disease that was observed 13 , 14 . Due to the significant sequence homology between SARS-CoV-2 and SARS-CoV, antibody-dependent enhancement (ADE) and vaccine-associated enhanced respiratory disease (VAERD) were raised as potential safety issues 57 , 58 . VAERD and ADE have not been described in current reports of SARS-CoV-2 vaccine phase 3 trials. Similarly, VAERD has not been reported in animal challenge studies with SARS-CoV-2 vaccines that conferred protection 50 . With ADE the effect of waning antibody titers after vaccination (or after infection) and potential safety signals are unknown, which emphasizes the importance of follow-up monitoring 57 .
Pregnant women seem to be disproportionately affected during pandemics and emerging pathogen outbreaks 59 , 60 . The Pregnancy Research Ethics for Vaccines, Epidemics, and New Technologies (PREVENT) Working Group has published a roadmap to guide the inclusion of the interests of pregnant women in the development and deployment of vaccines against emerging pathogens 61 , 62 .
Equally important is the surveillance on SARS-CoV-2 circulating strains as well as of other coronaviruses (MERS, seasonal) 63 . SARS-CoV-2 is evolving, with new lineages being reported all over the world. Amongst previous lineages, D614G was shown to have faster growth in vitro and enhanced transmission in small animals, and has subsequently become globally dominant 64 , 65 , 66 . Other variants of concern have been described in the UK (B.1.1.7) 67 and in Brazil (B.1.1.28.1/P1) 68 with higher capacity for transmission and, potentially, lethality. N501Y (B.1.351) isolated in South Africa has an increased affinity for the human ACE2 receptor, which together with the repeated and independent evolution of 501Y-containing lineages 69 strongly argues for enhanced transmissibility. The B.1.351 variant has nine spike alterations; it rapidly emerged in South Africa during the second half of 2020 and has shown resistance to neutralizing antibodies elicited by infection and vaccination with previously circulating lineages. The AstraZeneca COVID-19 vaccine rollout in South Africa was recently halted after the analysis showed minimal efficacy against mild and moderate cases due to B.1.351, which accounts for 90% of the cases in this country 70 . The Novavax vaccine efficacy is 86% against the variant identified in the UK and 60% against the variant identified in South Africa 71 . The efficacy of a single dose of Johnson & Johnson’s Ad26 was 57% against moderate to severe COVID-19 infection in South Africa 72 .
For the many people who have already been infected with SARS-CoV-2 globally and are presumed to have accumulated some level of immunity, new variants such as B.1.351 pose a significant reinfection risk, although vaccine-induced cell-mediated immune responses might mitigate this risk. Scientists do not know how variant lineages will evolve under vaccine-induced immune pressure during the vaccination rollout or whether choices that alter the schedule or dose may impact virus evolution. Whether vaccines efficacious against current circulating strains including the variants identified in the UK and Brazil will keep their efficacy against emerging variants is unknown and deserves enhanced global COVID-19 surveillance in both humans and animals, similar to those developed for influenza. Global influenza surveillance has been conducted through WHO’s Global Influenza Surveillance and Response System since 1952. The Global Influenza Surveillance and Response System is a global mechanism of surveillance, preparedness and response for seasonal, pandemic and zoonotic influenza, a global platform for monitoring influenza epidemiology and disease, and a global alert system for novel influenza viruses and other respiratory pathogens 73 . The Global Initiative on Sharing Avian Influenza Database ( https://www.gisaid.org ) promotes the rapid sharing of data from all influenza viruses and the coronavirus causing COVID-19. These include genetic sequence and related clinical and epidemiological data associated with human viruses, and geographical as well as species-specific data associated with avian and other animal viruses. This molecular epidemiology surveillance should be expanded to all EIDs, particularly the deadliest and most transmissible, as recently described for Ebola 25 . As with influenza, preparations for SARS-CoV-2 vaccine variants should be proactive, with a view that platforms such as mRNA could generate new vaccine strains very rapidly. A clear regulatory pathway for strain change needs discussion with the regulators.
Approval process for licensure and EUA and the risk of speed
Vaccines are classically approved by the country’s national regulatory authority such as the US FDA or by a centralized procedure through the EMA. Once approved for licensure by a stringent or functional national regulatory authority in the country of manufacture, the manufacturing company can submit a dossier for WHO prequalification. However, for SARS-CoV-2 vaccines intended for COVAX, WHO prequalification is not required for initial use if they have received WHO EUL. COVAX is one of three pillars of the Access to COVID-19 Tools Accelerator, which was launched in April 2020 by the WHO, the EC (European Commission) and France. Vaccines receiving WHO EUL can be purchased by UNICEF (United Nations International Children’s Emergency Fund), the largest purchaser of vaccines for Gavi, the Vaccine Alliance. Countries participating in COVAX can access the vaccines through the COVAX Facility either as 1 of the 98 self-financing countries or, for the 92 LMICs, funded through the Gavi COVAX advance market commitment (AMC; https://www.gavi.org ).
In the current pandemic situation, the US FDA is using the EUA process to allow initial use of the vaccines from Pfizer, Moderna and Johnson & Johnson 74 . EMA is taking the approach of conditional approval 75 . The WHO emergency use assessment and listing (EUAL) procedure was developed in the wake of the Ebola virus disease outbreak in Africa to expedite the availability of vaccines. The EUAL was intended as guidance for national regulatory authorities in circumstances when the “community may be more willing to tolerate less certainty about the efficacy and safety of products, given the morbidity and/or mortality of the disease and the shortfall of treatment and/or prevention options” 76 . In early 2020, the WHO issued a revised EUL procedure to assess whether submitted data demonstrate a reasonable likelihood that a vaccine’s quality, safety and performance are acceptable and that the benefits outweigh the foreseeable risks and uncertainties in the context of a public health emergency of international concern 77 . It is intended that vaccines approved through EUAL would eventually go to full review and receive prequalification. WHO member states have the prerogative through their national regulatory authority to use the EUL procedure to authorize the use of unlicensed vaccines.
Some countries have used their national regulatory authorities to secure approval of nationally produced vaccines. The Russian government approved the Ad26 and Ad5 combination-based COVID-19 vaccine, Sputnik V, produced by the Gamaleya Research Institute, for use by individuals aged 60 years and above 78 , 79 . China’s National Medical Products Agency has given conditional approval to the whole inactivated virus BBIBP-CorV COVID-19 vaccine developed by the Beijing Institute of Biological Products, a Sinopharm subsidiary 80 . The authorization allows the general public’s use of the inoculation and comes after the company announced that its vaccine proved 79.3% effective in phase 3 trials 81 . Although the interim results are not yet published, they must have been reviewed and approved by the Chinese Center for Disease Control and Prevention and National Medical Products Agency. The United Arab Emirates was first to approve the Sinopharm vaccine for EUA in early December 2020 based on interim analysis results 82 . The Sinovac CoronaVac vaccine was recently granted conditional approval on the basis of interim efficacy results 83 . The CanSinoBIO COVID-19 vaccine achieved 65.7% efficacy in preventing symptomatic cases in clinical trials (unpublished). The vaccine also showed a 90.98% success rate in stopping severe disease in one of its interim analysis. The vaccine was granted EUA in Mexico and Pakistan 84 .
Manufacturing—how to make more, faster
Production and distribution of hundreds of millions of doses of COVID-19 vaccine within a year of identification of the pandemic pathogen is unprecedented, and while the principles are straightforward, the manufacturing equation is complex and prone to delay. The technical platform utilized to make a vaccine (mRNA, whole inactivated virus, vector, protein with or without adjuvant), the dosage (low, mid, high), the schedule of vaccination (single or two dose) and the manufacturer capability, capacity and reputation are all important considerations for regulators and the WHO. The initial phase of manufacturing scale-up will be a key regulator of vaccine access initially. This could potentially be impacted by vaccine nationalism and the announced bilateral agreements between manufacturers and high-income countries. Companies such as Sinopharm, the Serum Institute of India or Bharat have a huge capacity for production but must supply the gigantic markets of China and India. Delays in the production of several western 85 and Chinese COVID-19 vaccines 86 have already been reported.
The Developing Countries Vaccine Manufacturers Network (DCVMN) was established in 2000 with the mission to increase the availability and affordability of quality vaccines to protect against known and emerging infectious diseases 87 . About 70% of the global EPI vaccine supplies and about 75% procured by UN (United Nations) agencies are produced by DCVMN members 88 . Several technology transfers to DCVMN members have occurred over the past decades to significantly contribute to global health. Following an initial collaboration on the oral cholera vaccine between Sweden and VABIOTECH in Vietnam, the International Vaccine Institute improved the vaccine and then transferred the technology back to VABIOTECH and to several DCVMN members, including Shantha Biotechnics (Shanchol), India; EuBiologics (Euvichol), Republic of Korea; and Incepta (Cholvax), Bangladesh. Shanchol, Euvichol and Euvichol Plus are WHO prequalified and the major contributors to the Gavi-supported global stockpile 16 while Cholvax is marketed in Bangladesh.
For COVID-19 vaccines, several companies have licensed or contracted vaccine production to other manufacturers—AstraZeneca and Novavax with the Serum Institute (India) and SK Bioscience (Korea); Moderna with Lonza (Switzerland), Johnson & Johnson with Biological E (India); and Chinese Sinovac with Butantan (Brazil) and BioFarma (Indonesia). Hopefully the license and contract manufacturing arrangements will allow the production of sufficient doses of vaccines to provide equitable access to at-risk populations globally 89 .
Under the pressures of the pandemic, and with the need for accelerated development of COVID-19 vaccines, optimization of more practical aspects of vaccine implementation, supply and dosing was secondary to the need for rapid proof of concept. COVID-19 mRNA vaccines and the VSV-EBO Ebola vaccine from Merck have a similar requirement for ultracold chain storage. While that might be overcome by relatively simple technology, the scalability of these technologies for universal vaccination is unknown. Additional development is needed to establish the stability of vaccines at higher temperatures (Pfizer mRNA). There is evidence to suggest the presence of some protection against COVID-19 after the first dose; this is critical information not only for COVID-19 but also to frame thinking around other EID vaccines.
Leave no one behind, or the unequal access to vaccines and treatments
The 2030 Agenda for Sustainable Development has the vision to leave no one behind, particularly low-income countries. COVID-19 has seen exceptionalism at either extreme. On the one hand, COVAX aims to provide at least 2 billion doses of WHO-approved vaccine to participating countries by the end of 2021—roughly 20% of each country’s vaccination needs. A total of 92 LMICs will receive vaccine largely through an AMC arranged by Gavi 90 . It now appears that the USA will join COVAX, which recently announced that it had secured agreements for sufficient doses to meet the 2021 target 50 .
Critically, vaccinating people in LMICs will require additional vaccine purchases, at a cost estimated in billions of dollars. In purely economic terms, it appears that such an investment could have substantial benefit for the global economy 91 . On the other hand, COVAX is on track to achieve its goals and poised to start delivering vaccines, and yet no AMC countries had yet been vaccinated when tens of millions of people were already being vaccinated in high-income countries. Among high-income countries, billions of doses have been preordered, several times more than justified by their populations. Can COVAX achieve its target of providing 2 billion doses by 2021, or will manufacturing bottlenecks lead to delay that will allow the coronavirus to continue to circulate in poorer countries and prolong the pandemic? If unable to access COVID-19 vaccines in a timely manner, the 2030 Agenda for Sustainable Development, especially Sustainable Development Goal 3 focusing on health, will be difficult to achieve, and low-income countries will be under extraordinary pressure as the COVID-19 pandemic forces them further into poverty and deeper inequality.
UN Secretary-General António Guterres has again stressed that COVID-19 vaccines must be a global public good, available to everyone, everywhere. “Vaccinationalism is self-defeating and would delay a global recovery” 92 . Modeling studies suggest that if high-income countries take the first 2 billion doses of available COVID-19 vaccines without regard to equity, global COVID-19 deaths will double 93 . Ensuring that all countries have rapid, fair and equitable access to COVID-19 vaccines is the promise of COVAX.
Final remarks
The lessons of the COVID-19 pandemic need to be compiled and applied to the development of future vaccines against emerging infectious diseases and novel pandemic pathogens. The permanent threat of emerging pathogens calls for vigilance, surveillance and preparedness for vaccine development and deployment, all crosscutting activities to be conducted flawlessly between epidemiologists, scientists, developers, human and veterinary health authorities, regulators and funders. Global health stakeholders have learned something about developing vaccines efficiently: they still have much to learn about making and using them with due regard to equity and access.
Zhu, N. et al. A novel coronavirus from patients with pneumonia in China, 2019. N. Engl. J. Med. 382 , 727–733 (2020).
CAS PubMed PubMed Central Google Scholar
Huang, C. et al. Clinical features of patients infected with 2019 novel coronavirus in Wuhan, China. Lancet 395 , 497–506 (2020).
Wang, D. et al. Clinical characteristics of 138 hospitalized patients with 2019 novel coronavirus-infected pneumonia in Wuhan, China. JAMA 323 , 1061–1069 (2020).
Friedler, A. Sociocultural, behavioural and political factors shaping the COVID-19 pandemic: the need for a biocultural approach to understanding pandemics and (re)emerging pathogens. Glob. Public Health 16 , 17–35 (2021).
Article PubMed Google Scholar
Gully, P. R. Pandemics, regional outbreaks, and sudden-onset disasters. Healthc. Manage. Forum 33 , 164–169 (2020).
Morens, D. M. & Fauci, A. S. Emerging infectious diseases: threats to human health and global stability. PLoS Pathog. 9 , e1003467 (2013).
Article CAS PubMed PubMed Central Google Scholar
Marston, H. D., Folkers, G. K., Morens, D. M. & Fauci, A. S. Emerging viral diseases: confronting threats with new technologies. Sci. Transl. Med. 6 , 253ps210 (2014).
Article Google Scholar
Morens, D. M. & Fauci, A. S. Emerging pandemic diseases: how we got to COVID-19. Cell 182 , 1077–1092 (2020).
Morens, D. M., Daszak, P. & Taubenberger, J. K. Escaping Pandora’s box—another novel coronavirus. N. Engl. J. Med. 382 , 1293–1295 (2020).
Article CAS PubMed Google Scholar
Sacchetto, L., Drumond, B. P., Han, B. A., Nogueira, M. L. & Vasilakis, N. Re-emergence of yellow fever in the neotropics—quo vadis? Emerg. Top. Life Sci. 4 , 399–410 (2020).
PubMed Google Scholar
World Health Organization. Yellow fever. https://www.who.int/biologicals/vaccines/yellow_fever/en/ (2015).
Juan-Giner, A. et al. Immunogenicity and safety of fractional doses of yellow fever vaccines: a randomised, double-blind, non-inferiority trial. Lancet 397 , 119–127 (2021).
Prompetchara, E., Ketloy, C., Thomas, S. J. & Ruxrungtham, K. Dengue vaccine: global development update. Asian Pac. J. Allergy Immunol. 38 , 178–185 (2020).
PubMed CAS Google Scholar
Thomas, S. J. & Yoon, I. K. A review of Dengvaxia ® : development to deployment. Hum. Vaccin. Immunother. 15 , 2295–2314 (2019).
Article PubMed PubMed Central Google Scholar
Hu, D. et al. Origins of the current seventh cholera pandemic. Proc. Natl Acad. Sci. USA 113 , E7730–E7739 (2016).
Shaikh, H., Lynch, J., Kim, J. & Excler, J. L. Current and future cholera vaccines. Vaccine 38 , A118–A126 (2020).
Klemm, E. J. et al. Emergence of an extensively drug-resistant Salmonella enterica serovar Typhi clone harboring a promiscuous plasmid encoding resistance to fluoroquinolones and third-generation cephalosporins. mBio 9 , e00105–18 (2018).
Yousafzai, M. T. et al. Ceftriaxone-resistant Salmonella Typhi outbreak in Hyderabad City of Sindh, Pakistan: high time for the introduction of typhoid conjugate vaccine. Clin. Infect. Dis. 68 , S16–S21 (2019).
Qamar, F. N. et al. Antimicrobial resistance in typhoidal salmonella: surveillance for enteric fever in Asia project, 2016–2019. Clin. Infect. Dis. 71 , S276–S284 (2020).
Marchello, C. S., Carr, S. D. & Crump, J. A. A systematic review on antimicrobial resistance among Salmonella Typhi worldwide. Am. J. Trop. Med. Hyg. 103 , 2518–2527 (2020).
Andrews, J. R. et al. Typhoid conjugate vaccines: a new tool in the fight against antimicrobial resistance. Lancet Infect. Dis. 19 , e26–e30 (2019).
D’Souza, M. P. & Frahm, N. Adenovirus 5 serotype vector-specific immunity and HIV-1 infection: a tale of T cells and antibodies. AIDS 24 , 803–809 (2010).
Lin, J. T. et al. Safety and immunogenicity from a phase I trial of inactivated severe acute respiratory syndrome coronavirus vaccine. Antivir. Ther. 12 , 1107–1113 (2007).
Martin, J. E. et al. A SARS DNA vaccine induces neutralizing antibody and cellular immune responses in healthy adults in a Phase I clinical trial. Vaccine 26 , 6338–6343 (2008).
Jacob, S. T. et al. Ebola virus disease. Nat. Rev. Dis. Primers 6 , 13 (2020).
Wolf, J. et al. Applying lessons from the Ebola vaccine experience for SARS-CoV-2 and other epidemic pathogens. NPJ Vaccines 5 , 51 (2020).
Ball, P. The lightning-fast quest for COVID vaccines—and what it means for other diseases. Nature 589 , 16–18 (2021).
Feldmann, H., Feldmann, F. & Marzi, A. Ebola: lessons on vaccine development. Annu. Rev. Microbiol. 72 , 423–446 (2018).
Gouglas, D., Christodoulou, M., Plotkin, S. A. & Hatchett, R. CEPI: driving progress toward epidemic preparedness and response. Epidemiol. Rev. 41 , 28–33 (2019).
Rottingen, J. A. et al. New vaccines against epidemic infectious diseases. N. Engl. J. Med. 376 , 610–613 (2017).
Sandbrink, J. B. & Shattock, R. J. RNA vaccines: a suitable platform for tackling emerging pandemics? Front. Immunol. 11 , 608460 (2020).
Jackson, N. A. C., Kester, K. E., Casimiro, D., Gurunathan, S. & DeRosa, F. The promise of mRNA vaccines: a biotech and industrial perspective. NPJ Vaccines 5 , 11 (2020).
US Department of Health and Human Health Services. Explaining Operation Warp Speed. https://health.mo.gov/living/healthcondiseases/communicable/novel-coronavirus-lpha/pdf/fact-sheet-operation-warp-speed.pdf 2020).
van Riel, D. & de Wit, E. Next-generation vaccine platforms for COVID-19. Nat. Mater. 19 , 810–812 (2020).
