- Open access
- Published: 10 May 2024

Incidence, risk factors and outcomes of nosocomial infection in adult patients supported by extracorporeal membrane oxygenation: a systematic review and meta-analysis
- Ali Ait Hssain ORCID: orcid.org/0000-0002-7764-5793 1 , 2 , 3 ,
- Amir Vahedian-Azimi ORCID: orcid.org/0000-0002-1678-7608 4 ,
- Abdulsalam Saif Ibrahim ORCID: orcid.org/0000-0003-1181-909X 1 , 2 ,
- Ibrahim Fawzy Hassan ORCID: orcid.org/0000-0002-0283-125X 1 , 2 ,
- Elie Azoulay ORCID: orcid.org/0000-0002-8162-1508 5 &
- Michael Darmon ORCID: orcid.org/0000-0003-4198-8038 5
Critical Care volume 28 , Article number: 158 ( 2024 ) Cite this article
1129 Accesses
13 Altmetric
Metrics details
An increasing number of patients requires extracorporeal membrane oxygenation (ECMO) for life support. This supportive modality is associated with nosocomial infections (NIs). This systematic review and meta-analysis aim to assess the incidence and risk factors of NIs in adult.
We searched PubMed, Scopus, Web of Science, and ProQuest databases up to 2022. The primary endpoint was incidence of NI. Secondary endpoints included time to infection, source of infection, ECMO duration, Intensive care and hospital length of stay (LOS), ECMO survival and overall survival. Incidence of NI was reported as pooled proportions and 95% confidence intervals (CIs), while dichotomous outcomes were presented as risk ratios (RR) as the effective index and 95% CIs using a random-effects model.
Among the 4,733 adult patients who received ECMO support in the 30 included studies, 1,249 ECMO-related NIs per 1000 ECMO-days was observed. The pooled incidence of NIs across 18 studies involving 3424 patients was 26% (95% CI 14–38%).Ventilator-associated pneumonia (VAP) and bloodstream infections (BSI) were the most common NI sources. Infected patients had lower ECMO survival and overall survival rates compared to non-infected patients, with risk ratio values of 0.84 (95% CI 0.74–0.96, P = 0.01) and 0.80 (95% CI 0.71–0.90, P < 0.001), respectively.
Results showed that 16% and 20% lower of ECMO survival and overall survival in patients with NI than patients without NI, respectively. However, NI increased the risk of in-hospital mortality by 37% in infected patients compared with non-infected patients. In addition, this study identified the significant positive correlation between ECMO duration and ECMO-related NI.
Extracorporeal membrane oxygenation (ECMO), also known as extracorporeal life support, is an advanced life support modality for critically-ill patients with severe but reversible cardiac and/or respiratory failure [ 1 ]. Despite improvements in both technology and management of ECMO, this technique is associated with specific risks and complications [ 2 ]. As consequences, many patients treated with ECMO face life-threatening complications such as bleeding, coagulopathy, thrombosis, infection, and stroke [ 3 , 4 ].
Nosocomial infections (NI) are a common complication in patients treated with ECMO [ 5 , 6 ]. Main sources of ECMO-related NI include bloodstream infections (BSIs), urinary tract infections (UTIs), surgical site infections (SSIs), and ventilator-associated pneumonia (VAP) [ 7 , 8 ]. In addition to typical ECMO-related NI, specific ECMO-related infections, such as localized infections at peripheral cannulation insertion sites or mediastinitis in the setting of central cannulation also exists [ 9 , 10 , 11 ]. In studies examining different ECMO modalities, including (veno-venous) VV ECMO for respiratory failure and (veno-arterial) VA ECMO for cardiogenic shock, the infection risk was found to range from 8 to 64% [ 12 , 13 , 14 , 15 ]. Moreover, previous studies have suggested that NIs during ECMO may be related to some predisposing factors, including patients’ underlying condition, the severity of illness, and immunocompromised [ 16 , 17 , 18 ]. However, to date, there is no unified understanding of ECMO-related NI from diagnosis to treatment or prevention.
Significant heterogeneity may be expected from existing studies due to differences in case-mix, monocentric design of the performed studies, and inclusion of various ECMO modalities. This systematic review and meta-analysis aim to investigate the incidence of ECMO-related NIs as well as to examine ECMO survival, overall survival and the risk factors related to NI in published studies.
Study design
This systematic review and meta-analysis were performed according to predefined eligibility criteria, search strategies, criteria for study selection and methods for extracting data. It was performed according following the Preferred Reporting Items for Systematic review and Meta-Analysis Protocols (PRISMA-P) 2020 statement [ 19 ]. The predefined protocol was registered in the International Prospective Register of Systematic Reviews (PROSPERO) database (CRD42023372412).
Search strategy and inclusion exclusion criteria
Electronic databases, including PubMed/MEDLINE, Scopus, Web of Science and ProQuest were searched from inception until 1st November 2022. English language publications reporting outcome and clinical characteristics of NI in adult patients receiving ECMO for more than 24 h were selected. To further identify articles for inclusion, all relevant studies and their citations list were examined. The full search strategy is available in Supplementary file 1, Table S1.
The PICOS (Population, Intervention, Comparison, Outcome, and Study type) mnemonic was used for synthesis in this meta-analysis to defined inclusion criteria [ 20 ]. Studies were eligible if they met all of the following inclusion criteria: (a) Population: adult (≥ 18 years) patients, male or female; (b) Intervention: supported by ECMO ≥ 24 h; (c) Comparison: compare NI patients with non-NI patients; (d) Outcomes: primary outcome indicators were the prevalence and incidence of NI, and secondary outcome indicators were ECMO survival, survival to hospital discharge, ECMO duration, ICU length of stay (LOS), hospital LOS, microorganism species causing ECMO-related NI, risk factors related to NI and related clinical characteristics of NI and (e) Study type: published retrospective or prospective cohort study. Studies were excluded if (a) studies enrolled patients who had been co-infected before receiving ECMO treatment; (b) studies without access to the full text, publication on animal experiments, review articles, letters-to-the-editor, editorial, case report and conference papers; (c) studies published in non- English languages.
A first screening was performed by title and abstract to identify seemingly related articles. A second screening was performed on selected article after complete assessment of the manuscripts. At each step, assessment was performed independently by two authors (A. AH and A.VA). Disagreement was resolved by discussion and if needed by adjudication by a third author. The final agreement between the three evaluating authors was assessed through Kendall's coefficient of agreement (r = 0.92; P < 0.001). Data were extracted from the included studies using a pre-designed form (Supplementary file 2, sheet 1). Moreover, the methodological quality of included manuscripts was assessed [ 21 , 22 ].
Quality appraisal
The methodological quality of the included manuscripts was assessed using the JBI critical appraisal tool for cohort studies.. The tool evaluates cohort studies based on 11 criteria, with responses recorded as “Yes”, “No”, “Unclear”, or “Not Applicable”. After evaluating all components of the study, an overall rating was determined based on the number of “Yes” responses: good (≥ 8 yes), medium (5–7 yes), or poor (≤ 4 yes). In addition, the Cochrane Risk of Bias in Observational Studies of Exposures (ROBINS-E) tool was used to evaluate the risk of bias of the included studies [ 21 , 22 ]. The ROBINS-E tool assesses 7 domains of bias: confounding, selection of participants into the study, classification of exposures, departures from intended exposures, missing data, measurement of outcomes and, selection of the reported result. Domains are classified as low risk of bias, high risk of bias, or unclear risk of bias [ 23 ].
Definition of NI and survival rates
ECMO-related NI was defined according to the Center for Disease Control and Prevention (CDC) as an infection occurring > 24 h after initiation and < 48 h after discontinuation of ECMO [ 24 , 25 , 26 ]. Various types of NIs include blood stream infection (BSI), respiratory tract infection (RTI), urinary tract infection (UTI), surgical site infection (SSI), cannula site infection (CSI), and ventilator-associated pneumonia (VAP) [ 27 , 28 ]. The overall survival rate was defined as the percentage of patients with ECMO who survived to discharge from the hospital out of the total number of patients who received ECMO.
Primary and secondary outcomes
The primary outcome of this study was the incidence (NI per 1000 ECMO days) of different types of NI in adult patients receiving ECMO. The secondary outcomes included incidence (number of patients developing ≥ 1 episode of NI), time to infection, sources of infection, pathogens, duration of ECMO, ICU and hospital length of stay, ECMO and hospital survival rate.
Statistical analysis
Descriptive results were reported as percentages, mean ± standard deviation (SD) or median with interquartile range (IQR) calculated from the total number of patients in the analysis. GraphPad Prism 9© (GraphPad Software Inc., La Jolla, CA) and Excel program was used for and forest plots and graphs.
Incidence of NIs and its different types as primary outcomes were reported as pooled proportions and their 95% confidence intervals (CIs), while dichotomous outcomes were presented as pooled risk ratios (RR) and their 95% CIs. In addition, subgroups analysis was carried out based on countries. Due to methodologic variations and sample diversity across studies, the random-effects Linear Mixed Models (REML) was used to extract the pooled estimate. We applied the fixed effect model when the data were homogeneous.
Heterogeneity was assessed using the I-squared (I 2 ) statistic, and significance results of the test and values > 50% for I 2 indicated substantial heterogeneity and the corresponding p-values < 0.05 were also considered as significant [ 29 ]. In analyses with significant heterogeneity, a sensitivity analysis and meta-regression analysis were conducted to check the source of heterogeneity. In addition, we used the Galbraith plot to examine heterogeneity [ 30 ]. Risk of publication bias was evaluated by visual inspection of funnel plots, the Egger [ 31 ] and Begg [ 32 ] test were also conducted. Moreover, a nonparametric trim-and-fill method of assessing publication bias was conducted and if there was a publication bias the modified effect size was estimated after adjusting [ 33 ]. Finally, we assessed the effect of individual studies on ES, using cumulative analysis based on publication year. Statistical analyses were performed on Review Manager (RevMan) version 5, and STATA version 17 (Stata Corp; College Station; TX, USA). All tests were two-sided y and p-values lower than 0.05 was considered significant.
Literature search and manuscript selection
The search strategy included PubMed/Medline ( n = 413), Web of Science ( n = 493), ProQuest ( n = 2) and Scopus ( n = 808) databases resulting in 1,716 studies. After removing duplicates ( n = 484) and irrelevant studies ( n = 1169), 63 articles remained for full-text evaluation. Of these, 33 studies were excluded due to an inadequate study population ( n = 11), inappropriate study design ( n = 11) or lack of relevant outcome ( n = 10) (Fig. 1 ). Details of the 33 excluded studies and the cause for their exclusion are available in Supplementary file 2, sheet 2.