Article PubMed CAS Google Scholar
Polack, F. P. et al. Safety and efficacy of the BNT162b2 mRNA Covid-19 vaccine. N. Engl. J. Med. 383 , 2603–2615 (2020).
Baden, L. R. et al. Efficacy and safety of the mRNA-1273 SARS-CoV-2 vaccine. N. Engl. J. Med. 384 , 403–416 (2021).
US Food and Drug Administration. FDA takes key action in fight against COVID-19 by issuing emergency use authorization for first COVID-19 vaccine. https://www.fda.gov/news-events/press-announcements/fda-takes-key-action-fight-against-covid-19-issuing-emergency-use-authorization-first-covid-19 (2020).
US Food and Drug Administration. FDA takes additional action in fight against COVID-19 by issuing emergency use authorization for second COVID-19 vaccine. https://www.fda.gov/news-events/press-announcements/fda-takes-additional-action-fight-against-covid-19-issuing-emergency-use-authorization-second-covid (2020).
European Medicines Agency. EMA recommends first COVID-19 vaccine for authorisation in the EU. https://www.ema.europa.eu/en/news/ema-recommends-first-covid-19-vaccine-authorisation-eu (2020).
European Medicines Agency. EMA recommends COVID-19 Vaccine Moderna for authorisation in the EU. https://www.ema.europa.eu/en/news/ema-recommends-covid-19-vaccine-moderna-authorisation-eu (2021).
Liu, M. A. A Comparison of plasmid DNA and mRNA as vaccine technologies. Vaccines 7 , 37 (2019).
Article CAS PubMed Central Google Scholar
Pollard, A. J. et al. Safety and immunogenicity of a two-dose heterologous Ad26.ZEBOV and MVA-BN-Filo Ebola vaccine regimen in adults in Europe (EBOVAC2): a randomised, observer-blind, participant-blind, placebo-controlled, phase 2 trial. Lancet Infect. Dis . https://doi.org/10.1016/S1473-3099(20)30476-X (2020).
Adalja, A. A., Watson, M., Cicero, A. & Inglesby, T. Vaccine platform technologies: a potent tool for emerging infectious disease vaccine development. Health Secur. 18 , 59–60 (2020).
Excler, J. L. & Kim, J. H. Novel prime-boost vaccine strategies against HIV-1. Expert Rev. Vaccines 18 , 765–779 (2019).
European Pharmaceutical Review. AstraZeneca to test combination of AZD1222 and Sputnik V vaccines. https://www.europeanpharmaceuticalreview.com/news/136683/astrazeneca-to-test-combination-of-azd1222-and-sputnik-v-vaccines/ (2020).
World Health Organization. Draft landscape and tracker of COVID-19 candidate vaccines. https://www.who.int/publications/m/item/draft-landscape-of-covid-19-candidate-vaccines (2021).
Fritzell, B. Bridging studies. Dev. Biol. Stand. 95 , 181–188 (1998).
CAS PubMed Google Scholar
WHO Ad Hoc Expert Group on the Next Steps for Covid-19 Vaccine Evaluation. Placebo-controlled trials of Covid-19 vaccines—why we still need them. N. Engl. J. Med . 384 , e2 (2021).
Clemens, J., Brenner, R., Rao, M., Tafari, N. & Lowe, C. Evaluating new vaccines for developing countries. Efficacy or effectiveness? JAMA 275 , 390–397 (1996).
Kim, J. H., Marks, F. & Clemens, J. D. Looking beyond COVID-19 vaccine phase 3 trials. Nat. Med. 27 , 205–211 (2021).
Follmann, D. et al. Assessing durability of vaccine effect following blinded crossover in COVID-19 vaccine efficacy trials. Preprint at https://www.medrxiv.org/content/10.1101/2020.12.14.20248137v1 (2020).
World Health Organization. Global Advisory Committee on Vaccine Safety, 27–28 May 2020. https://www.who.int/vaccine_safety/committee/reports/May_2020/en/ (2020).
World Health Organization. COVID-19 vaccines: safety surveillance manual. https://apps.who.int/iris/handle/10665/338400 (2020).
European Medicines Agency. Pharmacovigilance plan of the EU Regulatory Network for COVID-19 Vaccines. https://www.ema.europa.eu/en/documents/other/pharmacovigilance-plan-eu-regulatory-network-covid-19-vaccines_en.pdf (2020).
Lee, G. M., Romero, J. R. & Bell, B. P. Postapproval vaccine safety surveillance for COVID-19 vaccines in the US. JAMA 324 , 1937–1938 (2020).
Kieny, M. P. & Rago, L. Regulatory policy for research and development of vaccines for public health emergencies. Expert Rev. Vaccines 15 , 1075–1077 (2016).
Lambert, P. H. et al. Consensus summary report for CEPI/BC March 12–13, 2020 meeting: assessment of risk of disease enhancement with COVID-19 vaccines. Vaccine 38 , 4783–4791 (2020).
Zellweger, R. M., Wartel, T. A., Marks, F., Song, M. & Kim, J. H. Vaccination against SARS-CoV-2 and disease enhancement - knowns and unknowns. Expert Rev. Vaccines 19 , 691–698 (2020).
Creanga, A. A. et al. Severity of 2009 pandemic influenza A (H1N1) virus infection in pregnant women. Obstet. Gynecol. 115 , 717–726 (2010).
Menendez, C., Lucas, A., Munguambe, K. & Langer, A. Ebola crisis: the unequal impact on women and children’s health. Lancet Glob. Health 3 , e130 (2015).
The PREVENT Working Group. Pregnant women and vaccines against emerging epidemic threats: ethics guidance on preparedness, research and response. http://vax.pregnancyethics.org/prevent-guidance (2018).
Krubiner, C. B. et al. Pregnant women and vaccines against emerging epidemic threats: ethics guidance for preparedness, research, and response. Vaccine 39 , 85–120 (2021).
Edridge, A. W. D. et al. Seasonal coronavirus protective immunity is short-lasting. Nat. Med. 26 , 1691–1693 (2020).
Plante, J. A. et al. Spike mutation D614G alters SARS-CoV-2 fitness. Nature https://doi.org/10.1038/s41586-020-2895-3 (2020).
Korber, B. et al. Tracking changes in SARS-CoV-2 spike: evidence that D614G increases infectivity of the COVID-19 virus. Cell 182 , 812–827 (2020).
Hou, Y. J. et al. SARS-CoV-2 D614G variant exhibits efficient replication ex vivo and transmission in vivo. Science 370 , 1464–1468 (2020).
Volz, E. et al. Evaluating the effects of SARS-CoV-2 spike mutation D614G on transmissibility and pathogenicity. Cell 184 , 64–75.e11 (2021).
Faria, N. R. Genomic characterisation of an emergent SARS-CoV-2 lineage in Manaus: preliminary findings. Virological https://virological.org/t/586 (2020).
Wibmer, C. K. et al. SARS-CoV-2 501Y.V2 escapes neutralization by South African COVID-19 donor plasma. Preprint at bioRxiv https://doi.org/10.1101/2021.01.18.427166 (2021).
BBC. Covid: South Africa halts AstraZeneca vaccine rollout over new variant. https://www.bbc.com/news/world-africa-55975052 (2021).
Mahase, E. Covid-19: Novavax vaccine efficacy is 86% against UK variant and 60% against South African variant. BMJ 372 , n296 (2021).
Johnson & Johnson. Johnson & Johnson announces single-shot Janssen COVID-19 vaccine candidate met primary endpoints in interim analysis of its phase 3 ENSEMBLE trial. https://www.jnj.com/johnson-johnson-announces-single-shot-janssen-covid-19-vaccine-candidate-met-primary-endpoints-in-interim-analysis-of-its-phase-3-ensemble-trial (2021).
World Health Organization. Global Influenza Surveillance and Response System (GISRS). https://www.who.int/influenza/gisrs_laboratory/en/
US Food and Drug Administration. Emergency use authorization. https://www.fda.gov/emergency-preparedness-and-response/mcm-legal-regulatory-and-policy-framework/emergency-use-authorization (2021).
European Medicines Agency. Conditional marketing authorization. https://www.ema.europa.eu/en/human-regulatory/marketing-authorisation/conditional-marketing-authorisation (2020).
Smith, M. J., Ujewe, S., Katz, R. & Upshur, R. E. G. Emergency use authorisation for COVID-19 vaccines: lessons from Ebola. Lancet 396 , 1707–1709 (2020).
World Health Organization. Emergency use listing procedure. https://www.who.int/publications/m/item/emergency-use-listing-procedure (2020).
Pharmaceutical Technology. Russia approves Sputnik V Covid-19 vaccine for senior citizens. https://www.pharmaceutical-technology.com/news/russia-sputnik-senior-citizens/ (2021).
Logunov, D. Y. et al. Safety and efficacy of an rAd26 and rAd5 vector-based heterologous prime-boost COVID-19 vaccine: an interim analysis of a randomised controlled phase 3 trial in Russia. Lancet https://doi.org/10.1016/S0140-6736(21)00234-8 (2021).
Xinhuanet. China approves first self-developed COVID-19 vaccine. http://www.xinhuanet.com/english/2020-12/31/c_139632402.htm (2020).
Sinopharm. China grants conditional market approval for Sinopharm CNBG’s COVID-19 vaccine. http://www.sinopharm.com/en/s/1395-4173-38862.html (2021).
CNBC. Dubai is offering the Pfizer vaccine to residents for free in addition to China’s Sinopharm shot. https://www.cnbc.com/2020/12/23/dubai-offering-pfizer-sinopharm-covid-vaccines-to-residents-for-free.html (2020).
Reuters. China approves Sinovac Biotech COVID-19 vaccine for general public use. https://www.reuters.com/article/us-health-coronavirus-vaccine-sinovac-idUSKBN2A60AY (2021).
Reuters. Pakistan approves Chinese CanSinoBIO COVID vaccine for emergency use. https://www.reuters.com/article/us-health-coronavirus-pakistan-vaccine/pakistan-approves-chinese-cansinobio-covid-vaccine-for-emergency-use-idUSKBN2AC1FG (2021).
Euronews. Coronavirus: 15m people in the UK have now had their first COVID jab—what about wider Europe? https://www.euronews.com/2021/02/14/coronavirus-15m-people-in-the-uk-have-now-had-their-first-covid-jab-what-about-wider-europ (2021).
Deng, C. & Malsin, J. China’s Covid-19 vaccine makers struggle to meet demand. The Wall Street Journal https://www.wsj.com/articles/chinas-covid-19-vaccine-makers-struggle-to-meet-demand-11612958560 (10 February 2021).
Pagliusi, S. et al. Developing countries vaccine manufacturers network: doing good by making high-quality vaccines affordable for all. Vaccine 31 , B176–B183 (2013).
Jadhav, S., Gautam, M. & Gairola, S. Role of vaccine manufacturers in developing countries towards global healthcare by providing quality vaccines at affordable prices. Clin. Microbiol. Infect. 20 , 37–44 (2014).
Kim, J. H. SARS-CoV-2 vaccine development, access, and equity. J. Exp. Med. 217 , e20201288 (2020).
Nhamo, G., Chikodzi, D., Kunene, H. P. & Mashula, N. COVID-19 vaccines and treatments nationalism: challenges for low-income countries and the attainment of the SDGs. Glob. Public Health 16 , 319–339 (2020).
World Bank. Global economy to expand by 4% in 2021; vaccine deployment and investment key to sustaining the recovery. https://www.worldbank.org/en/news/press-release/2021/01/05/global-economy-to-expand-by-4-percent-in-2021-vaccine-deployment-and-investment-key-to-sustaining-the-recovery (2021).
UN News. WHO chief warns against ‘catastrophic moral failure’ in COVID-19 vaccine access. https://news.un.org/en/story/2021/01/1082362 (18 January 2021).
Chinazzi, M. et al. The effect of travel restrictions on the spread of the 2019 novel coronavirus (COVID-19) outbreak. Science 368 , 395–400 (2020).
Download references
Author information
Authors and affiliations.
International Vaccine Institute, Seoul, Republic of Korea
Jean-Louis Excler & Jerome H. Kim
Coalition for Epidemic Preparedness Innovations (CEPI), London, UK
Melanie Saville
Gavi, the Vaccine Alliance, Geneva, Switzerland
Seth Berkley
You can also search for this author in PubMed Google Scholar
Contributions
J.-L.E., M.S., S.B. and J.H.K. equally contributed to the synopsis of the manuscript. J.-L.E. and J.H.K. wrote the text and tables of the manuscript. J.H.K. provided the figure. M.S. and S.B. edited the manuscript.
Corresponding authors
Correspondence to Jean-Louis Excler or Jerome H. Kim .
Ethics declarations
Competing interests.
J.-L.E. is a consultant for vaccine safety for the Brighton Collaboration, Johnson & Johnson and the US Military HIV Research Program. J.H.K. is a consultant to SK Bioscience. M.S. has a financial interest in Sanofi (shares). S.B. does not have any financial or nonfinancial conflicts of interest.
Additional information
Publisher’s note Springer Nature remains neutral with regard to jurisdictional claims in published maps and institutional affiliations.
Peer review information Nature Medicine thanks Robert Carnahan and David Morens for their contribution to the peer review of this work. Joao Monteiro was the primary editor on this article and managed its editorial process and peer review in collaboration with the rest of the editorial team.
Rights and permissions
Reprints and permissions
About this article
Cite this article.
Excler, JL., Saville, M., Berkley, S. et al. Vaccine development for emerging infectious diseases. Nat Med 27 , 591–600 (2021). https://doi.org/10.1038/s41591-021-01301-0
Download citation
Received : 27 January 2021
Accepted : 01 March 2021
Published : 12 April 2021
Issue Date : April 2021
DOI : https://doi.org/10.1038/s41591-021-01301-0
Share this article
Anyone you share the following link with will be able to read this content:
Sorry, a shareable link is not currently available for this article.
Provided by the Springer Nature SharedIt content-sharing initiative
This article is cited by
Parental hesitancy toward children vaccination: a multi-country psychometric and predictive study.
- Hamid Sharif-Nia
- Fatemeh Khoshnavay Fomani
BMC Public Health (2024)
Current landscape of mRNA technologies and delivery systems for new modality therapeutics
- Ruei-Min Lu
- Hsiang-En Hsu
- Han-Chung Wu
Journal of Biomedical Science (2024)
Empowering standardization of cancer vaccines through ontology: enhanced modeling and data analysis
- Xingxian Li
Journal of Biomedical Semantics (2024)
Assessment of immunopathological responses of a novel non-chemical biocide in C57BL/6 for safe disinfection usage
- Keun Bon Ku
- Jihwan Chae
- Heung Kyu Lee
Laboratory Animal Research (2024)
Nanotechnology’s frontier in combatting infectious and inflammatory diseases: prevention and treatment
- Yujing Huang
- Xiaohan Guo
Signal Transduction and Targeted Therapy (2024)
Quick links
- Explore articles by subject
- Guide to authors
- Editorial policies
Sign up for the Nature Briefing: Microbiology newsletter — what matters in microbiology research, free to your inbox weekly.

An official website of the United States government
Official websites use .gov A .gov website belongs to an official government organization in the United States.
Secure .gov websites use HTTPS A lock ( Lock Locked padlock icon ) or https:// means you've safely connected to the .gov website. Share sensitive information only on official, secure websites.
- Publications
- Account settings
- Advanced Search
- Journal List
Current topics in research ethics in vaccine studies
Prasad s kulkarni.
- Author information
- Copyright and License information
Address for correspondence: Dr. Prasad Kulkarni, Medical Director, Serum Institute of India Ltd, 212/2, Hadapsar, Pune, India. E-mail: [email protected]
This is an open-access article distributed under the terms of the Creative Commons Attribution-Noncommercial-Share Alike 3.0 Unported, which permits unrestricted use, distribution, and reproduction in any medium, provided the original work is properly cited.
About 7.6 million children under the age of five die every year, according to 2010 figures,[ 1 ] out of these 2.4 million children die from vaccine preventable diseases.[ 2 ] The problem is compounded by the absence of effective therapies for many infectious diseases. Obviously, new, more cost-effective and improved vaccines are needed today and in the future.
Vaccines have some distinct features than drugs. Unlike therapeutic molecules, vaccines have preventive role against specific infectious diseases. The target population is healthy people, mostly children and infants; as a result, tolerability of adverse events is less. Additionally, vaccines are highly complex substances derived from living microorganisms and their quality and safety needs to be demonstrated on a lot-to-lot basis. Naturally, these factors have some bearing on the clinical trials of vaccines. Here we discuss some of the current ethical issues in vaccine clinical trials.
Pediatric trials
Most of the vaccine studies are conducted in children, some of them in infants and even in newborns because that is where you want to catch them for prevention of an infection. However, children by themselves are unable to consent, and the vaccinator has to accept a legal guardian's agreement. Also, one would expect children to experience more adverse reactions than adults. For these and many other reasons, it is generally agreed that vaccine studies are, at least primarily, unethical in children if the relevant investigation can be done among adults. The main problem here is, however, that many infections are characteristically only pediatric diseases, or at least, those infections are specially harmful to the youngest.
One therefore needs to seek for a difficult balance between the true and ostensible need of a vaccine in the pediatric population. The CIOMS rightly states that “Before undertaking research involving children, the investigator must ensure that-the research might not be equally well be carried out in adults; and the purpose of the research is to obtain knowledge relevant to the health needs of children.”[ 3 ]
Parental consent
More in developing countries than elsewhere, parents or guardians of children may have little or no understanding of research trials. They may be unfamiliar with concepts such as “informed consent” and “confidentiality” and may not understand the scientific terms and processes involved in trials, including the use of randomization and placebos. Yet these parents will be called upon to give consent on behalf of their small children, or to explain to their older heirs (children) what is happening in the trial.
Another concern is consent of an appropriate legal representative in the absence of parental consent. Recently a demonstration project on a vaccine was conducted in India. An investigation was prompted after press reports of some deaths. Though the deaths were not found caused by the vaccine, consent obtained from hostel wardens in some subjects living in hostels was questioned.[ 4 ]
Need for the trial
Before launching a trial in children one must show that there is compelling need to use children to establish safety, immunogenicity, effectiveness or efficacy of the vaccine. Such a trial would not be justified if the child comes from population in which that particular disease is not a problem. Malaria vaccine cannot be tested soon in Europe or North America.
An absolute care must be taken to ensure that socioeconomic inequalities between industrialized and developing countries are not exploited i.e., that children in a poor country are not asked to undertake risks to produce a vaccine that, for economic or other reasons, would primarily benefit their counterparts in industrialized countries. At the same time, research should not be impeded that aims to reduce the inequality of health care and to benefit pediatric populations in need in developing countries.
Selection of control
If a good vaccine is already in use in some other country or community which is more or less comparable to site where the trial is planned, that vaccine should be used as the comparator. If such a vaccine does not exist, a placebo “vaccine” may be used, provided the set-up is thoroughly explained to the participants, their families and the community. Placebo controls are ethically acceptable when there is no proven vaccine for the indication for which the candidate vaccine is to be tested.[ 5 , 6 ]
A modification of this setting is that the placebo recipients receive the true vaccine later–but all this has to be explained in understandable words to the participants.