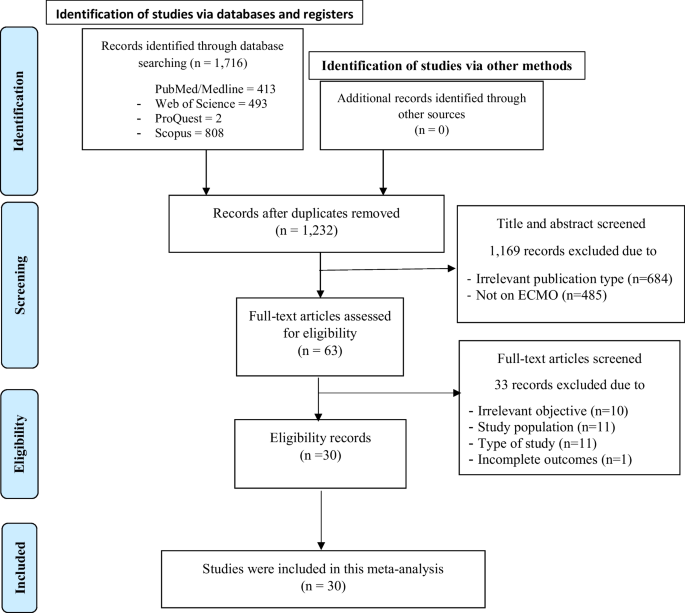
The literature search results and the screening process based on PRISMA 2020 flowchart
Quality appraisal results
According to the results of quality assessment, most 24/30 (80%) studies had good quality [ 6 , 8 , 10 , 12 , 13 , 14 , 16 , 34 , 35 , 36 , 37 , 38 , 39 , 40 , 41 , 42 , 43 , 44 , 45 , 46 , 47 , 48 , 49 , 50 ], and only 6/30 (20%) studies had moderate quality [ 17 , 51 , 52 , 53 , 54 , 55 ] (Supplementary file 1, Table S2). In addition, 100% of included studies were classified as having low risk of bias based on Cochrane ROBINS-E tool (Supplementary file 1, Table S3–S4).
Characteristics of included studies.
Thirty studies with 4733 participants were included. Every one of these studies was of retrospective observational design. Of these participants, 65.4% (3097) were male and the median age was 50 (Range: 18–77). Among the included studies, only one was a multi-center retrospective study [ 55 ], while the rest were single-center retrospective studies. Main reason to initiate ECMO was need for cardiac support (63.1%, 2548), respiratory support (33.6%, n = 1356), and other causes for the remaining 3.3% ( n = 135). Most of the studies included both VV ECMO and VA ECMO, four studies focusing only on VA ECMO [ 12 , 16 , 42 , 51 ], and three studies on VV ECMO [ 37 , 46 , 54 ] (Tables 1 and 2 ).
Descriptive results
Clinical outcomes available in included studies are reported in Supplementary file 1, Table S5–S9. A comprehensive analysis of 30 studies involving 4733 adult patients on ECMO treatment revealed that there were 1249 ECMO-related NIs per 1000 ECMO-days. Males accounted for 60.93% of infected patients, with an average age of 53.17 ± 13.95 years. Hypertension was the most common underlying condition in both infected and non-infected patients (Supplementary file 1, Figure S1). Patients with NIs had significantly longer ECMO, ICU, and hospital stays (Supplementary file 1, Figure S1). The total incidence range of NIs was 4.1–85.4% with 2059 pathogens identified from 1,498 NI episodes in 1249 infected patients. The incidence of ECMO-related NI was 2.98–24.7% for BSI, 3.97–17% for SSI, 3.97–24.7% for RTI, 1.99–31% for UTI, 23.9–55.4% for VAP, and 7.1–11% for CSI. Gram-negative bacteria were identified as the most prevalent pathogens (48.6%), followed by Gram-positive bacteria and fungi. Acinetobacter baumannii , Pseudomonas aeruginosa , and Klebsiella pneumoniae were the most common Gram-negative bacteria, while Enterococcus spp ., Coagulase-negative Staphylococcus , and Staphylococcus aureus were the predominant Gram-positive bacteria.
Risk factors for NI
Results showed that the MV duration, hospital LOS, ECMO mode (VV ECMO vs. VA ECMO), having underlying diseases (yes vs. no), mechanical complication, SOFA score, SAPS score, ECMO catheter colonization, age, duration of arterial catheter, acute renal failure, acute hepatic failure, body mass index (MBI), cardiopulmonary resuscitation (CPR) < 5 min and hemodialysis were significantly increased the risk of NI (Supplementary file 1, Figure S2B). According to pooled analysis in the current study, the cumulative odds ratio of ECMO duration to predict NI was 1.05 (95%CI 1.02–1.08, P < 0.001), with substantial significant heterogeneity between studies (I 2 = 98.8%, P < 0.001) (Supplementary file 1, Figure S3).
Primary outcomes
The pooled incidence rate of NIs, as reported in 18 studies involving 3,424 patients, was found to be 0.26 (95% CI 0.14–0.38, P < 0.001), indicating a statistically significant result. However, there was substantial heterogeneity observed between the studies (I 2 = 91.8%, P < 0.001) (Fig. 2 A). To address this heterogeneity, a sensitivity analysis was conducted where the study or studies causing the heterogeneity were excluded. Upon recalculating, the adjusted pooled incidence of NI (based on 13 studies and 2,761 patients) was determined to be 0.12 (95% CI 0.07–0.16, P < 0.001) with a mild heterogeneity (I 2 = 35.7%, P = 0.01) (Fig. 2 B).
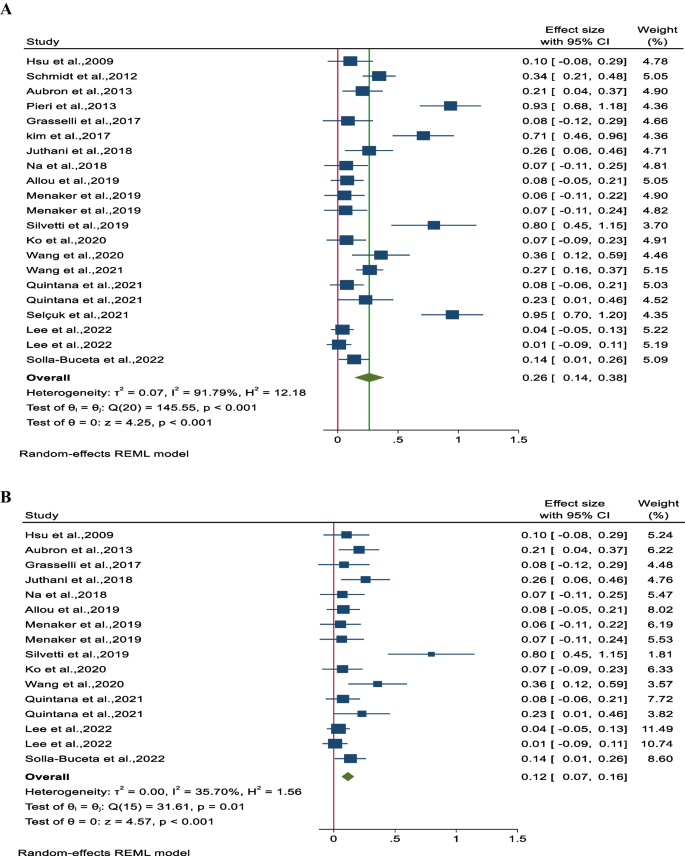
Forest plot for A pooled incidence NIs per 1000 ECMO-day and B pooled incidence of NIs per 1000 ECMO-day after reducing heterogeneity
Secondary outcomes
Ecmo survival.
The survival rate of patients undergoing combined ECMO treatment was determined to be 62% (95% CI 54–70; 11 studies involving 1651 participants). Notably, there was substantial heterogeneity among the studies (I 2 = 65.8%, P < 0.001 (Fig. 3 A). The impact of nosocomial infections on ECMO survival was assessed in 10 studies involving 1613 patients. It was found that ECMO survival rates were significantly lower in patients with NIs, with a pooled risk ratio (RR) of 84% (95% CI 74–96%). A moderate level of heterogeneity was observed among the studies (I 2 = 42.5%, P = 0.05) (Fig. 3 B).
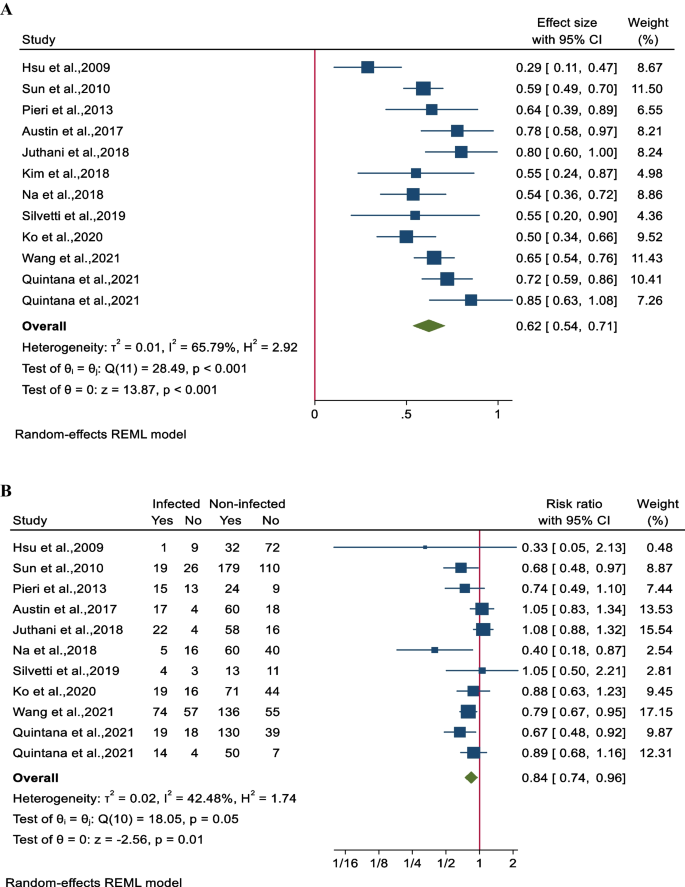
Forest plot for pooled ECMO survival rates for A all participants in each study and B between infected and non-infected patients
Overall survival
The overall survival rate was determined to be 54% (95% CI 49–59; 11 studies involving 1651 participants). Notably, there was significant heterogeneity observed among the studies (I 2 = 64.5%, P < 0.001; Fig. 4 A). Comparing the overall survival rates between the nosocomial infection (NI) group and control patients revealed a substantial difference, with the NI group showing a lower survival rate of 80% (95% CI 71–90; 24 studies involving 4205 patients). There was also notable heterogeneity among the studies (I 2 = 53.7%, P < 0.001, Fig. 4 B). Additionally, detailed subgroup analysis, sensitivity analysis, and assessment of publication bias can be found in Supplementary File 3, Figs. 1A–5D.