An alternative to the use of placebo is to give another vaccine that provides comparable benefit against another disease, or more willingly, against similar disease caused by different agents. This was the approach in Finland in the 1970s, when the first vaccines against bacterial meningitis (due to Neisseria meningitidis and H. influenza) were tested in children.[ 7 ] Here it was important these two types of meningitis were equally common in that community. For some vaccines, the choice is not difficult since there are no effective interventions so far, e.g., malaria or HIV vaccines.
In Indonesia, an exceptional approach was taken on 1998-2002.[ 8 ] Half of children received traditional DTP (diphtheria-tetanus-pertussis) vaccine, whereas the other half of children got DTP with H. influenza type B (Hib) component. Thus, all children were not in an equivalent position, but the setup was considered justified because in the absence of disease burden data and vaccine efficacy data in the region, the trial was deemed helpful for the decision whether or not to introduce Hib vaccination in Indonesia and the whole region.
When, instead of clinical efficacy, “only” immunogenicity (antibody production) is measured, the rules of equipoise are looser. The comparator vaccine may function more as “compensation” to the child in the trial's control arm. For instance, meningococcal C conjugate vaccine in a pneumococcal vaccine trial, or rabies vaccine in a Japanese encephalitis vaccine trial does not restore equipoise but benefit the child who would not otherwise receive that vaccine.
Age de-escalation
Age de-escalation means that phase I and II trials are conducted first in adults, then in older children, and finally, if relevant, in small children. Epidemiology of the disease, the risks/benefits of the vaccine for each age group, and the safety profile are all factors to be taken into account in de-escalation.
However, if a new vaccine is only for infants, trials in older children may expose them to unnecessary risks without giving any benefit to these too “old” vaccinees. Rotavirus vaccines are good examples in this category. Sometimes adult participants can be used in the first trials, although they are of no help in the efficacy trials.
Sometimes there are grounds to use child participants already in phase I trials. This is the case if the new vaccine would likely cause problems in adults (but not in children) because of prior immunity in adults e.g., DTP vaccine.
Participation of adolescents
Only a few vaccines as targeted just for adolescents: examples are human papillomavirus (HPV) and herpes virus (HSV) vaccines. However, adolescents may be used in the de-escalation studies before progressing to small children. The participation of adolescents often involves complex legal, ethical issues and operational issues.
Informed consent is problematic, because adolescents often have the intellectual and emotional capacity to provide consent, but do not have the legal right to consent. Also their views may not be the same as their parents’ views, and appropriate confidentiality can be difficult to maintain. An extreme would be a situation in which the youth disagrees but the parents agree the trial, or in which a willing adolescent would be included in the absence of parental consent.
The participation of adolescent girls is further complicated by the potential or soon materializing pregnancy. Not only would it perhaps risk the young mother and the fetus, but also raise complex issues regarding the consent, confidentiality and legal liability. Routine pregnancy testing of adolescent girls prior to the inclusion in a trial would also have its cultural problems.
Limitations of informed consent
Obtaining informed consent in a developing country has its own problems and should be seen as a process which begins from the voluntary decision to participate in the study. The decision should be based on sufficient information prior to the trial entry. The informed consent form should be simple enough to be understood by the often not-too-educated individual, or in case of a child, by parents or legal guardian, but still comprehensive to explain the concepts, potential risks and benefits, implications of the use of a placebo or other comparator, care that will be provided, and the indemnity for injury or death arising from the trial. Importantly, it must be stated clearly that a withdrawal from study is allowed at any time without giving an explanation for the decision. If the circumstances of the trial change significantly, the consent form is to be changed accordingly, and the whole study warrant discussion with the already enrolled participants. Another consent is then to be obtained.
The problems in getting valid consent are heightened in developing countries where people may be unfamiliar with scientific research, concepts and vocabulary. Thus, the expectations may be unrealistic. Also the individual's full autonomy might become endangered because of the society's cultural and/or gender norms, or the family or spousal pressure. All of these challenges are further complicated when the trial deals with children.
Child's assent
In the case of a child, every effort should be made to explain to him/her also, in language that is understandable to the child, what the participation means, as regards to potential risks (discomfort, time spent, etc.) and benefits, The investigators should document the child's assent.
Community consent
Since an informed consent may be culturally sensitive, family or community discussions are sometimes necessary, albeit the community consent should not be considered as a substitute for the individual consent. There may also be tension between the ethical responsibility to maintain individual confidentiality, and cultural norms that press for “shared confidentiality”. Within appropriate boundaries of confidentiality, it may be useful to have an impartial witness/observer present during an oral consent particularly if verbal rather than signed consent is sought. Such witnessed consent must be recorded in the trial files.
Potential for inducement
The improved medical care provided during the trial may constitute an inducement and may impact on the willingness to participate. Indeed, trial participants often accept the trial in the belief that they will receive improved treatment. It is important to explain that participation will not necessarily ensure protection against disease. In case of a study using placebo, the entire set-up and the meaning of randomization should be explained, including the fact that the participant might fall in the placebo group. Any care or other benefits that perhaps are offered should be described.
Another concern is if the parents see an opportunity for economic benefits, they may encourage enrolling their and perhaps other children in trials in which those should not necessarily be included. All efforts should be made to avoid any exploitation, and to minimize all mental, emotional and physical harm.
Standard of care
In case of vaccine trials in developing countries, the situation is tricky because of a high burden of disease and low standards of health care in that community. With the contribution of local authorities, a standard of care should be offered. This means an improvement in the health conditions of participants, and that it is sustainable. These efforts need an approval from the local ethics committees.
Duration of follow-up
An active follow-up should extend at least to the end of the trial. In case of an adverse effect, the, follow-up should be continued for an additional six months. In high mortality populations, it may be desirable to analyse long-term mortality changes and to follow-up participants for a number of years. Passive follow-up is advisable even longer, and if existing mechanisms can be used for this purpose.
Long-term follow-up may complicate a trial substantially and greatly increase the costs. Therefore, gathering only passive data may suffice. Creative follow-up should be contemplated, both for safety and long-term protection. The high titer measles vaccine was studied in some African countries, however on a long term follow up, it was discovered that female mortality was higher following the vaccine,[ 9 ] which resulted in abandoning the use of the vaccine. This important finding was detected only because of long-term follow-up.
Screening of subjects
Vaccine trials need to be conducted in healthy people and hence, the screening for inclusion/exclusion criteria is very critical. Enrolment of children with underlying medical conditions can complicate the safety outcomes. A recent vaccine trial in India brought forth this issue. A death was reported in the study after an infant had received a licensed vaccine used as a control. The investigation revealed that the infant who died had a pre-existing medical condition.[ 10 ] It is recognized that physical screening of young infants has limitations; however, every effort should be made to ascertain the health status. In case of suspicious cases, it is better to err on the safer side.
CONCLUSIONS
Vaccine clinical research needs to deal with certain ethical issues because of the inherent nature of these trials. The issues are more complicated since the research mostly happens in pediatric populations in developing countries. Keeping in mind these issues while designing research on vaccines is critical.
Source of Support: Nil
Conflict of Interest: None declared
- 1. Children: Reducing mortality. Fact sheet N°178, June 2012. [Last accessed on 2012 Sep 4]. Available from: http://www.who.int/mediacentre/factsheets/fs178/en/index.html .
- 2. [Last accessed on 2012 Sep 4]. Available from: http://www.cdc.gov/globalhealth/wiw/
- 3. Annex 2 International ethical guidelines for biomedical research involving human subjects. [Last accessed on 2012 Sep 4]. Available from: http://www.crc.gov.my/guidelines/pdf/International%20ethical%20guidelines%20for.pdf . [ PubMed ]
- 4. Srinivasan S. HPV vaccine trials and sleeping watchdogs. Indian J Med Ethics. 2011;8:73–4. doi: 10.20529/IJME.2011.031. [ DOI ] [ PubMed ] [ Google Scholar ]
- 5. UNAIDS. Guidance Document: Ethical Considerations in HIV Preventive Vaccine Research. May 2000. [Last accessed on 2012 Sep 4]. Available from: http://data.unaids.org/Publications/IRC-pub01/JC072-EthicalCons_en.pdf)
- 6. World Medical Association. Declaration of Helsinki: Ethical Principles for Medical Research Involving Human Subjects. 2004. [Last accessed on 2012 Sep 4]. Available from: http://www.wma.net/e/policy/b3.htm . [ PubMed ]
- 7. Peltola H, Käyhty H, Sivonen A, Mäkelä PH. Haemophilus influenzae type b capsular polysaccharide vaccine in children: A double-blind field study of 100,000 vaccinees 3 months to 5 years of age in Finland. Pediatrics. 1977;60:730–7. [ PubMed ] [ Google Scholar ]
- 8. Gessner BD, Sutanto A, Linehan M, Djelantik IG, Fletcher T, Gerudug IK, et al. Incidences of vaccine-preventable Haemophilus influenzae type b pneumonia and meningitis in Indonesian children: hamlet-randomised vaccine-probe trial. Lancet. 2005;365:43–52. doi: 10.1016/s0140-6736(04)17664-2. [ DOI ] [ PubMed ] [ Google Scholar ]
- 9. Aaby P, Samb B, Simondon F, Knudsen K, Seck AM, Bennett J, et al. Sex-specific differences in mortality after high-titre measles immunization in rural Senegal. Bull World Health Organ. 1994;72:761–70. [ PMC free article ] [ PubMed ] [ Google Scholar ]
- 10. Mudur G. Inquiry into vaccine trial in India suggests ineffective oversight. BMJ. 2009;338:b1418. doi: 10.1136/bmj.b1418. [ DOI ] [ PubMed ] [ Google Scholar ]
- View on publisher site
- PDF (493.4 KB)
- Collections
Similar articles
Cited by other articles, links to ncbi databases.
- Download .nbib .nbib
- Format: AMA APA MLA NLM
Add to Collections

Vaccines News
Top headlines, latest headlines.
- Maternal Antibodies: Malaria Vaccine Responses
- RSV Vaccine Effective for Older Adults
- Boost Performance of mRNA Vaccines
- Hacking Bacteria to Attack Cancer
- Mpox Vaccine: Safe, Robust Antibody Response
- Challenges in Vaccinations of Adults
- Getting the Flu and Then Your Shot May Help
- COVID Vaccination: Lower Cardiovascular Risk
- Antibody Inhibits Food Poisoning Bacteria
- Vaccine Against an Ancient Scourge
Earlier Headlines
Thursday, september 26, 2024.
- People With Unmedicated Mental Illness Are Less Likely to Be Vaccinated Against COVID-19, Swedish Study Finds
Friday, September 20, 2024
- A Two-Dose Schedule Could Make HIV Vaccines More Effective
Thursday, September 12, 2024
- Researchers Develop Promising Lassa Fever Vaccine
Monday, September 9, 2024
- Hep C: We're Closer Than Ever to Solving Mystery of Deadly Virus
Friday, September 6, 2024
- One Antibody to Neutralize Them All?
- Global Experts Help Nanomedicines Deliver on Healthcare Promise
- New RSV Vaccine for Older Adults Can Result in Individual and Societal Cost Savings, Benefits
Wednesday, September 4, 2024
- New Study Provides Insight to Why COVID Vaccines Hit Some Harder Than Others
- Investigational Mpox mRNA Vaccine Reduces Disease Severity in Primates Compared to Available Vaccines
- H5 Influenza Vaccines: What Needs to Be Done to Reduce the Risk of a Pandemic
Tuesday, September 3, 2024
- COVID-19 Vaccination Mandates Boosted Uptake Among Health Care Workers, Study Finds
Tuesday, August 27, 2024
- Game-Changing Needle-Free COVID-19 Intranasal Vaccine
Monday, August 26, 2024
- Gut Bacteria Composition Influences Rotavirus Vaccine Efficacy
Thursday, August 22, 2024
- Universal Flu Vaccine Candidate Protects Against Infection in Mice
Tuesday, August 20, 2024
- T Helper Cells May Be the Key to Improving Annual Influenza Vaccines
Thursday, August 15, 2024
- Nasal Spray Flu Vaccine Candidate Shows Promise When Administered Alongside High Dose Annual Shot
Wednesday, August 14, 2024
- Candidate Malaria Vaccine Provides Lasting Protection in NIH-Sponsored Trials
- Good News for People With MS: COVID-19 Vaccine Not Tied to Relapse, Study Finds
- New Vaccine Against Cervical Cancer Combines Prophylactic and Therapeutic Activity
- Shingles Increases Risk of Subsequent Cognitive Decline, Study Suggests
Sunday, August 11, 2024
- The Threat of Mpox Has Returned, but Public Knowledge About It Has Declined
Friday, August 9, 2024
- Prioritizing the Elderly for COVID-19 Boosters Reduces Overall Deaths
- New Two-Step Flu Vaccine Strategy Shows Promise in Pig Model
Tuesday, August 6, 2024
- Drug Bypasses Suppressive Immune Cells to Unleash Immunotherapy
- Researchers Create New Treatment and Vaccine for Flu and Various Coronaviruses
Friday, August 2, 2024
- Breakthrough in Bid to Develop Vaccines and Drugs for Neglected Tropical Disease
Thursday, August 1, 2024
- Meta-Analysis Pinpoints What Vaccination Intervention Strategies Different Countries Should Adopt
Wednesday, July 31, 2024
- Nasal COVID-19 Vaccine Halts Transmission, Animal Study Finds
Thursday, July 25, 2024
- Scientists Identify Key Protein Behind Spread of Shingles Virus
Friday, July 19, 2024
- Study Shows Promise for a Universal Influenza Vaccine
Wednesday, July 10, 2024
- Nanoparticle Vaccines Enhance Cross-Protection Against Influenza Viruses
Monday, July 8, 2024
- Study Backs RSV Vaccine Safety During Pregnancy
- Scientists Discover How to Improve Vaccine Responses to Potentially Deadly Bacterium
Wednesday, July 3, 2024
- Mobile Phone Data Helps Track Pathogen Spread and Evolution of Superbugs
Tuesday, July 2, 2024
- Advancing Toward a Preventative HIV Vaccine
- Regular Vaccine Boosts May Help People Who Are Immunocompromised Fight COVID-19, Study Suggests
Thursday, June 27, 2024
- Vaccination May Reduce Memory Loss from COVID-19 Infections
- Bird Flu Stays Stable on Milking Equipment for at Least One Hour
Wednesday, June 19, 2024
- A New Tuberculosis Vaccine Candidate Recombinant Protein With Additional Post-Translational Modifications Occurring in Mycobacterium Tuberculosis Cells
Friday, June 14, 2024
- More Hospitals Than Ever Require Staff to Get Flu Shots, U.S. Study Finds

Tuesday, June 11, 2024
- Safer Virus Helps Eliminate Cancer
Monday, June 10, 2024
- Feeling Rough After Your COVID Shot? Congrats, It's Working!
Friday, June 7, 2024
- How $4 Billion Funded the Unprecedented COVID-19 Pandemic Response
Thursday, June 6, 2024
- Study Finds No Association Between COVID-19 Vaccines and Stillbirths
Friday, May 31, 2024
- Antibodies May Aid Effort to Fight Influenza B
Thursday, May 30, 2024
- Novel Vaccine Concept Generates Immune Responses That Could Produce Multiple Types of HIV Broadly Neutralizing Antibodies
- Researchers Take Step Toward Development of Universal COVID-19 Antibodies
Wednesday, May 29, 2024
- Local Disparities May Prevent National Vaccination Efforts for Rubella
- Bird Flu: Diverse Range of Vaccines Platforms 'crucial' For Enhancing Human Pandemic Preparedness
Tuesday, May 28, 2024
- How COVID-19 'breakthrough' Infections Alter Your Immune Cells
Thursday, May 23, 2024
- Century-Old Vaccine Protects Type 1 Diabetics from Infectious Diseases
Friday, May 17, 2024
- Repeat COVID-19 Vaccinations Elicit Antibodies That Neutralize Variants, Other Viruses
- A Trial HIV Vaccine Triggered Elusive and Essential Antibodies in Humans
Monday, May 13, 2024
- Birth by C-Section More Than Doubles Odds of Measles Vaccine Failure
Thursday, May 9, 2024
- GPS-Like System Shows Promise as HIV Vaccine Strategy to Elicit Critical Antibodies
Wednesday, May 8, 2024
- A Tailored Vaccine Could One Day Treat Eczema in Children
- An Adjuvant Made in Yeast Could Lower Vaccine Cost and Boost Availability
Tuesday, May 7, 2024
- Mobile Teams Bring COVID-19 Vaccines to Rural Villages in Sierra Leone
Monday, May 6, 2024
- New Vaccine Effective Against Coronaviruses That Haven't Even Emerged Yet
- Expanding a Lymph Node, Boosting a Vaccine
Wednesday, May 1, 2024
- New Strategy Could Lead to Universal, Long-Lasting Flu Shot
- New mRNA Cancer Vaccine Triggers Fierce Immune Response to Fight Malignant Brain Tumor
Monday, April 29, 2024
- Microarray Patches Safe and Effective for Vaccinating Children, Trial Suggests
- Study Finds School Entry Requirements Linked to Increased HPV Vaccination Rates
Wednesday, April 24, 2024
- A Vaccine to Fight Antibiotic Resistance
Thursday, April 18, 2024
- Metabolic Health Before Vaccination Determines Effectiveness of Anti-Flu Response
Monday, April 15, 2024
- Vaccine Breakthrough Means No More Chasing Strains
Wednesday, April 3, 2024
- Developing a Vaccine for the 'zombie Drug' Xylazine
- New Discovery Unravels Malaria Invasion Mechanism
Monday, April 1, 2024
- Researchers Develop More Broadly Protective Coronavirus Vaccine
Tuesday, March 26, 2024
- Just Ask: Patients in the ER Are Willing to Get a Flu Shot
Wednesday, March 20, 2024
- Powerful New AI Can Predict People's Attitudes to Vaccines
Monday, March 18, 2024
- Addressing Both Flu and COVID-19 Through a Single, Multitasking Injection
Friday, March 15, 2024
- Key Metabolic Process Responsible for Rapid Immune Responses Discovered
- DNA Origami-Based Vaccines Toward Safe and Highly-Effective Precision Cancer Immunotherapy
- Common Viruses Trigger Most Cases of Intussusception in Children
Thursday, March 14, 2024
- Vac to the Future
Tuesday, March 12, 2024
- Scientists Find Weak Points on Epstein-Barr Virus
- Vaccine Monitoring Crucial as SARS-CoV-2 Variants Continue to Evolve
Friday, March 8, 2024
- Zika Virus Vaccine Emerges as an Unlikely Hero in Battling Brain Cancer
- Optimizing Boosters: How COVID mRNA Vaccines Reshape Immune Memory After Each Dose
Thursday, March 7, 2024
- COVID Vaccines Are Safe for Pregnant Women and Babies, Study Finds
Monday, March 4, 2024
- Zika Vaccine Safe, Effective When Administered During Pregnancy
Friday, March 1, 2024
- New Antibodies Target 'dark Side' Of Influenza Virus Protein
- Latest Booster Reduces Adults' Risk of Moderate or Severe COVID by More Than Half
Thursday, February 29, 2024
- Changes in Flu Circulation Means U.S. Likely to See Vaccines Move from Quadrivalent to Trivalent
Wednesday, February 28, 2024
- In Fight Against Brain Pathogens, the Eyes Have It
Monday, February 26, 2024
- Long-Term Data Reveals SARS-CoV-2 Infection and Vaccine-Induced Antibody Responses Are Long-Lasting
- 'Hexaplex' Vaccine Aims to Boost Flu Protection
Thursday, February 22, 2024
- How Children's Birthdays Help Show the Best Month for Flu Shots
Wednesday, February 21, 2024
- TB Vaccine Shrinks Liver Cancer Tumors in Mice
Tuesday, February 20, 2024
- Study Identifies Increase in Antibiotic-Resistant Typhoid
- Breakthrough in Developing the PD-1-Enhanced DNA Vaccine for Over 6-Year cART-Free AIDS Prevention and Virologic Control
Monday, February 19, 2024
- Flu Vaccines Were Effective in 2022-2023 Flu Season, Studies Find
Wednesday, February 14, 2024
- COVID-19 Vaccination and Boosting During Pregnancy Protects Infants for Six Months, Study Finds
Tuesday, February 6, 2024
- Switching Arms Improves Effectiveness of Two-Dose Vaccinations, Study Suggests
- How T Cells Combat Tuberculosis
Friday, February 2, 2024
- Scientists See an Ultra-Fast Movement on Surface of HIV Virus
Tuesday, January 30, 2024
- DNA Particles That Mimic Viruses Hold Promise as Vaccines
Friday, January 26, 2024
- Common Cold or COVID-19? Some T Cells Are Ready to Combat Both
- LATEST NEWS
- Health & Medicine
- Diseases & Conditions
- Alzheimer's Research
- Amyotrophic Lateral Sclerosis
- Attention Deficit Disorder
- Back and Neck Pain
- Birth Defects
- Bladder Disorders
- Blood Clots
- COVID and SARS
- Cervical Cancer
- Bladder Cancer
- Multiple Myeloma
- Pancreatic Cancer
- Brain Tumor
- Colon Cancer
- Breast Cancer
- Ovarian Cancer
- Lung Cancer
- Mesothelioma
- Skin Cancer
- Prostate Cancer
- Cerebral Palsy
- Chikungunya
- Chronic Fatigue Syndrome
- Cold and Flu
- Crohn's Disease
- Cystic Fibrosis
- Dengue Fever
- Down Syndrome
- Eating Disorder Research
- Encephalitis
- Epilepsy Research
- Erectile Dysfunction
- Fibromyalgia
- Gastrointestinal Problems
- HIV and AIDS
- Headache Research
- Hearing Loss
- Heart Health
- Cholesterol
- Stroke Prevention
- Heart Disease
- Hormone Disorders
- Hypertension
- Infectious Diseases
- Insomnia Research
- Irritable Bowel Syndrome
- Kidney Disease
- Liver Disease
- Lung Disease
- Lyme Disease
- Mental Health Research
- Multiple Sclerosis Research
- Mumps, Measles, Rubella
- Muscular Dystrophy
- Osteoporosis
- Parkinson's Research
- Prostate Health
- Restless Leg Syndrome
- Sickle Cell Anemia
- Sleep Disorder Research
- Thyroid Disease
- Triglycerides
- Tuberculosis
- Medical Topics
- Accident and Trauma
- Alternative Medicine
- Birth Control
- Bone and Spine
- Chronic Illness
- Controlled Substances
- Dietary Supplements and Minerals
- Epigenetics
- Food Additives
- Foodborne Illness
- Foot Health
- Gene Therapy
- Health Policy
- Human Biology
- Immune System
- Joint Health
- Medical Imaging
- Nervous System
- Pain Control
- Personalized Medicine
- Pharmacology
- Psychology Research
- Wounds and Healing
- PHYSICAL/TECH
- ENVIRONMENT
- SOCIETY & EDUCATION
- Bio-Based Fibers May Be Worse Than Plastics
- Towards a Hydrogen-Powered Future
- Deaf Male Mosquitoes Don't Mate
- AI for Real-Time, Patient-Focused Insight
- Tonga Eruption: Seismic Wave 15 Minutes Earlier
- The Secrets of Baseball's Magic Mud
- Exploding Neutron-Stars: Dance of Electrons
- Synthetic Genes for Medicine and Biotech
- Fossil of Huge Terror Bird Discovered
- Fastest-Feeding Black Hole in the Early Universe
Trending Topics
Strange & offbeat.
An official website of the United States government
Official websites use .gov A .gov website belongs to an official government organization in the United States.
Secure .gov websites use HTTPS A lock ( Lock Locked padlock icon ) or https:// means you've safely connected to the .gov website. Share sensitive information only on official, secure websites.
- Publications
- Account settings
- Advanced Search
- Journal List

Addressing Parental Vaccine Hesitancy towards Childhood Vaccines in the United States: A Systematic Literature Review of Communication Interventions and Strategies
Olivia olson, corinne berry, nirbhay kumar.
- Author information
- Article notes
- Copyright and License information
Correspondence: [email protected] (O.O.); [email protected] (N.K.)
Received 2020 Sep 15; Accepted 2020 Oct 4; Collection date 2020 Dec.
Licensee MDPI, Basel, Switzerland. This article is an open access article distributed under the terms and conditions of the Creative Commons Attribution (CC BY) license ( http://creativecommons.org/licenses/by/4.0/ ).
Parental vaccine hesitancy is becoming an increasingly important public health concern in the United States. In March 2020, an assessment of the latest CDC National Immunization Survey data found that more than one-third of U.S. children between the ages of 19 and 35 months were not following the recommended early childhood immunization schedule. Furthermore, a 2019 national survey found that approximately 1 in 4 parents reported serious concerns towards vaccinating their children. Vaccine hesitancy is now associated with a decrease in vaccine coverage and an increase in vaccine-preventable disease outbreaks and epidemics in the United States. Many studies have focused on understanding and defining the new socio-medical term, vaccine hesitancy; few have attempted to summarize past and current health communication interventions and strategies that have been successful or unsuccessful in tackling this growing phenomenon. This systematic literature review will attempt to aid public health professionals with a catalogue of health communication interventions and strategies to ultimately address and prevent parental vaccine hesitancy in the long term. Out of 1239 search results, a total of 75 articles were included for analysis, ranging from systematic reviews, quantitative surveys, and experimental designs to ethnographic and qualitative studies. For the presentation of results, a taxonomy was used to organize communication interventions according to their intended purpose. The catalogue of interventions was further broken down into specific components and themes that were identified in the literature as essential to either the success or failure in preventing and addressing parental vaccine hesitancy towards childhood vaccines.
Keywords: vaccine hesitancy, childhood vaccines, health communication, messaging, vaccine literacy, interventions, strategies, campaigns
1. Introduction
1.1. overview of parental vaccine hesitancy towards childhood vaccines in the u.s..
Vaccination is widely considered one of the greatest achievements in public health [ 1 , 2 ]. Vaccination programs have contributed significantly to the decline in mortality and morbidity from various infectious diseases. Most notably, vaccination has been credited with the elimination of polio in the Americas and eradication of smallpox worldwide [ 3 ]. Despite being recognized as one of the most successful public health measures for controlling and preventing disease, there has been an upward trend in the perception that vaccination is unsafe and unnecessary by a growing number of individuals in the United States (U.S.) [ 4 ]. As a result, hesitancy towards vaccination is beginning to threaten historical achievements that have been made in the U.S. to eliminate and reduce the burden of many infectious diseases that have afflicted humanity for centuries.
One particular concern in the U.S. is parental vaccine hesitancy towards childhood vaccines. Parental vaccine hesitancy has serious consequences for children and surrounding communities. A 2019 national survey found that approximately 1 in 4 parents reported serious concerns towards vaccinating their children [ 5 ]. In 2018, the Centers for Disease Control and Prevention (CDC) reported that while overall childhood immunization rates remained high during the time period 2012 to 2017, there continued to be an increase in the number of children with no vaccines at age 24 months [ 6 ]. More recently, in March 2020, an assessment of the latest CDC National Immunization Survey data found that more than one-third of U.S. children between the ages of 19 and 35 months were not following the recommended early childhood immunization schedule [ 7 ]. Concurrent with the increase in parental vaccine hesitancy is an increase in parents choosing to opt out their children from vaccinations required for school entry by obtaining non-medical vaccine exemptions [ 8 ]. The consequences of the increase in parental vaccine hesitancy over the last several years can most notably be seen in the recent U.S. measles outbreaks. Measles is one of the most contagious infectious diseases. Measles was declared eliminated from the U.S. in 2000 due to the nation’s effective vaccination program and strong public health system for detecting and responding to infectious disease outbreaks. Today, outbreaks of measles are occurring in the U.S. at an alarming rate, as unvaccinated groups are exposed to occasional outbreaks of the measles virus from outside the country [ 9 ]. A notable epidemic was the December 2014 measles outbreak among 125 children in Disneyland [ 10 ]. Outbreaks of measles have continued in the U.S. over the last two decades. The first spike in measles cases was in 2008 with 64 confirmed reported measles cases, followed by 667 reported cases in 2014, 375 reported cases in 2018, and a record-breaking outbreak of 1,282 cases in 2019 [ 11 ]. Large measles outbreaks among children are now occurring alongside an increasing number of parents either delaying or refusing to vaccinate their children against measles [ 12 ].
While opposition to vaccination has existed as long as vaccination itself, the subject of vaccines is especially contentious today [ 13 ]. There are numerous messages circulating widely both in support of and against childhood vaccines in the U.S. [ 14 , 15 ]. Parents seeking information about childhood vaccines have ample opportunity for exposure to false and misleading information about vaccines through traditional media outlets and interactive Web 2.0 and social media outlets online [ 16 ]. The plethora of immunization misinformation online buries science-based information and greatly affects health care professionals’ communication abilities and public immunization programs [ 16 , 17 ]. Misinformation and controversies about childhood vaccines tend to focus on questioning the safety and efficacy of specific vaccines [ 18 , 19 ]. One of the more prominent controversies has been the claim that the measles, mumps and rubella (MMR) vaccine causes autism. This claim originated from the 1998 Lancet article published by Andrew Wakefield, which was later retracted. While there is no scientific evidence that the MMR vaccine causes autism, the 1998 article had already caused fear, confusion and distrust over the safety of the vaccine that continues to this day [ 20 ]. Data from the last decade show that vaccine hesitancy among parents is continuing to increase as some parents begin to follow alternative childhood vaccination schedules, some become selective about which vaccines to give their child, and others refuse vaccines all together [ 19 , 21 , 22 , 23 , 24 , 25 , 26 , 27 ].
The decrease in childhood vaccine coverage and increase in childhood vaccine-preventable disease outbreaks and epidemics in the U.S. and around the world has been associated with what is now known as “vaccine hesitancy” [ 28 ]. In 2019, vaccine hesitancy was named one of the top 10 public health threats by the World Health Organization [ 28 ]. According to the WHO’s Strategic Advisory Group of Experts on Immunization (SAGE), vaccine hesitancy refers to a delay in the acceptance or refusal of vaccines despite the availability of vaccination services. Vaccine hesitancy is complex and context specific, varying across time, place, and vaccines. It is influenced by factors such as complacency, convenience, and confidence [ 29 ]. Vaccine hesitancy has been an emerging term in the socio-medical literature and describes vaccine decision-making or a lack of “vaccine confidence” and results in either delay or complete refusal [ 30 , 31 ]. Reasons for vaccine hesitancy are complex, and there are far more parents who are hesitant towards vaccines than outright refusing all vaccines [ 32 ]. A large number of conceptual models have been developed for grouping vaccine hesitancy determinants, which have brought both clarity and confusion to understanding the determinants of this phenomenon [ 31 ].
With the growing rates of vaccine hesitancy in the U.S., public health authorities are looking for effective communication interventions and strategies to promote vaccination, combat anti-vaccination messaging, and address vaccine hesitancy. To date, little is known about key health communication interventions and strategies that could be successful in addressing and preventing childhood vaccine hesitancy in the long term. What is known is that thus far, the majority of interventions to combat vaccine hesitancy have been educational and focused on a “knowledge-deficit” approach, assuming that vaccine-hesitant individuals will change their mind if given proper information. [ 24 , 33 , 34 ]. However, research has shown that vaccine hesitancy is more complex, involving emotional, cognitive, cultural, spiritual, social, and political factors [ 24 , 29 , 30 , 35 ]. Research has also shown that it matters which message techniques are used, and which messengers deliver the vaccine information [ 19 , 36 ]. Furthermore, the spread of false and misleading information about vaccines has led many parents to question the efficacy and safety of vaccines, dramatically impacting public understanding and trust in vaccines [ 24 , 25 , 37 , 38 ].
1.2. Vaccines, Vaccination, and Immunization
Vaccines help the body’s immune system fight off infections caused by bacterial, parasitic, and viral pathogens capable of causing serious, debilitating, and often deadly diseases. Since the introduction of the first vaccine, vaccination has been able to control many major diseases: smallpox, measles, mumps and rubella, diphtheria, pertussis, tetanus, yellow fever, and poliomyelitis [ 39 , 40 , 41 , 42 , 43 ]. Vaccination leads to the development of long-lasting and specific immunity against the infectious pathogen without any serious adverse events. Vaccination can be divided into two categories, active and passive vaccination approaches. In passive vaccination, preformed antibodies are administered just before or around the time of exposure. On the other hand, in the active vaccination approach, vaccines stimulate the immune system to produce either specific humoral (antibodies) and cellular immune responses, or both. Vaccines can be further divided into different categories: live attenuated vaccines, killed vaccines, subunit vaccines, nucleic acid (DNA and mRNA) vaccines, and vectored vaccines [ 39 , 40 ]. It is important to emphasize that an infection by a pathogen also leads to activation of the body’s immune system to elicit specific immune responses capable of providing partial or complete immunity to fight off the ongoing infection or future infections [ 39 , 40 , 44 ]. The significance of specific immune responses also lies in the fact that active vaccination leads to the generation of immunological memory, which helps the body to respond quickly and in a robust manner to prevent disease and death. Thus, a vaccine activates the body’s immune system upon administration (the process of vaccination), resulting in the generation of effective immunity (the process of getting immunized) to an infection or the disease caused by a pathogen [ 39 , 40 , 44 ].
1.3. Components of Vaccines
Individual components included in a vaccine formulation either participate during the activation of appropriate innate and adaptive immune responses to provide immunity or serve to stabilize the vaccine and maintain safety for the long lasting effectiveness of the vaccines ( Table 1 ) [ 45 , 46 ]. The components that help the immune system respond and build immunity to a specific disease include antigens and adjuvants. Antigens can be small amounts of weakened, attenuated or killed pathogen that can cause disease or a defined macromolecular component (protein, carbohydrate, or nucleic acid). Adjuvants are immunomodulatory components that activate the innate immune system and help the immune system respond strongly to a vaccine to mount an appropriate and desired immune response [ 45 ]. The other components that keep vaccines safe and stable include preservatives and stabilizers. Preservatives protect the vaccine from external contaminations, and stabilizers such as sugar or gelatin enhance the stability of vaccines during different phases of production, storage, and shipping.
Key components of approved vaccines in the U.S. reproduced from the CDC [ 46 ].
* Aluminum salts have been the only adjuvant used in most FDA-approved and licensed vaccines in the U.S.A.; other adjuvants such as ASO4, ASO1, etc. have also received FDA approval during the past decade, but not for vaccines used in children currently.
The components of newly licensed and existing vaccines are continuously reviewed and undergo an exhaustive safety and toxicity evaluation [ 46 ]. It is only upon such regulatory approvals by the FDA that new or modified existing vaccines move forward to licensure and recommendation for use in the U.S.A. by the CDC. Thus, monitoring vaccine safety and effectiveness remains an ongoing process for any vaccine intended for public use. The CDC routinely publishes the written recommendations for vaccinating U.S. children and sets the immunization schedules based on recommendations from the Advisory Committee on Immunization Practices (ACIP) and approval by the American Academy of Pediatrics (AAP), the American Academy of Family Physicians (AAFP), and the American College of Obstetricians and Gynecologists [ 47 ]. Table 2 outlines the current CDC-recommended vaccination schedule for children. Additional information about specific types of vaccines and vaccine-preventable diseases can be found on the CDC website [ 43 , 46 , 48 ].
Recommended vaccinations for infants and children (birth through 6 years) reproduced from the CDC [ 48 ].
* Two doses given at least four weeks apart are recommended for children age 6 months through 8 years of age who are getting an influenza (flu) vaccine for the first time and for some other children in this age group.
The goal of this systematic literature review is to aid public health authorities in developing effective strategies to address parental vaccine hesitancy towards childhood vaccines in the U.S. The definition, scope, and determinants of vaccine hesitancy differ greatly for different age groups, children, adolescents, young adults, and older adults. They also differ depending on the vaccine such as the MMR vaccine, the human papilloma virus (HPV) vaccine, the polio vaccine, the pertussis vaccine, and the seasonal flu vaccine, to name a few. The scope and determinants of vaccine hesitancy differ greatly for high-income countries (HICs) and lower- and middle-income countries (LMICs). This review will therefore focus specifically on parental vaccine hesitancy towards childhood vaccines in the U.S. The majority of discussion will focus on one main objective: exploring key health communication interventions and strategies that could be successful in addressing and preventing parental vaccine hesitancy towards childhood vaccines in the long term. There will also be discussion on the definition of vaccine hesitancy and the key determinants of vaccine hesitancy for childhood immunizations.