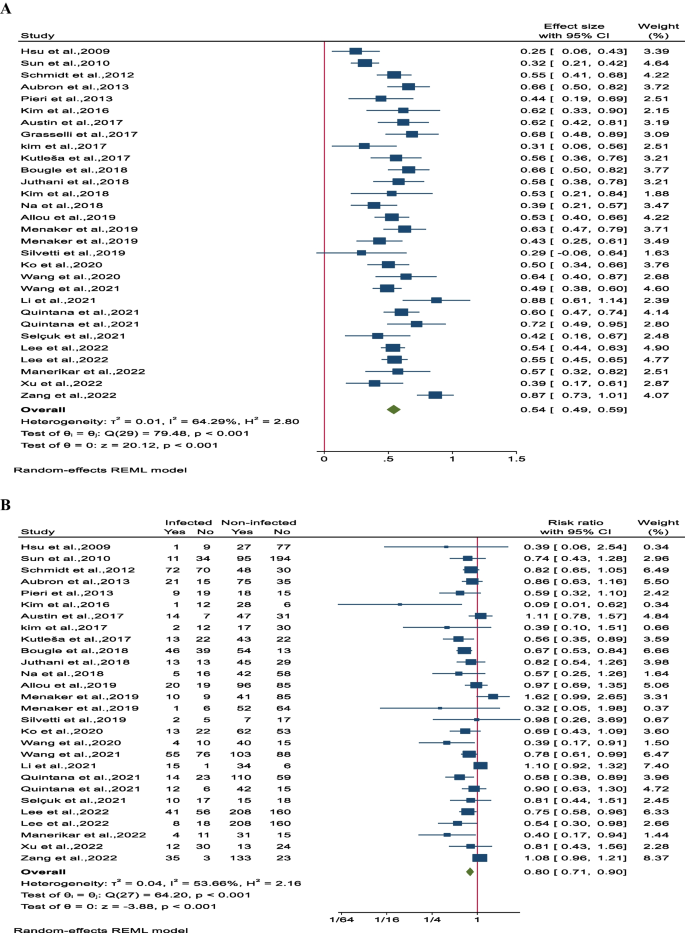
Forest plot of overall survival rates for A all participants in each study and B between infected and non-infected patient
Influence of NI on outcome was not affected by publication date (Supplementary file 1, Figure S4A). NI rate was however associated with the publication date (Supplementary file 1, Figure S4B). However, overall survival was lower among studies published from 2009 to 2013 (Supplementary file 1, Figure S5A–S5B).
Meta-regression
In order to explore the heterogeneity, a meta-regression analysis was conducted. Mortality was found to be linked to factors such as patients' severity of illness based on APACHE II scores, age, and VV ECMO, while ECMO survival was associated with nosocomial infections. The findings of the meta-regression analysis are presented in Supplementary File 1, Table S10. Additionally, a forest plot depicting the impact of these variables on the outcomes is included in Supplementary File 4, Figures S1–S19.
This systematic review and meta-analysis aimed to assess the overall incidence of ECMO-related NIs and their impact on mortality, offering a comprehensive evaluation. Across 18 studies involving 3,424 patients, the pooled incidence of NIs was 26%. The time to the first NI ranged from 3 to 15.6 days after ECMO initiation, with a notable number of patients developing NIs beyond two weeks [ 16 , 17 , 44 ]. The incidence of NIs varied widely among studies, ranging from 4.1% to 85.4%. This variability could be attributed to factors like case mix, diagnostic criteria, reporting systems, antibiotic prophylaxis strategies, and center-specific effects [ 5 , 56 , 57 ].
The incidence of ECMO-related NIs and their impact on outcomes in patients supported by ECMO have been previously reported in literature. Studies have shown that the rate of infection can vary, with reports ranging from 8 to 46%. Previous reviews of the Extracorporeal Life Support Organization (ELSO) registry by Bizzarro et al. [ 58 ], and Vogel et al. [ 59 ], found rates of infection to be 11.7% and 10.2%, respectively, which is lower than the rates seen in our study. This discrepancy among studies may be attributed to differences in study populations, methodologies, variations in infection prevention practices, the emergence of new pathogens and antimicrobial resistance, as well as improvements in surveillance and reporting methods [ 60 , 61 ].
Overall, 2059 pathogens were isolated from 1498 NI episodes in 1249 (26.4%) infected patients. Our findings identified VAP (33%), BSI (15%), and RTI (15%) as the most common ECMO-related NIs, primarily caused by GNB like Acinetobacter baumannii, Pseudomonas aeruginosa, and Klebsiella pneumoniae . Studies indicate VAP rates ranging from 10.7 to 54.5%, mainly attributed to GNB such as Pseudomonas aeruginosa , Klebsiella pneumoniae , and Acinetobacter species , and GPB like Staphylococcus aureus [ 8 , 16 , 17 , 40 , 47 , 51 ]. BSI prevalence in adult ECMO patients ranges from 2.6 to 44.7%, with GBP, especially coagulase-negative staphylococci and Staphylococcus aureus , being the primary pathogens, followed by GNB (10–20%) such as Acinetobacter baumannii and Pseudomonas aeruginosa , and fungal infections like Candida spp. [ 13 , 37 , 39 ]. RTI rates vary from 1.1 to 32.1%, primarily caused by GNB like Klebsiella pneumoniae , Pseudomonas aeruginosa , and Haemophilus influenza [ 16 , 51 ].
The study, consistent with the previous work by Li et al. [ 5 ], identified several risk factors for NIs in adult patients undergoing ECMO. These risk factors included the duration of MV, length of hospital stay, ECMO mode, underlying diseases, disease severity, ECMO catheter colonization, patient age, duration of arterial catheter placement, acute renal failure, acute hepatic failure, BMI, ECPR exceeding 5 min, hemodialysis, and mechanical complications. Patients supported by VV ECMO exhibited a higher susceptibility to developing Nosocomial Infections (NIs) compared to those on VA ECMO [ 14 , 41 , 47 ]. Despite this, the VA ECMO modality is recognized for its increased complexity, entailing higher risks of vascular trauma, systemic embolization, and ischemia [ 62 ]. The exact reason behind the heightened NI risk in VV ECMO patients remains somewhat ambiguous. This elevated risk may be linked to the prolonged ECMO treatment and duration of ventilator support in VV ECMO patients [ 14 , 41 ]. Additionally, the longer duration of VV ECMO in lung transplant recipients inherently exposes them to an extended period of susceptibility to NIs, potentially leading to skewed infection rates and outcomes when contrasted with heart transplant patients supported by VA ECMO with shorter durations of support. The study highlights a significant association between NIs and adverse outcomes in adult ECMO patients, resulting in a relative risk reduction of 16% in ECMO survival rates and 20% in overall survival rates. Moreover, NIs were found to elevate the relative risk of hospital mortality, particularly in cases of prolonged ECMO duration, which showed a potential four-fold increase in NI risk [ 34 , 37 , 38 , 42 , 45 , 47 , 52 , 55 ].This heightened risk can be attributed to the critical condition of patients on long-term ECMO, prolonged exposure to risks, and the intensity of invasive care. Time-dependent bias is a critical consideration in studies involving ECMO duration and nosocomial infections. In the context of ECMO, the duration of ECMO support can act both as a risk factor for developing infections and as a consequence of infection occurrence. Prolonged ECMO duration has been associated with an increased risk of nosocomial infections due to factors such as prolonged exposure to invasive devices, prolonged hospitalization, and compromised immune function [ 63 ]. Longer ECMO duration not only increases the likelihood of acquiring infections but can also be a consequence of infections that prolong the need for ECMO support. This bidirectional relationship underscores the complexity of managing infections in ECMO patients and emphasizes the need for vigilant monitoring, infection prevention strategies, and timely interventions to mitigate the risks associated with prolonged ECMO support.
The observed increase in NI rates in more recent studies [ 45 , 46 , 47 , 48 ], despite older studies showing lower survival rates is indeed a noteworthy finding [ 13 , 14 , 16 ]. This apparent discrepancy does not necessarily negate the conclusion that NIs can impact mortality in ECMO patients. Instead, it may reflect improved surveillance, detection, and reporting of NIs over time. One plausible explanation for this occurrence could be advancements in critical care practices and infection control measures over time. With improvements in healthcare protocols, including enhanced sepsis management, antimicrobial stewardship, and ECMO circuit technology, it is possible that while NI rates have risen in recent years, overall survival rates have improved due to better management of infections. Moreover, the evolving landscape of ECMO therapy, including patient selection criteria, cannulation techniques, and anticoagulation strategies, may have influenced both NI rates and patient outcomes over time. The study could be useful for clinicians and researchers regarding infection risk factors in ECMO patients. Further studies aiming at identifying high-risk patients are needed so that clinicians and researchers can pinpoint high-risk patients for tailored monitoring and interventions.
This study has several limitations that should be considered. Firstly, the retrospective and single-center nature of most included studies, along with small sample sizes, limits data availability on confounding factors and the establishment of appropriate exposure and comparison groups. Secondly, there was significant heterogeneity due to variations in case mix, nosocomial infection rates, and management practices across different centers. Thirdly, a notable limitation is the inadequate consideration of time dependency of nosocomial infections in most studies, potentially leading to misleading associations between ECMO/ICU duration and infection outcomes. Lastly, the potential impact of changes in sepsis definitions and management practices over the years on the identification of BSI in ECMO patients is a critical consideration. These evolving standards may introduce variability in how infections are identified and managed, which could affect the study's outcomes. To address these limitations, we have conducted sensitivity analyses to mitigate potential biases arising from these changes, ensuring the robustness of our results. These limitations highlight the need for future research to address these gaps and improve our understanding of the impact of NIs on patient outcomes.
This study highlights a heightened risk of NIs, particularly Ventilator-VAP, BSI, and RTI, in patients undergoing ECMO for refractory respiratory or cardiogenic failure. The pooled analysis revealed a 26% incidence rate per 1000 ECMO-days of NIs in adult ECMO patients. Our findings indicate a 16% and 20% lower ECMO survival and overall survival, respectively, in patients with NIs compared to those without. The dynamic nature of ECMO therapy, encompassing evolving patient selection criteria, cannulation techniques, and anticoagulation strategies, may have impacted both NI rates and patient outcomes. Further research is warranted to delve deeper into assessing the risk of nosocomial infections while considering time-dependent confounders, evaluating the efficacy of prevention strategies, and understanding their impact on both infection rates and outcomes.
Availability of data and materials
The data that support the findings of this study are available from the corresponding author upon reasonable request.
Abbreviations
- Extracorporeal membrane oxygenation
Nosocomial infections
Bloodstream infections
Urinary tract infections
Surgical site infections
Ventilator-associated pneumonia
Cannula site infection
Venoarterial extracorporeal membrane oxygenation
Venovenous extracorporeal membrane oxygenation
Length of stay
Confidence intervals
Risk ratios
Linear Mixed Models
Ventetuolo CE, Muratore CS. Extracorporeal life support in critically ill adults. Am J Respir Crit Care Med. 2014;190(5):497–508. https://doi.org/10.1164/rccm.201404-0736CI .