2. Materials and Methods
A systematic literature review was conducted following a structured search and screening process inspired by the Preferred Reporting Items for Systematic Reviews and Meta-Analysis (PRISMA) guidelines ( Figure 1 ). The search was performed in PubMed, PsychINFO, ProQuest, Cochrane Library, and Communication and Mass Media Complete databases, targeting publications from January 2008 to October 2019. Additional articles were identified through Google Scholar and other sources. The search strings included a variety of combinations of terms related to “vaccine hesitancy”, “communication”, and “intervention”. The full list of search terms is included in Table 3 . The results were filtered to include articles that were full-text, available in English, and predominately from the U.S. International publications from a few high-income, English-dominant countries including the U.K., Australia, and Canada were also included. The databases were initially searched for articles related to the main objective: exploring key communication interventions and strategies that can be successful in addressing and preventing parental vaccine hesitancy towards childhood vaccines in the long term. The inclusion criteria required the focus of the study to be on parental vaccine hesitancy around childhood vaccines. Articles with a primary focus outside of parental vaccine hesitancy and childhood vaccines were excluded. Articles that focused on annual/seasonal vaccines (i.e., influenza), adolescent immunizations (i.e., HPV or meningitis), or adult and older-adult vaccines (i.e., shingles) were also excluded. Articles were also excluded if their focus was on strategies and interventions to solely reduce immunization pain or if the intervention broadly focused on improving immunization rates and not addressing vaccine hesitancy. This systematic literature review followed a four-step screening process. First, reference manager software was used to combine and review the search results and eliminate any duplicates. Studies with multiple publications were narrowed down to the most applicable or informative article. Second, the articles were screened by two reviewers for relevance by title and abstract. The third screening process ensured that articles were available in full text and met the inclusion and exclusion criteria. Lastly, articles were excluded if not relevant or adding new information to the main objective. Relevant data were collected in Excel, including the study design and methods, sample size, target audience, country of publication, study results, and study implications. A total of 75 articles were included, ranging from systematic reviews, quantitative surveys, and experimental designs to ethnographic and qualitative studies.
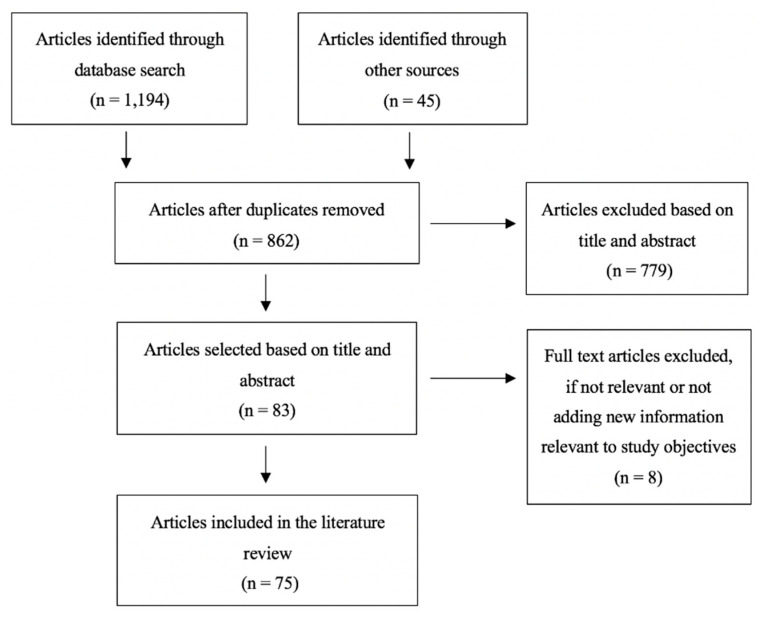
Preferred Reporting Items for Systematic Reviews and Meta-Analysis (PRISMA) flow diagram.
Table of search terms used.
3.1. Defining Vaccine Hesitancy
Several definitions have been proposed for the emerging term “vaccine hesitancy”. The WHO SAGE Working Group’s definition of vaccine hesitancy is the most commonly cited in the literature [ 4 , 23 , 24 ]. MacDonald, in 2015, highlighted the importance of one practical definition of vaccine hesitancy for immunization program managers, policy makers, clinicians, and researchers to effectively understand and address issues around vaccine hesitancy [ 4 , 31 ]. According to the WHO SAGE Working Group on Vaccine Hesitancy, it refers to a delay in the acceptance or refusal of vaccines despite the availability of vaccine services. Vaccine hesitancy is complex and context specific, varying across time, place, and vaccines. It includes factors such as complacency, convenience, and confidence [ 29 ]. The WHO SAGE Working Group, along with several other social scientists, further define vaccine hesitancy on the continuum of those who fully accept with few doubts, those who delay the vaccination schedule, and those who completely reject vaccines ( Figure 2 ) [ 29 , 31 , 49 ]. Several tools have been developed to define and measure vaccine hesitancy including the Vaccine Confidence Scale, the Global Vaccine Confidence Index, and the Vaccine Hesitancy Scale [ 29 , 50 , 51 , 52 , 53 ]. The most widely used tool specifically designed to measure parental vaccine hesitancy is the Parent Attitudes about Childhood Vaccines (PACV) survey, which was developed in 2011 [ 54 , 55 ]. The PACV survey is a short and self-administered validated survey that measures attitudes and beliefs about immunization prior to health care appointments to allow health care providers (HCP) to be informed about any hesitancy parents may have towards vaccines and to assist HCP in adapting their messages and communication strategies [ 56 , 57 , 58 , 59 ].
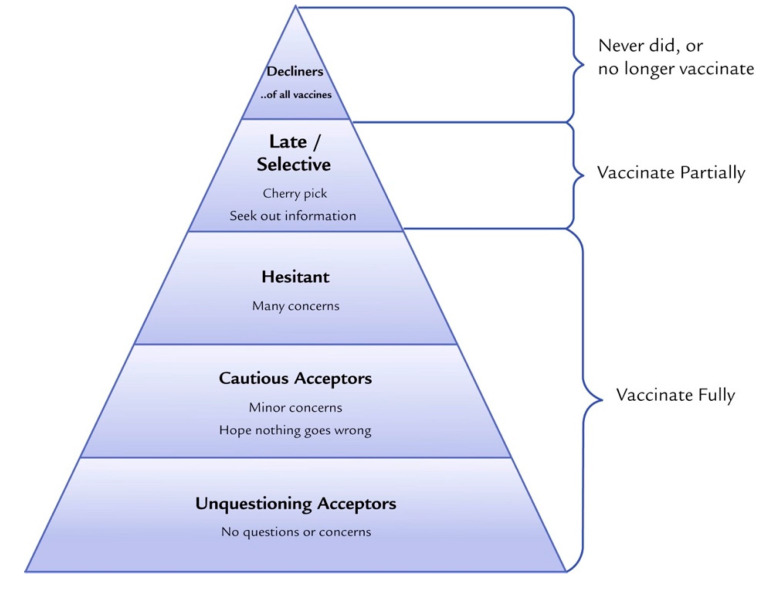
Vaccine acceptance spectrum from Leask et al. 2015. Improving Communication about Vaccination, adapted from Julie Leask’s online blog [ 60 ].
3.2. Key Determinants of Childhood Vaccine Hesitancy
The complexity of vaccine hesitancy and its determinants has led to a number of conceptual models for grouping vaccine hesitancy determinants [ 24 , 31 , 61 , 62 , 63 ]. The more well-known and widely referenced model “Vaccine Hesitancy Determinants Matrix” outlines factors influencing the decision-making behavior to accept, delay, or reject some or all vaccines under three categories: contextual, individual and group, and vaccine/vaccination-specific influences ( Table 4 ) [ 29 ].
The WHO SAGE Working Group Determinants of Vaccine Hesitancy Matrix [ 29 ].
3.3. Key Communication Interventions and Strategies That Address Vaccine Hesitancy
A select number of communication interventions found from the literature are categorized below as per Kaufman’s comprehensive taxonomy for organizing interventions for childhood vaccination in routine and campaign contexts [ 64 ]. The interventions were categorized as those that inform and educate, remind or recall, enhance community ownership, teach skills, provide support, facilitate decision making, and enable communication.
3.3.1. Inform and Educate
Gowda et al. [ 65 ] evaluated individually tailored versus untailored education materials for improving vaccination intention and positive vaccination attitudes for the MMR vaccine among parents who were surveyed and identified on a scale of vaccine hesitancy. The researchers tested web-based, individually tailored education material at four levels: image tailoring to parents’ race, content tailoring to specific vaccine-related concerns, experiential tailoring for parents’ past experiences, and name tailoring to the child’s name for messaging content. The tailored materials had a significantly greater impact on positive vaccination intentions and attitudes towards vaccination compared to untailored information provided through general educational web pages [ 65 ]. In another study, Jolley et al. [ 66 ] studied the effectiveness of attempting to counter and correct conspiracy arguments about childhood vaccines. It was found that anti-conspiracy arguments were not effective in increasing a person’s intention to vaccinate unless an individual was exposed to an anti-conspiracy theory message before providing the original conspiracy theory message.
3.3.2. Remind or Recall
Frew et al. [ 67 ] conducted a systematic literature review of 34 studies that used parental reminder and recall interventions based on the use of postcards, letters, the telephone, or a combination to improve early childhood immunization uptake. Researchers found that the majority of reminder and recall systems (79%) increased the likelihood of immunization uptake from study baselines by varying degrees, anywhere from 9% to 55%. In the same review, it was found that the use of tailored calendars that aim to encourage the immunization of children up to 24 months resulted in an increase in the immunization rate by 66% in the intervention group compared to 47% among the controls. In this study, calendars were tailored to the age of the baby, and included tailored pictures and messages from the family [ 67 ]. Frew et al. also discovered that several studies employed reminder and recall systems not only for vaccine-hesitant parents but for health care providers as well. It was found that utilizing a hospital’s electronic health record (EHR) to remind HCPs to discuss childhood vaccines with parents led to an improvement in early childhood immunization uptake, reflected by a reduction in missed opportunities by 36% over a 2 year period [ 67 ].
3.3.3. Enhance Community Ownership
Schoeppe et al. [ 68 ] introduced an education outreach and social marketing intervention called “The Immunity Community”, which aimed to reduce vaccine hesitancy. The goals of the “Immunity Community” intervention were to 1) address parental vaccine hesitancy by empowering parents to be immunization advocates in their community, 2) improve awareness of immunization as a social norm among parents at participating sites, and 3) change those parents’ attitudes and behaviors. Surveys revealed statistically significant improvements in vaccine-related attitudes: the percentage of parents who were concerned about other parents not vaccinating their children increased from 81.2% to 88.6%, and the percentage reporting themselves as “vaccine-hesitant” decreased from 22.6% to 14.0% [ 68 ]. This study demonstrates the promise of using parent advocates as part of a community-based approach to reduce vaccine hesitancy. Attwell et al. [ 69 ] evaluated the “I Immunize” campaign, which used a similar approach to “The Immunity Community” but focused more specifically on an identity and values-based approach to change vaccination attitudes. The campaign was carried out in a community that was known for their “alternative lifestyles” and lower-than-national vaccine coverage rates and utilized local community members to be advocates for the campaign. Community members were featured on posters, billboards, local newspapers, and social media posts, to deliver co-promoted behavioral messaging such as “My name is Andrew, I use cloth nappies, I eat at wholefoods, and I immunize”. The results from a community-wide survey of 304 respondents showed that 60% of the intended audience members were positively influenced by the campaign messages, 17% were negatively impacted, and 23% declared no impact. The researchers of the campaign attributed the success of the campaign to the involvement of local community members at every stage of the intervention in order to fit the needs of their audience [ 69 ].
3.3.4. Teach Skills
Henricksen et al. [ 58 ] studied the impact of physician communication training on parental vaccine hesitancy. In this two-arm cluster randomized trial carried out from March 2012 to December 2013 in four hospitals, mothers and providers were recruited from the postpartum units of the hospitals. The first component of the intervention involved a 45-min training that physicians went through, administered by a pediatrician immunization expert and health educator. The training was based on the Ask, Acknowledge, Advise communication framework. The second component was the delivery of paper materials with the branding “Let’s Talk Vaccines” detailing information on how to reinforce training messages and reach non-attenders. The third component was the delivery of 6-monthly email newsletters to physicians on a webinar version of the original training. Although this training was novel, it only had a marginal effect to no effect, and further improvements will be needed for the effectiveness of this approach [ 58 ].
3.3.5. Provide Support
Daley et al. [ 70 ] introduced an internet-based platform with vaccine information and interactive social media components to improve parents’ vaccine-related attitudes. In the intervention, study participants were randomized into the following groups: a study website with vaccine information and social media components, a website with vaccine information only, or the usual care. The parents who received the vaccine information with social media components were more likely to vaccinate their child as compared with parents who received the usual care only. Furthermore, the study highlighted the importance of social media for vaccination intention [ 70 ]. Another study conducted by Gagneur et al. [ 71 ] evaluated the impact of motivational interviewing techniques to support new mothers and increase vaccination coverage for new infants. A single session delivered one time in the maternity wards led to a significant increase of 15% in the vaccination intention of mothers compared to mothers who did not receive the intervention [ 71 ].
3.3.6. Facilitate Decision-Making
Williams et al. [ 59 ] conducted a randomized trial to increase acceptance of childhood vaccines by vaccine-hesitant parents. The researchers sought to explore the importance of facilitating decision-making for parents by delivering information on how to find accurate information on the Internet regarding childhood vaccines. The educational intervention for the randomized group included three components: (1) a short video developed by experts in vaccine safety and behavioral health, (2) an educational handout on common vaccine concerns, and (3) a handout with written instructions on how to find accurate medical information on the Internet. The researchers found that this brief educational intervention resulted in a short-term (~ 2-month long) benefit in improving parental attitudes towards vaccines [ 59 ].
3.3.7. Enable Communication
Davis et al. [ 72 ] introduced the Immunization Education Package (IEP) intervention to improve physician/parent communication around vaccine risks/benefits during well-baby visits in which immunizations were scheduled. The IEP intervention design consisted of a vaccine contraindication screening test, a large colorful poster entitled “7 questions parents need to ask about baby shots” that was placed in examination rooms, an information sheet with answers to the seven poster questions, and an office-based in-service conducted by a local opinion leader to provide advice. Overall, IEP showed significant improvements in both written and verbal vaccine communication, and the distribution of the federally mandated Vaccine Information Statements (VIS) increased from 33% to 91% at immunization visits [ 72 ]. Berry et al. [ 73 ] introduced the Sharing Knowledge about Immunization Project (SKAI) to improve communication between patient and provider and to implement better support systems for health care providers to address vaccine-hesitant parents [ 73 ]. This project explored the “triage and treat” approach to the clinical assessment and care of vaccine hesitancy, exploring the use of a referral pathway to a specialist immunization clinic. The SKAI approach was well received and showed an increase in parents’ intents to vaccinate their children [ 73 ]. Salmon et al. [ 74 ] introduced “MomsTalkShots”, a phone, tablet, and computer app that aims to increase the uptake of maternal infant vaccines and encourage communication between mothers and pediatricians. The mobile app initially screens patients based on survey questions and offers tailored vaccination information based on the parent’s vaccine attitudes, beliefs, intentions, demographics, and source credibility. A full evaluation of this intervention is still underway, but initial results have revealed that women are finding the app helpful (95%), trustworthy (94%), interesting (97%), and clear to understand (99%) [ 74 ].
3.4. Key Themes Identified in the Literature for Targeting Interventions
3.4.1. audiences targeted.
The primary target group for interventions and communication strategies to reduce parental vaccine hesitancy towards childhood vaccines has been parents with children aged 0–5 years who had serious questions/concerns about the safety of vaccines but were not adamantly against vaccinating their child, i.e., parents with strong beliefs and attitudes against vaccines [ 26 , 66 , 75 ]. Another group included health care providers, family members, teachers, and community members/neighborhood coalitions who were core influencers of vaccine-hesitant parents [ 59 , 67 , 76 ]. These efforts have highlighted the importance of including first-time mothers/parents for future studies [ 26 , 71 , 75 ].
3.4.2. Messenger of Vaccination Information
For interventions and communication strategies to be successful in preventing and addressing vaccine hesitancy, the “messenger” can be just as important as the “vaccine message” itself. Studies have revealed that parents are receptive to the message only if they find the messenger trustworthy and credible. It has also been suggested that the racial and/or ethnic background of the messenger (health care worker and physician) may also promote effective communication to improve vaccination rates in underrepresented minorities. It is also a common finding that health care professionals (HCP) are the preferred channels for vaccine information for parents ( Table 5 ).
Messengers of vaccination information.
3.4.3. Timing of Vaccination Information
Pregnancy represents an optimal point in time for delivering vaccination information to parents. This is the time when a parent’s intent to immunize their new infant can be most influenced and when negative attitudes about vaccination can be reshaped. This is also the time when parents are forming the foundation for all other future decisions regarding the overall health of the child [ 25 , 26 , 56 , 57 , 67 , 71 , 75 , 77 , 79 ]. Many studies suggest that education and interventions should be implemented very early in the pregnancy period since vaccine attitudes are likely formed before making vaccine decisions as parents [ 56 , 75 , 96 ]. Other studies have shown that intervening as late as postpartum can be effective [ 71 ]. Traditionally, vaccine information is provided to parents at well-child visits [ 57 ]. However, studies have shown that parents want vaccine information before and during the prenatal period and before each of the following well-child appointments [ 67 , 95 ]. The stepwise delivery of vaccination information, or the delivery of vaccination information at different points in time, has also been shown to increase the likelihood of childhood vaccination. This is because more frequent reminders can positively influence parents’ intent to immunize and reinforce positive beliefs and attitudes about childhood vaccination [ 67 , 79 , 83 , 97 ]. First-time mothers/parents have been cited as an important target population for the delivery of interventions to prevent the formation of vaccine-hesitancy attitudes and behaviors. This is an area for further research and evaluation for effectiveness [ 26 , 71 , 75 ].
3.4.4. Amount of Vaccination Information
The amount of vaccination information that is currently provided is inadequate and not enough to assist parents with a decision about whether to vaccinate their child or not. Parents want longer-than-usual appointments with their HCP to discuss vaccine information. Parents want to have enough time to receive clear answers to their questions and concerns, and to be engaged in an open dialogue-based discussion about childhood vaccines. Furthermore, parents want vaccine information that is tailored to their needs and tailored to their child [ 25 , 77 , 84 , 98 , 99 ]. It is important to also consider information overload that can confuse parents when designing interventions, especially interventions with multiple components to address vaccine hesitancy. If the intervention is being delivered by well-known trusted messengers of health information such as HCP, it is also important to consider the burden of time and additional training for HCP [ 100 ].
3.4.5. Content of Vaccination Information
The content of vaccination information must be tailored to the context and the target group ( Table 6 ) [ 38 , 63 , 67 , 79 , 85 ]. For example, the needs for vaccination information are very dependent on whether parents are slightly vaccine hesitant, moderately vaccine hesitant, or severely vaccine hesitant [ 31 , 37 , 85 ]. For parents that are lower on the vaccine-hesitancy spectrum (cautious acceptors or hesitant), the content of vaccination information that is requested is more general; parents want information about vaccine safety including balanced information about the benefits and risks of vaccination. These parents also want information about how to mitigate pain related to receiving vaccines/shots [ 23 , 25 , 26 , 77 ]. For parents who are higher on the vaccine-hesitancy spectrum (outright decliners or late/selective with vaccines), the vaccination information that is most often requested is more specific, such as the reasons behind combined versus single vaccines, alternatives to vaccines, the science behind how vaccines are made, technical information about vaccine production and delivery, and the reasons behind vaccine policies and recommendations. In addition, several studies have suggested that the content of vaccination information for vaccine-hesitant parents must also focus on providing information about the diseases that vaccines prevent since most of today’s parents do not have first-hand experience with the more deadly diseases that are now preventable [ 23 , 25 , 26 , 77 ].