Article PubMed PubMed Central Google Scholar
Coco LV, Lorusso R, Raffa GM, Malvindi PG, Pilato M, Martucci G, et al. Clinical complications during veno-arterial extracorporeal membrane oxigenation in post-cardiotomy and non post-cardiotomy shock: still the achille’s heel. J Thorac Dis. 2018;10(12):6993–7004. https://doi.org/10.21037/jtd.2018.11.103 .
Kim JH, Pieri M, Landoni G, Scandroglio AM, Calabrò MG, Fominskiy E, et al. Venovenous ECMO treatment, outcomes, and complications in adults according to large case series: a systematic review. Int J Artif Organs. 2020;44(7):481–8. https://doi.org/10.1177/0391398820975408 .
Article PubMed Google Scholar
Zangrillo A, Landoni G, Biondi-Zoccai G, Greco M, Greco T, Frati G, et al. A meta-analysis of complications and mortality of extracorporeal membrane oxygenation. Crit Care Resusc. 2013;15(3):172–8.
PubMed Google Scholar
Li X, Wang L, Wang H, Hou X. Outcome and clinical characteristics of nosocomial infection in adult patients undergoing extracorporeal membrane oxygenation: a systematic review and meta-analysis. Front Public Health. 2022;10: 857873. https://doi.org/10.3389/fpubh.2022.857873 .
Li ZJ, Zhang DF, Zhang WH. Analysis of nosocomial infection and risk factors in patients with ECMO treatment. Infect Drug Resist. 2021;14:2403–10. https://doi.org/10.2147/IDR.S306209 .
Wang J, Christensen C, Siddique A, Merritt H, Cawcutt K. Characteristics of venous–venous extracorporeal membrane oxygenation related bloodstream infections. J Card Surg. 2022;37(5):1431–4. https://doi.org/10.1111/jocs.16361 .
Aubron C, Cheng AC, Pilcher D, Leong T, Magrin G, Cooper DJ, et al. Infections acquired by adults who receive extracorporeal membrane oxygenation: risk factors and outcome. Infect Control Hosp Epidemiol. 2013;34(1):24–30. https://doi.org/10.1086/668439 .
Thomas G, Hraiech S, Cassir N, Lehingue S, Rambaud R, Wiramus S, et al. Venovenous extracorporeal membrane oxygenation devices-related colonisations and infections. Ann Intensive Care. 2017. https://doi.org/10.1186/s13613-017-0335-9 .
Allou N, Lo Pinto H, Persichini R, Bouchet B, Braunberger E, Lugagne N, et al. Cannula-related infection in patients supported by peripheral ECMO: clinical and microbiological characteristics. ASAIO J. 2019;65(2):180–6. https://doi.org/10.1097/MAT.0000000000000771 .
Bull T, Corley A, Lye I, Spooner AJ, Fraser JF. Cannula and circuit management in peripheral extracorporeal membrane oxygenation: an international survey of 45 countries. PLoS ONE. 2019;14(12): e0227248. https://doi.org/10.1371/journal.pone.0227248 .
Article CAS PubMed PubMed Central Google Scholar
Silvetti S, Ranucci M, Pistuddi V, Isgro G, Ballotta A, Ferraris L, et al. Bloodstream infections during post-cardiotomy extracorporeal membrane oxygenation: incidence, risk factors, and outcomes. Int J Artif Organs. 2019;42(6):299–306. https://doi.org/10.1177/0391398818817325 .
Article CAS PubMed Google Scholar
Hsu MS, Chiu KM, Huang YT, Kao KL, Chu SH, Liao CH. Risk factors for nosocomial infection during extracorporeal membrane oxygenation. J Hosp Infect. 2009;73(3):210–6. https://doi.org/10.1016/j.jhin.2009.07.016 .
Sun HY, Ko WJ, Tsai PR, Sun CC, Chang YY, Lee CW, et al. Infections occurring during extracorporeal membrane oxygenation use in adult patients. J Thorac Cardiovasc Surg. 2010;140(5):1125-1132.e1122. https://doi.org/10.1016/j.jtcvs.2010.07.017 .
O’Neill JM, Schutze GE, Heulitt MJ, Simpson PM, Taylor BJ. Nosocomial infections during extracorporeal membrane oxygenation. Intensive Care Med. 2001;27(8):1247–53. https://doi.org/10.1007/s001340101029 .
Schmidt M, Brechot N, Hariri S, Guiguet M, Luyt CE, Makri R, et al. Nosocomial infections in adult cardiogenic shock patients supported by venoarterial extracorporeal membrane oxygenation. Clin Infect Dis. 2012;55(12):1633–41. https://doi.org/10.1093/cid/cis783 .
Grasselli G, Scaravilli V, Di Bella S, Biffi S, Bombino M, Patroniti N, et al. Nosocomial infections during extracorporeal membrane oxygenation: incidence, etiology, and impact on patients’ outcome. Crit Care Med. 2017;45(10):1726–33. https://doi.org/10.1097/CCM.0000000000002652 .
Biffi S, Di Bella S, Scaravilli V, Peri AM, Grasselli G, Alagna L, et al. Infections during extracorporeal membrane oxygenation: epidemiology, risk factors, pathogenesis and prevention. Int J Antimicrob Agents. 2017;50(1):9–16. https://doi.org/10.1016/j.ijantimicag.2017.02.025 .