Content of vaccination information.
3.4.6. Approaches to Vaccine Messaging
The effectiveness of vaccine communication strategies depends upon the message-framing techniques used [ 37 ]. Messaging in the form of storytelling with the use of gists, emotive anecdotes, and imagery has been shown to be among the most persuasive messaging strategies. Fear-based messaging, while effective in anti-vaccine messaging and the anti-vaccination movement, has been shown to be counterproductive in promoting vaccination ( Table 7 ).
Message-framing techniques for vaccination information.
3.4.7. Vaccine Misinformation and Disinformation
Successful health communication interventions and strategies must address the issues of vaccine misinformation and disinformation ( Table 8 ). There are two basic types of false information: misinformation or inadvertently drawing conclusions based on incorrect or incomplete facts, and disinformation, the deliberate spread of falsehoods to promote an agenda. Combating misinformation and disinformation in the fight against growing vaccine hesitancy has proved to be very difficult. It is argued that misinformation and disinformation can be corrected with factual information [ 17 ]. However, several studies have shown that attempting to correct misinformation and disinformation is difficult to do and can even be counter effective and reinforce strongly held false beliefs about childhood vaccines [ 18 , 107 , 111 ]
Common misconceptions about childhood vaccination.
3.4.8. Vaccine Literacy
Vaccine literacy is defined as not only knowledge about vaccines but the ability to use critical and evaluation skills to seek out the right information, especially with the ever-increasing information available in the media, particularly on the Internet [ 35 , 113 , 114 ]. Parents generally find it difficult to know which vaccine information sources to trust, and are confused about where to find information that they believe is unbiased and balanced. Vaccine literacy is an important factor in reducing the negative effects of exposure to misleading reports on vaccination [ 38 , 114 ].
3.4.9. Face-to-Face Communication Strategies
One-on-one dialogue-based communication strategies are seen as one of the most effective communication strategies for motivating parents to discuss childhood vaccines and to increase positive attitudes, behaviors, and intentions to vaccinate [ 25 , 27 , 34 , 64 , 73 , 95 , 97 ]. Parents often comment that they want to be engaged in discussion about childhood vaccinations with their HCP. They do not want to simply receive written material from their HCP. There have been a few interventions testing the effectiveness of posters in the physician’s office encouraging childhood vaccination communication between parents and HCP. These interventions have been shown to prompt more discussion between parents and HCP [ 27 , 79 , 115 ]. Social mobilization or engaging an entire community in the topic of childhood vaccination has also been shown to be an effective strategy. These are interventions that involve members of an institution, community networks, civic and religious groups, and others in a coordinated way to reach specific groups of people for dialogue and planned messaging [ 19 , 34 , 49 , 63 , 67 , 84 , 115 ]. For example, the “I Immunize” campaign trained parents as vaccine advocates to mobilize their communities to be more informed about the importance of childhood vaccines [ 69 ]. Lastly, recall and reminder systems for parents and HCP have been recommended for encouraging one-on-one discussion about childhood vaccinations [ 33 , 97 ].
3.4.10. Technology-Based Communication Strategies
Technology use in the U.S. is now widespread, with parents using the Internet and social media via computers and cellphones to search for health information [ 116 ]. As a result, information about vaccination in particular has changed in origin, nature, and speed. The Internet and Web 2.0 now produce, relay, and significantly accelerate the spread of vaccine information. While the Internet has allowed for free and anonymously accessible information, which can empower parents to own their health-related decisions, it has come with extreme costs. Information on the Internet is uncontrolled, and searches undertaken by web users are heavily dictated by algorithms, which contribute to hearsay and even magnify and amplify it [ 15 ]. Anti-vaccination messages, in particular, have largely been successful on the Internet and social media platforms, utilizing message-framing techniques such as storytelling and fear-based messaging to grab the audience’s attention and influence the number of shares [ 19 , 23 , 38 , 57 , 76 , 87 , 93 ]. A closer look at the successes of the anti-vaccination messages has, in turn, led to strategic pro-vaccine communication and social marketing interventions and campaigns on the Internet and Web 2.0 to improve positive vaccine beliefs, attitudes, intentions, and behaviors. In two randomized controlled trials (RCTs) that utilized a web-based social media intervention, parents were referred to vaccination information on a website or on a social media platform or were given standard paper-based vaccination information from their HCP. In both RCTs, it was found that referring parents to vaccine information on websites with social media components was more effective at increasing positive vaccination attitudes than distributing standard paper-based vaccination information [ 57 , 70 ]. Mobile applications on smartphones and tablets have also been shown to positively reinforce positive vaccination attitudes, behaviors, and intentions to vaccinate [ 74 , 100 , 105 ]. However, it is noted in several studies that for interventions interested in utilizing the Internet and social media to deliver vaccination information, it is critical to understand that this technology rapidly evolves, and therefore, these interventions need to be monitored and updated constantly [ 14 , 26 , 61 , 70 ].
4. Discussion
The main objective of this systematic literature review was to explore key health communication strategies and interventions that could be successful in addressing and preventing parental vaccine hesitancy towards childhood vaccines in the long term. This systematic literature review attempted to reduce reviewer bias through the use of objective reproducible criteria to select relevant publications and articles and assess their validity. One of the main strengths of the systematic literature review includes a narrow focus on the research question and objective. Other strengths include the comprehensive search for evidence, the criteria-based selection of relevant evidence, the analysis for validity, the objective summary of themes identified from the literature, and the evidence-based inferences. However, the strengths of a systematic literature review can also be weaknesses. The narrow focus of this systematic literature review did not allow for comprehensive coverage of vaccine hesitancy for all age groups, vaccines, and geographic contexts. Furthermore, only articles in English were assessed, not accounting for other publications and articles written in multiple languages. Finally, while the systematic literature review attempted to reduce review bias in the methodology, the evidence-based inferences from the study results could produce some bias.
4.1. Public Health Implications of the Findings
To date, there has not been a recent review on the trends and evidence base around health communication interventions and strategies to address parental vaccine hesitancy. In order to address this growing phenomenon, it is imperative that we understand which strategies are proving effective and which strategies are proving ineffective. Furthermore, there needs to be a stronger focus on preventing vaccine hesitancy.
4.2. Interventions That Have Been Successful or Unsuccessful
This systematic literature review provided an overview of interventions that were identified in the literature in the time period of January 2008 and October 2019. No single intervention or strategy was deemed to be effective on its own in addressing the complexity of parental vaccine hesitancy. Overall, the interventions that demonstrated the most success were multi-component, employed a variety of media or touchpoints, incorporated some dialogue element, and were personalized and tailored to the target populations’ specific vaccine concerns, historical experiences, religious or political affiliations, socioeconomic status, and trusted messengers for information. The specific components and communication strategies of interventions that proved essential for their success included the use of messaging-framing techniques such as storytelling with the use of gists, emotive anecdotes, and imagery. In addition, message-framing techniques that utilized science-based messaging also proved to be persuasive if presented in plain and simple language with the avoidance of medical and clinical jargon. Specific components and communication strategies that proved unsuccessful and sometimes detrimental included the use of message-framing techniques that used heavy statistical and numerical messaging, fear-based messaging, and messaging that attempted to correct or debunk commonly held myths and misconceptions about childhood vaccination. Several studies have proposed that future research should address the challenges of combating misinformation and disinformation. Furthermore, studies have pointed to the need to explore monitoring and evaluation systems for identifying vaccine-hesitant parents and strategically targeting interventions. Lastly, arguments have been made to mimic the effective strategies used in anti-vaccination movements, from the message-framing techniques to their tailoring of messaging and use of messengers for campaigns.
5. Recommendations and Conclusions
The following recommendations are intended to aid public health professionals in adapting, implementing, and scaling up health communication interventions and strategies to address and prevent parental vaccine hesitancy towards childhood vaccines in the long term. These recommendations are based on the catalogue of interventions and themes identified in the literature.
5.1. Start Early and Build Trust with Parents
Interventions should target parents early in pregnancy and target first-time mothers and parents, taking advantage of prenatal appointments and the first postnatal appointment. This is the time when parents are thinking about childhood immunizations the most and deciding whether to accept the recommended schedule, be selective about immunization, or outright refuse immunizations for their child.
5.2. Tailor the Information to the Target Audience, Their Reasons for Hesitancy, and the Specific Context
Parents want vaccine information that is tailored to their needs and tailored to their child. Future interventions should ensure that the needs of the target population are well studied. This includes understanding the target population’s specific vaccine concerns, historical experiences, religious or political affiliation, socioeconomic status, demographic background, and trusted messengers for information. This also includes targeting interventions depending on where parents reside on the vaccine-hesitancy continuum scale: those who accept all vaccines but are still concerned, those who refuse or delay some vaccines but accept others, and those who refuse all vaccines. Furthermore, vaccine messaging and stimuli should evoke different values. The message and messenger of vaccine information must be able to appeal to the values of the target audience in order to change vaccine behaviors.
5.3. Present Vaccination as the Default Approach
Health care providers need to be better equipped to present vaccination as the default approach and to address the issues of vaccine hesitancy through more training. Another solution could be the introduction of a “triage and treat” approach to clinical assessment and care for vaccine-hesitant parents. With regard to vaccine messaging, there needs to be a strong focus on presenting vaccination as the social norm. The challenge of today’s media environment is the amplification of dissenting views and perspectives. It inaccurately portrays that a larger proportion of individuals are hesitant or refusing vaccination.
5.4. Develop Vaccine Education Materials Using Health Literacy Best Practices
Parents that are on the vaccine-hesitancy scale often request science-based vaccine messaging in plain language that avoids medical jargon. Vaccine education materials should therefore go through an evaluation process before being released to the public. This does not mean that materials need to be “dumbed down”; rather, materials need to be evaluated to ensure the content, word choice and style, use of numbers, organization, layout and design, and use of visual aids is appropriate for the target audience.
5.5. Incorporate Dialogue-Based Communication Strategies and Provide Balanced Information about Vaccines
Interventions that incorporate dialogue elements are the most effective. Parents want an interactive and engaging approach to learning about childhood vaccines. Furthermore, parents want balanced information about both the benefits and risks including the side effects of vaccines. Parents report that too often, the standard childhood vaccination messaging focuses exclusively on the benefits and does not address the possible risks and side effects, which leads parents to have more questions and more concerns.
5.6. Tell Stories with Gists and Emotive Anecdotes
The effectiveness of vaccine communication strategies depends on the message-framing techniques used. Messaging in the form of storytelling with the use of gists, emotive anecdotes, and imagery has been shown to be among the most persuasive messaging strategies. The use of fear-based messaging should be avoided, as it has proven to reinforce vaccine hesitancy in parents.
5.7. Choose the Messenger of Vaccine Information Carefully
Health care providers are identified as the most trustworthy messengers of vaccine information. Future interventions involving HCP should carefully consider and mitigate the potential for any unintended consequences, i.e., disturbance of the trust between HCP and parents. A second important messenger of vaccination information is a parent’s social network, which can include family, friends, colleagues, neighbors, and other personal relations. It has been found that a parent’s social network can be more predictive of a parent’s decision to vaccinate their child than any other variable, including the parent’s own perceptions of vaccination.
5.8. Focus on Vaccine Messaging That Centers Both the Child and Community
It is unclear whether it is more advantageous to frame vaccine messaging as a social responsibility or an individual’s responsibility. Therefore, it is important to provide both kinds of messaging and tailor the message specifically to the target population’s background and values.
5.9. Use Technology to Promote Vaccination
Internet-based interventions to address parents’ vaccine concerns can be a constructive strategy since parents often seek vaccine information on the Internet. Utilizing web-based platforms with social media applications has shown a lot of promise in engaging this target group. Furthermore, new mothers and parents often use baby apps and other phone apps throughout their pregnancy and after childbirth for information on how to keep their child healthy. It could be advantageous to develop a phone app for new moms/new parents with information about childhood vaccines.
5.10. Use Caution When Addressing Vaccine Misinformation
Several emerging studies have shown a backfire effect when interventions attempt to correct or debunk vaccine misinformation, leading to the intervention actually reinforcing strongly held false beliefs about vaccines and increasing vaccine hesitancy. This is an area for further research. What is clear, however, is that there must be improvements in the monitoring and tracking of the spread of misleading and inaccurate vaccine information on the Internet. If misinformation cannot be corrected, it needs to be prevented.
5.11. Improve Parents’ Vaccine Literacy and Critical Thinking Skills
The expansion of the Internet has allowed for vaccine misinformation and fear-based anti-vaccine messages to have a greater reach and impact. There is a need for interventions to combat the spread of misinformation and disinformation. There is an enormous need for interventions to focus on improving vaccine literacy and critical thinking for parents. This could involve a series of community interventions that introduce parents to how to be an informed health care consumer and, more specifically, a more informed consumer of vaccine information.
Acknowledgments
We sincerely apologize to many other investigators whose research contributions could not be adequately cited due to space limitation. The authors thank members at CommunicateHealth for numerous discussions about vaccine hesitancy.
Author Contributions
Conceptualization, O.O., C.B., and N.K.; methodology, O.O. and C.B; investigation, O.O., C.B., and N.K.; resources, O.O. and C.B.; writing—original draft preparation, O.O.; writing—reviewing and editing, O.O., C.B., and N.K.; supervision, C.B. and N.K. All authors have read and agreed to the published version of the manuscript.
This project received no external funding.
Conflicts of Interest
The authors declare no conflict of interest.
- 1. Centers for Disease Control Ten Great Public Health Achievements—United States, 1900–1999. [(accessed on 7 February 2020)]; Available online: https://www.cdc.gov/mmwr/preview/mmwrhtml/00056796.htm .
- 2. Centers for Disease Control Ten Great Public Health Achievements—United States, 2001–2010. [(accessed on 7 February 2020)]; Available online: https://www.cdc.gov/mmwr/preview/mmwrhtml/mm6019a5.htm .
- 3. Greenwood B. The contribution of vaccination to global health: Past, present and future. Philos. Trans. R. Soc. B Biol. Sci. 2014;369:20130433. doi: 10.1098/rstb.2013.0433. [ DOI ] [ PMC free article ] [ PubMed ] [ Google Scholar ]
- 4. Dubé E., Laberge C., Guay M., Bramadat P., Roy R., Bettinger J.A. Vaccine hesitancy. Hum. Vaccines Immunother. 2013;9:1763–1773. doi: 10.4161/hv.24657. [ DOI ] [ PMC free article ] [ PubMed ] [ Google Scholar ]
- 5. Kempe A., Saville A.W., Albertin C., Zimet G., Breck A., Helmkamp L., Vangala S., Dickinson L.M., Rand C., Humiston S., et al. Parental Hesitancy About Routine Childhood and Influenza Vaccinations: A National Survey. Pediatrics. 2020;146:e20193852. doi: 10.1542/peds.2019-3852. [ DOI ] [ PMC free article ] [ PubMed ] [ Google Scholar ]
- 6. Centers for Disease Control and Prevention (CDC) [(accessed on 7 February 2020)];Vaccination Coverage Among Children Aged 19–35 Months—United States. 2017 Available online: https://www.cdc.gov/mmwr/volumes/67/wr/mm6740a4.htm .
- 7. Hargreaves A.L., Nowak G., Frew P., Hinman A.R., Orenstein W.A., Mendel J., Aikin A., Nadeau J.A., McNutt L.-A., Chamberlain A.T., et al. Adherence to Timely Vaccinations in the United States. Pediatrics. 2020;145:e20190783. doi: 10.1542/peds.2019-0783. [ DOI ] [ PubMed ] [ Google Scholar ]
- 8. Olive J.K., Hotez P.J., Damania A., Nolan M.S. The state of the antivaccine movement in the United States: A focused examination of nonmedical exemptions in states and counties. PLoS Med. 2018;15:e1002578. doi: 10.1371/journal.pmed.1002578. [ DOI ] [ PMC free article ] [ PubMed ] [ Google Scholar ]
- 9. Centers for Disease Control and Prevention U.S. Public Health Response to the Measles Outbreak. (2019, February 27) [(accessed on 7 February 2020)]; Available online: https://www.cdc.gov/washington/testimony/2019/t20190227.htm .
- 10. Centers for Disease Control and Prevention Measles Outbreak—California, December 2014–February 2015. (2015, February 20) [(accessed on 7 February 2020)]; Available online: https://www.cdc.gov/mmwr/preview/mmwrhtml/mm6406a5.htm .
- 11. Centers for Disease Control and Prevention [(accessed on 25 April 2020)];Measles Cases and Outbreaks. (2020, April 6) Available online: https://www.cdc.gov/measles/cases-outbreaks.html .
- 12. Patel M., Lee A., Redd S., Clemmons N., McNaiill R., Cohn A., Gastanaduy P. Increase in Measles Cases—United States, January 1–April 26. [(accessed on 7 February 2020)];2019 doi: 10.15585/mmwr.mm6817e1. Available online: https://www.cdc.gov/mmwr/volumes/68/wr/mm6817e1.htm . [ DOI ] [ PubMed ]
- 13. Hussain A., Ali S., Ahmed M., Hussain S. The Anti-vaccination Movement: A Regression in Modern Medicine. Cureus. 2018;10:2919. doi: 10.7759/cureus.2919. [ DOI ] [ PMC free article ] [ PubMed ] [ Google Scholar ]
- 14. Rosselli R., Martini M., Bragazzi N. The old and the new: Vaccine hesitancy in the era of the Web 2.0. Challenges and opportunities. J. Prev. Med. Hyg. 2016;57:E47–E50. [ PMC free article ] [ PubMed ] [ Google Scholar ]
- 15. Stahl J.-P., Cohen R., Denis F., Gaudelus J., Martinot A., Lery T., Lepetit H. The impact of the web and social networks on vaccination. New challenges and opportunities offered to fight against vaccine hesitancy. Med. Mal. Infect. 2016;46:117–122. doi: 10.1016/j.medmal.2016.02.002. [ DOI ] [ PubMed ] [ Google Scholar ]
- 16. Macdonald N.E., Dubé E. Promoting immunization resiliency in the digital information age. Can. Commun. Dis. Rep. 2020;46:20–24. doi: 10.14745/ccdr.v46i01a04. [ DOI ] [ PMC free article ] [ PubMed ] [ Google Scholar ]
- 17. Burki T. Vaccine misinformation and social media. Lancet Digit. Health. 2019;1:e258–e259. doi: 10.1016/S2589-7500(19)30136-0. [ DOI ] [ Google Scholar ]
- 18. Pluviano S., Watt C., Della Sala S. Misinformation lingers in memory: Failure of three pro-vaccination strategies. PLoS ONE. 2017;12:e0181640. doi: 10.1371/journal.pone.0181640. [ DOI ] [ PMC free article ] [ PubMed ] [ Google Scholar ]
- 19. Larson H.J., Cooper L.Z., Eskola J., Katz S.L., Ratzan S. Addressing the vaccine confidence gap. Lancet. 2011;378:526–535. doi: 10.1016/S0140-6736(11)60678-8. [ DOI ] [ PubMed ] [ Google Scholar ]
- 20. Godlee F., Smith J., Marcovitch H. Wakefield’s article linking MMR vaccine and autism was fraudulent. BMJ. 2011;342:c7452. doi: 10.1136/bmj.c7452. [ DOI ] [ PubMed ] [ Google Scholar ]
- 21. Hendrix K.S., Finnell S.M.E., Zimet G.D., Sturm L.A., Lane K.A., Downs S.M. Vaccine Message Framing and Parents’ Intent to Immunize Their Infants for MMR. Pediatrics. 2014;134:e675–e683. doi: 10.1542/peds.2013-4077. [ DOI ] [ PMC free article ] [ PubMed ] [ Google Scholar ]
- 22. Smith P.J., Humiston S.G., Marcuse E.K., Zhao Z., Dorell C.G., Howes C., Hibbs B. Parental Delay or Refusal of Vaccine Doses, Childhood Vaccination Coverage at 24 Months of Age, and the Health Belief Model. Public Health Rep. 2011;126:135–146. doi: 10.1177/00333549111260S215. [ DOI ] [ PMC free article ] [ PubMed ] [ Google Scholar ]
- 23. Edwards K.M., Hackell J.M. Countering Vaccine Hesitancy. Pediatrics. 2016;138:20162146. doi: 10.1542/peds.2016-2146. [ DOI ] [ PubMed ] [ Google Scholar ]
- 24. Dubé E., Vivion M., E MacDonald N. Vaccine hesitancy, vaccine refusal and the anti-vaccine movement: Influence, impact and implications. Expert Rev. Vaccines. 2014;14:99–117. doi: 10.1586/14760584.2015.964212. [ DOI ] [ PubMed ] [ Google Scholar ]
- 25. Glanz J., Kraus C.R., Daley M.F. Addressing Parental Vaccine Concerns: Engagement, Balance, and Timing. PLoS Biol. 2015;13:e1002227. doi: 10.1371/journal.pbio.1002227. [ DOI ] [ PMC free article ] [ PubMed ] [ Google Scholar ]
- 26. Salmon A.D., Dudley M.Z., Glanz J.M., Omer S.B. Vaccine hesitancy. Vaccine. 2015;33:D66–D71. doi: 10.1016/j.vaccine.2015.09.035. [ DOI ] [ PubMed ] [ Google Scholar ]
- 27. Williams E.S. What are the factors that contribute to parental vaccine-hesitancy and what can we do about it? Hum. Vaccines Immunother. 2014;10:2584–2596. doi: 10.4161/hv.28596. [ DOI ] [ PMC free article ] [ PubMed ] [ Google Scholar ]
- 28. World Health Organization [(accessed on 7 February 2020)];Ten threats to global health in 2019. 2019 Available online: https://www.who.int/news-room/feature-stories/ten-threats-to-global-health-in-2019 .
- 29. World Health Organization [(accessed on 7 February 2020)];Report of the SAGE Working Group on Vaccine Hesitancy. (2014, November 12) Available online: https://www.who.int/immunization/sage/meetings/2014/october/SAGE_working_group_revised_report_vaccine_hesitancy.pdf?ua=1 .
- 30. Kumar D., Chandra R., Mathur N., Samdariya S., Kapoor N. Vaccine hesitancy: Understanding better to address better. Isr. J. Health Policy Res. 2016;5:2. doi: 10.1186/s13584-016-0062-y. [ DOI ] [ PMC free article ] [ PubMed ] [ Google Scholar ]
- 31. Macdonald N.E. Vaccine hesitancy: Definition, scope and determinants. Vaccine. 2015;33:4161–4164. doi: 10.1016/j.vaccine.2015.04.036. [ DOI ] [ PubMed ] [ Google Scholar ]
- 32. Shen S., Dubey V. Addressing vaccine hesitancy: Clinical guidance for primary care physicians working with parents. Can. Fam. Physician Med. Fam. Can. 2019;65:175–181. [ PMC free article ] [ PubMed ] [ Google Scholar ]
- 33. Sadaf A., Richards J.L., Glanz J., A Salmon D., Omer S.B. A systematic review of interventions for reducing parental vaccine refusal and vaccine hesitancy. Vaccine. 2013;31:4293–4304. doi: 10.1016/j.vaccine.2013.07.013. [ DOI ] [ PubMed ] [ Google Scholar ]
- 34. Jarrett C., Wilson R., O’Leary M., Eckersberger E., Larson H.J. Strategies for addressing vaccine hesitancy—A systematic review. Vaccine. 2015;33:4180–4190. doi: 10.1016/j.vaccine.2015.04.040. [ DOI ] [ PubMed ] [ Google Scholar ]
- 35. Arede M., Bravo-Araya M., Bouchard É., Gill G.S., Plajer V., Shehraj A., Shuaib Y.A. Combating Vaccine Hesitancy: Teaching the next Generation to Navigate through the Post Truth Era. Front. Public Health. 2019;6:381. doi: 10.3389/fpubh.2018.00381. [ DOI ] [ PMC free article ] [ PubMed ] [ Google Scholar ]
- 36. Penţa M.A., Băban A. Message Framing in Vaccine Communication: A Systematic Review of Published Literature. Health Commun. 2017;33:1–16. doi: 10.1080/10410236.2016.1266574. [ DOI ] [ PubMed ] [ Google Scholar ]
- 37. Larson H., Clarke R.M., Jarrett C., Eckersberger E., Levine Z., Schulz W.S., Paterson P. Measuring trust in vaccination: A systematic review. Hum. Vaccines Immunother. 2018;14:1599–1609. doi: 10.1080/21645515.2018.1459252. [ DOI ] [ PMC free article ] [ PubMed ] [ Google Scholar ]
- 38. Betsch C., Brewer N.T., Brocard P., Davies P., Gaissmaier W., Haase N., Leask J., Renkewitz F., Renner B., Reyna V.F., et al. Opportunities and challenges of Web 2.0 for vaccination decisions. Vaccine. 2012;30:3727–3733. doi: 10.1016/j.vaccine.2012.02.025. [ DOI ] [ PubMed ] [ Google Scholar ]
- 39. Vetter V., Denizer G., Friedland L.R., Krishnan J., Shapiro M. Understanding modern-day vaccines: What you need to know. Ann. Med. 2017;50:110–120. doi: 10.1080/07853890.2017.1407035. [ DOI ] [ PubMed ] [ Google Scholar ]
- 40. Murphy K., Weaver C. Janeway’s Immunobiology. [(accessed on 28 September 2020)]; Available online: http://www.garlandscience.com/product/isbn/9780815345053 .
- 41. Office of Disease Prevention and Health Promotion Immunization and Infectious Diseases (2020, August 08) [(accessed on 23 August 2020)]; Available online: https://www.healthypeople.gov/2020/topics-objectives/topic/immunization-and-infectious-diseases .
- 42. World Health Organization [(accessed on 7 February 2020)];Vaccination greatly reduces disease, disability, death and inequity worldwide. (2008, February) doi: 10.2471/BLT.07.040089. Available online: https://www.who.int/bulletin/volumes/86/2/07-040089/en/ [ DOI ] [ PMC free article ] [ PubMed ]
- 43. Centers for Disease Control 14 Diseases You Almost Forgot About (Thanks to Vaccines). (2020, January 03) [(accessed on 7 February 2020)]; Available online: https://www.cdc.gov/vaccines/parents/diseases/forgot-14-diseases.html .
- 44. Wack A., Rappuoli R. Vaccinology at the beginning of the 21st century. Curr. Opin. Immunol. 2005;17:411–418. doi: 10.1016/j.coi.2005.05.005. [ DOI ] [ PMC free article ] [ PubMed ] [ Google Scholar ]
- 45. O’Hagan D.T., Rappuoli R. The safety of vaccines. Drug Discov. Today. 2004;9:846–854. doi: 10.1016/S1359-6446(04)03234-9. [ DOI ] [ PMC free article ] [ PubMed ] [ Google Scholar ]
- 46. Centers for Disease Control and Prevention [(accessed on 7 February 2020)];Vaccines: Vac-Gen/Additives in Vaccines Fact Sheet. (2019, August 5) Available online: https://www.cdc.gov/vaccines/vac-gen/additives.htm .
- 47. Centers for Disease Control and Prevention [(accessed on 7 February 2020)];Who Sets the Immunization Schedule? (2012, March 8) Available online: https://www.cdc.gov/vaccines/parents/schedules/sets-schedule.html .
- 48. Centers for Disease Control and Prevention [(accessed on 7 February 2020)];Easy-to-read Immunization Schedule by Vaccine for Ages Birth-6 Years. (2020, February 3) Available online: https://www.cdc.gov/vaccines/schedules/easy-to-read/child-easyread.html .
- 49. Leask J., Kinnersley P., Jackson C., Cheater F., Bedford H., Rowles G. Communicating with parents about vaccination: A framework for health professionals. BMC Pediatr. 2012;12:154. doi: 10.1186/1471-2431-12-154. [ DOI ] [ PMC free article ] [ PubMed ] [ Google Scholar ]
- 50. Oduwole O.E., Pienaar E.D., Mahomed H., Iwu C.J. Current tools available for investigating vaccine hesitancy: A scoping review protocol. BMJ Open. 2019;9:e033245. doi: 10.1136/bmjopen-2019-033245. [ DOI ] [ PMC free article ] [ PubMed ] [ Google Scholar ]
- 51. Larson H.J., Schulz W.S., Tucker J.D., Smith D.M.D. Measuring Vaccine Confidence: Introducing a Global Vaccine Confidence Index. PLoS Curr. 2015;7:e033245. doi: 10.1371/currents.outbreaks.ce0f6177bc97332602a8e3fe7d7f7cc4. [ DOI ] [ PMC free article ] [ PubMed ] [ Google Scholar ]
- 52. Gilkey M.B., Magnus B.E., Reiter P.L., McRee A.-L., Dempsey A.F., Brewer N.T. The Vaccination Confidence Scale: A brief measure of parents’ vaccination beliefs. Vaccine. 2014;32:6259–6265. doi: 10.1016/j.vaccine.2014.09.007. [ DOI ] [ PMC free article ] [ PubMed ] [ Google Scholar ]
- 53. Shapiro G.K., Tatar O., Dubé E., Amsel R., Knäuper B., Naz A., Perez S., Rosberger Z. The vaccine hesitancy scale: Psychometric properties and validation. Vaccine. 2018;36:660–667. doi: 10.1016/j.vaccine.2017.12.043. [ DOI ] [ PubMed ] [ Google Scholar ]
- 54. Opel D.J., Mangione-Smith R., Taylor J.A., Korfiatis C., Wiese C., Catz S., Martin D.P. Development of a survey to identify vaccine-hesitant parents. Hum. Vaccines. 2011;7:419–425. doi: 10.4161/hv.7.4.14120. [ DOI ] [ PMC free article ] [ PubMed ] [ Google Scholar ]
- 55. Opel D.J., Taylor J.A., Zhou C., Catz S., Myaing M., Mangione-Smith R. The Relationship between Parent Attitudes About Childhood Vaccines Survey Scores and Future Child Immunization Status. JAMA Pediatr. 2013;167:1065. doi: 10.1001/jamapediatrics.2013.2483. [ DOI ] [ PMC free article ] [ PubMed ] [ Google Scholar ]
- 56. Cunningham R.M., Minard C.G., Guffey D., Swaim L.S., Opel D.J., Boom J.A. Prevalence of Vaccine Hesitancy Among Expectant Mothers in Houston, Texas. Acad. Pediatr. 2018;18:154–160. doi: 10.1016/j.acap.2017.08.003. [ DOI ] [ PubMed ] [ Google Scholar ]
- 57. Glanz J.M., Wagner N.M., Narwaney K.J., Kraus C.R., Shoup J.A., Xu S., O’Leary S.T., Omer S.B., Gleason K.S., Daley M.F. Web-based Social Media Intervention to Increase Vaccine Acceptance: A Randomized Controlled Trial. Pediatrics. 2017;140 doi: 10.1542/peds.2017-1117. [ DOI ] [ PMC free article ] [ PubMed ] [ Google Scholar ]
- 58. Henrikson N.B., Opel D.J., Grothaus L., Nelson J., Scrol A., Dunn J., Faubion T., Roberts M., Marcuse E.K., Grossman D.C. Physician Communication Training and Parental Vaccine Hesitancy: A Randomized Trial. Pediatrics. 2015;136:70–79. doi: 10.1542/peds.2014-3199. [ DOI ] [ PubMed ] [ Google Scholar ]
- 59. Williams S.E., Rothman R.L., Offit P.A., Barnett E., Sullivan M., Edwards K.M. A Randomized Trial to Increase Acceptance of Childhood Vaccines by Vaccine-Hesitant Parents: A Pilot Study. Acad. Pediatr. 2013;13:475–480. doi: 10.1016/j.acap.2013.03.011. [ DOI ] [ PMC free article ] [ PubMed ] [ Google Scholar ]
- 60. Leask J. Improving communication about vaccination. (2015, May 12) [(accessed on 2 November 2019)]; Available online: https://julieleask.wordpress.com/2015/05/12/improving-communication-about-vaccination-sarah/
- 61. Leask J., Willaby H.W., Kaufman J. The big picture in addressing vaccine hesitancy. Hum. Vaccines Immunother. 2014;10:2600–2602. doi: 10.4161/hv.29725. [ DOI ] [ PMC free article ] [ PubMed ] [ Google Scholar ]
- 62. Thomson A., Robinson K., Vallée-Tourangeau G. The 5As: A practical taxonomy for the determinants of vaccine uptake. Vaccine. 2016;34:1018–1024. doi: 10.1016/j.vaccine.2015.11.065. [ DOI ] [ PubMed ] [ Google Scholar ]
- 63. Thomson A., Vallée-Tourangeau G., Suggs L.S. Strategies to increase vaccine acceptance and uptake: From behavioral insights to context-specific, culturally-appropriate, evidence-based communications and interventions. Vaccine. 2018;36:6457–6458. doi: 10.1016/j.vaccine.2018.08.031. [ DOI ] [ PubMed ] [ Google Scholar ]
- 64. Kaufman J., Ames H., Bosch-Capblanch X., Cartier Y., Cliff J., Glenton C., Lewin S., Muloliwa A.M., Oku A., Oyo-Ita A., et al. The comprehensive ‘Communicate to Vaccinate’ taxonomy of communication interventions for childhood vaccination in routine and campaign contexts. BMC Public Health. 2017;17:423. doi: 10.1186/s12889-017-4320-x. [ DOI ] [ PMC free article ] [ PubMed ] [ Google Scholar ]
- 65. Gowda C., Schaffer S.E., Kopec K., Markel A., Dempsey A.F. A pilot study on the effects of individually tailored education for MMR vaccine-hesitant parents on MMR vaccination intention. Hum. Vaccines Immunother. 2013;9:437–445. doi: 10.4161/hv.22821. [ DOI ] [ PMC free article ] [ PubMed ] [ Google Scholar ]
- 66. Jolley D., Douglas K.M. Prevention is better than cure: Addressing anti-vaccine conspiracy theories. J. Appl. Soc. Psychol. 2017;47:459–469. doi: 10.1111/jasp.12453. [ DOI ] [ Google Scholar ]
- 67. Frew P.M., Lutz C.S. Interventions to increase pediatric vaccine uptake: An overview of recent findings. Hum. Vaccines Immunother. 2017;13:2503–2511. doi: 10.1080/21645515.2017.1367069. [ DOI ] [ PMC free article ] [ PubMed ] [ Google Scholar ]
- 68. Schoeppe J., Melton M., Faubion T., Miller C., Matthys J., Hsu C., Cheadle A. The Immunity Community: A Community Engagement Strategy for Reducing Vaccine Hesitancy. Health Promot. Pract. 2017;18:654–661. doi: 10.1177/1524839917697303. [ DOI ] [ PubMed ] [ Google Scholar ]
- 69. Attwell K., Freeman M. I Immunise: An evaluation of a values-based campaign to change attitudes and beliefs. Vaccine. 2015;33:6235–6240. doi: 10.1016/j.vaccine.2015.09.092. [ DOI ] [ PubMed ] [ Google Scholar ]
- 70. Daley M.F., Narwaney K., Shoup J.A., Wagner N.M., Glanz J.M. Addressing Parents’ Vaccine Concerns: A Randomized Trial of a Social Media Intervention. Am. J. Prev. Med. 2018;55:44–54. doi: 10.1016/j.amepre.2018.04.010. [ DOI ] [ PMC free article ] [ PubMed ] [ Google Scholar ]
- 71. Gagneur A., Lemaître T., Gosselin V., Farrands A., Carrier N., Petit G., Valiquette L., De Wals P. A postpartum vaccination promotion intervention using motivational interviewing techniques improves short-term vaccine coverage: PromoVac study. BMC Public Health. 2018;18:811. doi: 10.1186/s12889-018-5724-y. [ DOI ] [ PMC free article ] [ PubMed ] [ Google Scholar ]
- 72. Davis T.C., Fredrickson D.D., Bocchini C., Arnold C.L., Green K.W., Humiston S.G., Wilder E., Bocchini J.A. Improving vaccine risk/benefit communication with an immunization education package: A pilot study. Ambul. Pediatr. 2002;2:193–200. doi: 10.1367/1539-4409(2002)002<0193:IVRBCW>2.0.CO;2. [ DOI ] [ PubMed ] [ Google Scholar ]
- 73. Berry N., Danchin M., Trevena L., Witteman H., Kinnersley P., Snelling T., Robinson P., Leask J. Sharing knowledge about immunization (SKAI): An exploration of parents’ communication needs to inform development of a clinical communication support intervention. Vaccine. 2018;36:6480–6490. doi: 10.1016/j.vaccine.2017.10.077. [ DOI ] [ PubMed ] [ Google Scholar ]
- 74. Salmon A.D., Limaye R.J., Dudley M.Z., Oloko O.K., Church-Balin C., Ellingson M.K., Spina C.I., Brewer S.E., Orenstein W.A., Halsey N.A., et al. MomsTalkShots: An individually tailored educational application for maternal and infant vaccines. Vaccine. 2019;37:6478–6485. doi: 10.1016/j.vaccine.2019.08.080. [ DOI ] [ PMC free article ] [ PubMed ] [ Google Scholar ]
- 75. Sowers C. Doctoral Dissertation. Catholic University of America; Washington, DC, USA: 2017. Influencing Parental Intent to Immunize through Prenatal Education. [ Google Scholar ]
- 76. Wang E., Baras Y., Buttenheim A. “Everybody just wants to do what’s best for their child”: Understanding how pro-vaccine parents can support a culture of vaccine hesitancy. Vaccine. 2015;33:6703–6709. doi: 10.1016/j.vaccine.2015.10.090. [ DOI ] [ PMC free article ] [ PubMed ] [ Google Scholar ]
- 77. Ames H.M., Glenton C., Lewin S. Parents’ and informal caregivers’ views and experiences of communication about routine childhood vaccination: A synthesis of qualitative evidence. Cochrane Database Syst. Rev. 2017;2:CD011787. doi: 10.1002/14651858.CD011787.pub2. [ DOI ] [ PMC free article ] [ PubMed ] [ Google Scholar ]
- 78. Bedford H. Pro-vaccine messages may be counterproductive among vaccine-hesitant parents. Evid. Based Med. 2014;19:219. doi: 10.1136/ebmed-2014-110037. [ DOI ] [ PubMed ] [ Google Scholar ]
- 79. Healy C.M., Pickering L.K. How to Communicate With Vaccine-Hesitant Parents. Pediatrics. 2011;127:S127–S133. doi: 10.1542/peds.2010-1722S. [ DOI ] [ PubMed ] [ Google Scholar ]
- 80. Nour R. A Systematic Review of Methods to Improve Attitudes Towards Childhood Vaccinations. Cureus. 2019;11:e5067. doi: 10.7759/cureus.5067. [ DOI ] [ PMC free article ] [ PubMed ] [ Google Scholar ]
- 81. Tafuri S., Gallone M., Cappelli M., Martinelli D., Prato R., Germinario C. Addressing the anti-vaccination movement and the role of HCWs. Vaccine. 2014;32:4860–4865. doi: 10.1016/j.vaccine.2013.11.006. [ DOI ] [ PubMed ] [ Google Scholar ]
- 82. Greenberg J., Dubé E., Driedger M. Vaccine Hesitancy: In Search of the Risk Communication Comfort Zone. PLoS Curr. 2017;9 doi: 10.1371/currents.outbreaks.0561a011117a1d1f9596e24949e8690b. [ DOI ] [ PMC free article ] [ PubMed ] [ Google Scholar ]
- 83. Lwembe S., Green S.A., Tanna N., Connor J., Valler C., Barnes R. A qualitative evaluation to explore the suitability, feasibility and acceptability of using a ’celebration card’ intervention in primary care to improve the uptake of childhood vaccinations. BMC Fam. Pract. 2016;17:101. doi: 10.1186/s12875-016-0497-9. [ DOI ] [ PMC free article ] [ PubMed ] [ Google Scholar ]
- 84. Kempe A., Daley M.F., McCauley M.M., Crane L.A., Suh C.A., Kennedy A.M., Basket M.M., Stokley S.K., Dong F., Babbel C.I., et al. Prevalence of Parental Concerns About Childhood Vaccines. Am. J. Prev. Med. 2011;40:548–555. doi: 10.1016/j.amepre.2010.12.025. [ DOI ] [ PubMed ] [ Google Scholar ]
- 85. Dubé E., Vivion M., Sauvageau C., Gagneur A., Gagnon R., Guay M. Nature Does Things Well, Why Should We Interfere? Qual. Health Res. 2015;26:411–425. doi: 10.1177/1049732315573207. [ DOI ] [ PubMed ] [ Google Scholar ]
- 86. Stevens J.C. Doctoral Dissertation. The University of Arizona; Tucson, AZ, USA: 2016. The C.A.S.E. Approach (Corroboration, about Me, Science, Explain/Advise): Improving Communication with Vaccine-Hesitant Parents. [ Google Scholar ]
- 87. Broniatowski D.A., Hilyard K.M., Dredze M. Effective vaccine communication during the disneyland measles outbreak. Vaccine. 2016;34:3225–3228. doi: 10.1016/j.vaccine.2016.04.044. [ DOI ] [ PMC free article ] [ PubMed ] [ Google Scholar ]
- 88. Meppelink C.S., Smit E.G., Fransen M.L., Diviani N. “I was Right about Vaccination”: Confirmation Bias and Health Literacy in Online Health Information Seeking. J. Health Commun. 2019;24:129–140. doi: 10.1080/10810730.2019.1583701. [ DOI ] [ PubMed ] [ Google Scholar ]
- 89. Moran M.B., Lucas M., Everhart K., Morgan A., Prickett E. What makes anti-vaccine websites persuasive? A content analysis of techniques used by anti-vaccine websites to engender anti-vaccine sentiment. J. Commun. Health. 2016;9:151–163. doi: 10.1080/17538068.2016.1235531. [ DOI ] [ Google Scholar ]
- 90. Reyna V.F. Risk perception and communication in vaccination decisions: A fuzzy-trace theory approach. Vaccine. 2012;30:3790–3797. doi: 10.1016/j.vaccine.2011.11.070. [ DOI ] [ PMC free article ] [ PubMed ] [ Google Scholar ]
- 91. Attwell K., Smith D.T. Parenting as politics: Social identity theory and vaccine hesitant communities. Int. J. Health Gov. 2017;22:183–198. doi: 10.1108/IJHG-03-2017-0008. [ DOI ] [ Google Scholar ]
- 92. Brunson E.K. The impact of social networks on parents’ vaccination decisions. Pediatrics. 2013;131:e1397–e1404. doi: 10.1542/peds.2012-2452. [ DOI ] [ PubMed ] [ Google Scholar ]
- 93. Weigmann K. An injection of confidence: Scientists explore new and old methods to counter anti-vaccine propaganda and overcome vaccine hesitancy so as to increase vaccination rates. EMBO Rep. 2017;18:21–24. doi: 10.15252/embr.201643589. [ DOI ] [ PMC free article ] [ PubMed ] [ Google Scholar ]
- 94. Brewer N.T., Chapman G.B., Rothman A.J., Leask J., Kempe A. Increasing Vaccination: Putting Psychological Science into Action. Psychol. Sci. Public Interest. 2017;18:149–207. doi: 10.1177/1529100618760521. [ DOI ] [ PubMed ] [ Google Scholar ]
- 95. Goldstein S., Macdonald N.E., Guirguis S. Health communication and vaccine hesitancy. Vaccine. 2015;33:4212–4214. doi: 10.1016/j.vaccine.2015.04.042. [ DOI ] [ PubMed ] [ Google Scholar ]
- 96. Dubé E., Gagnon D., Macdonald N.E. Strategies intended to address vaccine hesitancy: Review of published reviews. Vaccine. 2015;33:4191–4203. doi: 10.1016/j.vaccine.2015.04.041. [ DOI ] [ PubMed ] [ Google Scholar ]
- 97. Harvey H., Reissland N., Mason J. Parental reminder, recall and educational interventions to improve early childhood immunisation uptake: A systematic review and meta-analysis. Vaccine. 2015;33:2862–2880. doi: 10.1016/j.vaccine.2015.04.085. [ DOI ] [ PubMed ] [ Google Scholar ]
- 98. Brown K.F., Kroll J.S., Hudson M.J., Ramsay M., Green J., Long S.J., Vincent C.J., Fraser G., Sevdalis N. Factors underlying parental decisions about combination childhood vaccinations including MMR: A systematic review. Vaccine. 2010;28:4235–4248. doi: 10.1016/j.vaccine.2010.04.052. [ DOI ] [ PubMed ] [ Google Scholar ]
- 99. McKee C., Bohannon K. Exploring the Reasons Behind Parental Refusal of Vaccines. J. Pediatr. Pharmacol. Ther. 2016;21:104–109. doi: 10.5863/1551-6776-21.2.104. [ DOI ] [ PMC free article ] [ PubMed ] [ Google Scholar ]
- 100. Fadda M., Galimberti E., Fiordelli M., Romanò L., Zanetti A., Schulz P.J. Effectiveness of a smartphone app to increase parents’ knowledge and empowerment in the MMR vaccination decision: A randomized controlled trial. Hum. Vaccines Immunother. 2017;13:2512–2521. doi: 10.1080/21645515.2017.1360456. [ DOI ] [ PMC free article ] [ PubMed ] [ Google Scholar ]
- 101. Shelby A., Ernst K. Story and science: How providers and parents can utilize storytelling to combat anti-vaccine misinformation. Hum. Vaccines Immunother. 2013;9:1795–1801. doi: 10.4161/hv.24828. [ DOI ] [ PMC free article ] [ PubMed ] [ Google Scholar ]
- 102. Shoup J.A., Wagner N.M., Kraus C.R., Narwaney K.J., Goddard K.S., Glanz J.M. Development of an Interactive Social Media Tool for Parents With Concerns About Vaccines. Health Educ. Behav. 2014;42:302–312. doi: 10.1177/1090198114557129. [ DOI ] [ PMC free article ] [ PubMed ] [ Google Scholar ]
- 103. Dempsey A.F., Wagner N., Narwaney K., Pyrzanowski J., Kwan B.M., Kraus C., Gleason K., Resnicow K., Sevick C., Cataldi J., et al. ‘Reducing Delays In Vaccination’ (REDIVAC) trial: A protocol for a randomised controlled trial of a web-based, individually tailored, educational intervention to improve timeliness of infant vaccination. BMJ Open. 2019;9:e027968. doi: 10.1136/bmjopen-2018-027968. [ DOI ] [ PMC free article ] [ PubMed ] [ Google Scholar ]
- 104. Sadique Z., Devlin N., Edmunds W.J., Parkin D. The Effect of Perceived Risks on the Demand for Vaccination: Results from a Discrete Choice Experiment. PLoS ONE. 2013;8:e54149. doi: 10.1371/journal.pone.0054149. [ DOI ] [ PMC free article ] [ PubMed ] [ Google Scholar ]
- 105. Seeber L., Conrad T., Hoppe C., Obermeier P., Chen X., Karsch K., Muehlhans S., Tief F., Böttcher S., Diedrich S., et al. Educating parents about the vaccination status of their children: A user-centered mobile application. Prev. Med. Rep. 2017;5:241–250. doi: 10.1016/j.pmedr.2017.01.002. [ DOI ] [ PMC free article ] [ PubMed ] [ Google Scholar ]
- 106. Wagner N.M. Doctoral Dissertation. University of Colorado; Boulder, CO, USA: 2019. Assessing the Value of the Vaccine Social Media Intervention through the Re-aim Framework Implementation Dimension. [ Google Scholar ]
- 107. Moyer-Gusé E., Robinson M.J., McKnight J. The Role of Humor in Messaging about the MMR Vaccine. J. Health Commun. 2018;23:514–522. doi: 10.1080/10810730.2018.1473533. [ DOI ] [ PubMed ] [ Google Scholar ]
- 108. Haydarov R., Gordon J.C. Effect of combining attribute and goal framing within messages to change vaccination behavior. J. Commun. Health. 2015;8:45–54. doi: 10.1179/1753807615Y.0000000005. [ DOI ] [ Google Scholar ]
- 109. Kennedy A., Glasser J., Covello V., Gust D. Development of Vaccine Risk Communication Messages Using Risk Comparisons and Mathematical Modeling. J. Health Commun. 2008;13:793–807. doi: 10.1080/10810730802487463. [ DOI ] [ PubMed ] [ Google Scholar ]
- 110. O’Keefe D.J., Nan X. The Relative Persuasiveness of Gain- and Loss-Framed Messages for Promoting Vaccination: A Meta-Analytic Review. Health Commun. 2012;27:776–783. doi: 10.1080/10410236.2011.640974. [ DOI ] [ PubMed ] [ Google Scholar ]
- 111. Nyhan B., Reifler J., Richey S., Freed G.L. Effective Messages in Vaccine Promotion: A Randomized Trial. Pediatrics. 2014;133:e835–e842. doi: 10.1542/peds.2013-2365. [ DOI ] [ PubMed ] [ Google Scholar ]
- 112. Amin A.B., Bednarczyk R.A., Ray C.E., Melchiori K.J., Graham J., Huntsinger J.R., Omer S.B. Association of moral values with vaccine hesitancy. Nat. Hum. Behav. 2017;1:873–880. doi: 10.1038/s41562-017-0256-5. [ DOI ] [ PubMed ] [ Google Scholar ]
- 113. Lorini C., Santomauro F., Donzellini M., Capecchi L., Bechini A., Boccalini S., Bonanni P., Bonaccorsi G. Health literacy and vaccination: A systematic review. Hum. Vaccines Immunother. 2017;14:478–488. doi: 10.1080/21645515.2017.1392423. [ DOI ] [ PMC free article ] [ PubMed ] [ Google Scholar ]
- 114. Ratzan S.C. Vaccine Literacy: A New Shot for Advancing Health. J. Health Commun. 2011;16:227–229. doi: 10.1080/10810730.2011.561726. [ DOI ] [ PubMed ] [ Google Scholar ]
- 115. Nowak G.J., Gellin B.G., Macdonald N.E., Butler R. Addressing vaccine hesitancy: The potential value of commercial and social marketing principles and practices. Vaccine. 2015;33:4204–4211. doi: 10.1016/j.vaccine.2015.04.039. [ DOI ] [ PubMed ] [ Google Scholar ]
- 116. Stockwell M.S., Fiks A.G. Utilizing health information technology to improve vaccine communication and coverage. Hum. Vaccines Immunother. 2013;9:1802–1811. doi: 10.4161/hv.25031. [ DOI ] [ PMC free article ] [ PubMed ] [ Google Scholar ]
- View on publisher site
- PDF (950.2 KB)
- Collections
Similar articles
Cited by other articles, links to ncbi databases.
- Download .nbib .nbib
- Format: AMA APA MLA NLM
Add to Collections

COMMENTS
Vaccines have revolutionized modern medicine by preventing infectious diseases and safeguarding public health. This Collection showcases cutting-edge research on advancements in vaccine ...
The Dale and Betty Bumpers Vaccine Research Center (VRC) at the National Institutes of Health (NIH) was established to facilitate research in vaccine development. The VRC is dedicated to improving global human health through the rigorous pursuit of effective vaccines for human diseases. Established by former President Bill Clinton as part of an ...
Bacterial Polysaccharides, Enteric & Sexually Transmitted Diseases, Mycobacterial Diseases & Cellular Immunology, Respiratory & Special Pathogens, DNA Viruses,
By focusing on research to develop vaccine candidates and monoclonal antibodies, the ReVAMPP network will lay the groundwork for a faster and more effective pandemic response should a virus from one of the targeted families emerge as a pandemic threat. ... Project title: Vaccines and Therapeutic Antibodies to Respiro, Rubula, Peribunya and ...
Research Open Access 29 Oct 2024 npj Vaccines Volume: 9, P: 205 Electroporation-mediated novel albumin-fused Flt3L DNA delivery promotes cDC1-associated anticancer immunity
In Fig. 2, the overview of the global COVID-19 vaccine landscape in clinical development depicts that there are seven major types of vaccine candidates for COVID-19 is illustrated as (inactivated, non-replicating viral vectors, replicating viral vectors, protein subunit, nucleic acid-based, and virus-like particles [VLP]), showing the percentage of candidate vaccines that are currently under ...
Single reviewers assessed titles, abstracts, and full-text articles, and extracted data, with a second reviewer verifying included studies. ... Previous research has suggested that vaccine effectiveness of COVID-19 vaccines for symptomatic infections might be higher than for asymptomatic infections; 101 although, little work has been done to ...
Throughout 2020 and 2021, the global response to COVID-19 demonstrated the importance of vigilance and preparedness for infectious diseases. In this context, there was a marked demand placed on vaccine research and development (R&D), with researchers, federal agencies, public health institutions, manufacturers, and regulators being rapidly mobilized to develop an effective vaccine as quickly ...
Preparing for the next pandemic before it starts. This article, about the concept of universal vaccines, inaugurates a new periodic feature of NIH Research Matters called Research in Context.These in-depth articles will focus on cutting-edge NIH biomedical research topics, describing the current state of research in a field and where it may be heading.
Authorship impact on COVID-19 vaccine research. Of the 618 authors who contributed to the top 100 most-cited articles, 556 published just one article, about 90% of all authors. Table 1 shows only 17 authors with ≥ 3 publications, of which 10 were from the USA, 3 from Israel, 2 from Germany, and 2 from the UK. In addition, we confirmed that ...
Krubiner, C. B. et al. Pregnant women and vaccines against emerging epidemic threats: ethics guidance for preparedness, research, and response. Vaccine 39, 85-120 (2021).
Regional priorities for research and innovation, as stated in the Immunization Agenda 2030 or Global Vaccine Action Plan-related strategic plans, most commonly focus on implementation and operational research, and other research to support vaccine introduction decision-making and maximize the benefits of vaccines or efficiency of vaccination ...
Now, given the urgent need for COVID-19 vaccines, unprecedented financial investments and scientific collaborations are changing how vaccines are developed. This means that some of the steps in the research and development process have been happening in parallel, while still maintaining strict clinical and safety standards.
It's hard to overstate the benefits that innovative vaccines deployed in the past five decades have had on morbidity and mortality (see timeline). 1 The incidence of vaccine-preventable diseases ...
A recent test-negative design study in Canada showed positive findings regarding vaccine effectiveness with the BNT162b2, mRNA-1273, and ChAdOx1 nCoV-19 vaccines against symptomatic disease with ...
Vaccine clinical research needs to deal with certain ethical issues because of the inherent nature of these trials. The issues are more complicated since the research mostly happens in pediatric populations in developing countries. Keeping in mind these issues while designing research on vaccines is critical.
News on vaccines including HPV vaccine, shingles vaccine, bird flu vaccines and more. Read the latest research on vaccination risks and complications.
Dr. Barouch can be contacted at [email protected] or at the Center for Virology and Vaccine Research, E/CLS-1043, 330 Brookline Ave., Beth Israel Deaconess Medical Center, Boston, MA 02115.
Abstract. Parental vaccine hesitancy is becoming an increasingly important public health concern in the United States. In March 2020, an assessment of the latest CDC National Immunization Survey data found that more than one-third of U.S. children between the ages of 19 and 35 months were not following the recommended early childhood immunization schedule.
New approach to growing coronaviruses is research boon—but also raises safety concerns Artificial virus receptors could help researchers find vaccines and treatments, but some worry about risk of lab leaks. ... Such studies might help develop new antivirals and vaccines, says Yan, who adds that the technique could also provide new insights ...
Vaccine research is a broad field that includes many specialties. Researching job listings allows you to explore potential career paths and determine the one that suits your interests and professional aspirations. Defining your goal can help you choose educational programs to prepare you for a vaccine research career. 2. Earn a bachelor's degree
BioVaxys to Participate in the US Government Biomedical Advanced Research and Development Authority (BARDA) Rapid Response Partnership Vehicle (RRPV) Vaccine Development Consortium News provided by
VANCOUVER, BC, Nov. 5, 2024 /PRNewswire/ -- BioVaxys Technology Corp. (CSE: BIOV) (FRA: 5LB) (OTCQB: BVAXF) ("BioVaxys" or the "Company") is pleased to announce that it has been invited to and ...