Page MJ, McKenzie JE, Bossuyt PM, Boutron I, Hoffmann TC, Mulrow CD, et al. The PRISMA 2020 statement: an updated guideline for reporting systematic reviews. BMJ. 2021;372: n71. https://doi.org/10.1136/bmj.n71 .
Cumpston MS, McKenzie JE, Thomas J, Brennan SE. The use of ‘PICO for synthesis’ and methods for synthesis without meta-analysis: protocol for a survey of current practice in systematic reviews of health interventions. F1000Research. 2020. https://doi.org/10.12688/f1000research.24469.2 .
Morgan RL, Thayer KA, Santesso N, Holloway AC, Blain R, Eftim SE, et al. Evaluation of the risk of bias in non-randomized studies of interventions (ROBINS-I) and the 'target experiment’ concept in studies of exposures: rationale and preliminary instrument development. Environ Int. 2018;120:382–7. https://doi.org/10.1016/j.envint.2018.08.018 .
Higgins JP, Altman DG, Gøtzsche PC, Jüni P, Moher D, Oxman AD, et al. The cochrane collaboration’s tool for assessing risk of bias in randomised trials. BMJ. 2011;343: d5928. https://doi.org/10.1136/bmj.d5928 .
Wang WW, Zhou QX, Ma L, Feng SH, Yang ZR, Sun F, et al. Introduction of a tool to assess risk of bias in non-randomized studies-of environmental exposure (ROBINS-E). Zhonghua Liu Xing Bing Xue Za Zhi. 2022;43(1):98–104. https://doi.org/10.3760/cma.j.cn112338-20201112-01324 .
Garner JS, Jarvis WR, Emori TG, Horan TC, Hughes JM. CDC definitions for nosocomial infections, 1988. Am J Infect Control. 1988;16(3):128–40. https://doi.org/10.1016/0196-6553(88)90053-3 .
Centers for Disease Control and Prevention (CDC). CDC/NHSN surveillance definitions for specific types of infections. 2018; (17): 1–30.
Mornese Pinna S, Sousa Casasnovas I, Olmedo M, Machado M, Juàrez Fernández M, Devesa-Cordero C, et al. Nosocomial infections in adult patients supported by extracorporeal membrane oxygenation in a cardiac intensive care unit. Microorganisms. 2023. https://doi.org/10.3390/microorganisms11041079 .
Haque M, Sartelli M, McKimm J, Abu BM. Health care-associated infections—an overview. Infect Drug Resist. 2018;11:2321–33. https://doi.org/10.2147/idr.S177247 .
Control CfD, Prevention. Pneumonia (ventilator-associated [VAP] and non-ventilator-associated pneumonia [PNEU]) event. Device-associated Module PNEU/VAP. 2015.
Cumpston MS, McKenzie JE, Welch VA, Brennan SE. Strengthening systematic reviews in public health: guidance in the cochrane handbook for systematic reviews of interventions. J Public Health. 2022. https://doi.org/10.1093/pubmed/fdac036 .
Article Google Scholar
Wang D, Mou ZY, Zhai JX, Zong HX, Zhao XD. Application of Stata software to test heterogeneity in meta-analysis method. Zhonghua Liu Xing Bing Xue Za Zhi. 2008;29(7):726–9.
CAS PubMed Google Scholar
Egger M, Davey Smith G, Schneider M, Minder C. Bias in meta-analysis detected by a simple, graphical test. BMJ. 1997;315(7109):629–34. https://doi.org/10.1136/bmj.315.7109.629 .
Begg CB, Mazumdar M. Operating characteristics of a rank correlation test for publication bias. Biometrics. 1994;50(4):1088–101.
Duval S, Tweedie R. Trim and fill: a simple funnel-plot-based method of testing and adjusting for publication bias in meta-analysis. Biometrics. 2000;56(2):455–63. https://doi.org/10.1111/j.0006-341x.2000.00455.x .
Pieri M, Agracheva N, Fumagalli L, Greco T, De Bonis M, Calabrese MC, et al. Infections occurring in adult patients receiving mechanical circulatory support: the two-year experience of an Italian national referral tertiary care center. Med Intensiva. 2013;37(7):468–75. https://doi.org/10.1016/j.medin.2012.08.009 .
Kim DW, Yeo HJ, Yoon SH, Lee SE, Lee SJ, Cho WH, et al. Impact of bloodstream infections on catheter colonization during extracorporeal membrane oxygenation. J Artif Organs. 2016;19(2):128–33. https://doi.org/10.1007/s10047-015-0882-5 .
Kim GS, Lee KS, Park CK, Kang SK, Kim DW, Oh SG, et al. Nosocomial infection in adult patients undergoing veno-arterial extracorporeal membrane oxygenation. J Korean Med Sci. 2017;32(4):593–8. https://doi.org/10.3346/jkms.2017.32.4.593 .
Kutleša M, Santini M, Krajinović V, Papić N, Novokmet A, Josipović Mraović R, et al. Nosocomial blood stream infections in patients treated with venovenous extracorporeal membrane oxygenation for acute respiratory distress syndrome. Minerva Anestesiol. 2017;83(5):493–501. https://doi.org/10.23736/S0375-9393.17.11659-7 .
Sun G, Li B, Lan H, Wang J, Lu L, Feng X, et al. Risk factors for nosocomial infections in patients receiving extracorporeal membrane oxygenation supportive therapy. Med Clin. 2017;149(10):423–8. https://doi.org/10.1016/j.medcli.2017.03.038 .
Menaker J, Galvagno S, Rabinowitz R, Penchev V, Hollis A, Kon Z, et al. Epidemiology of blood stream infection in adult extracorporeal membrane oxygenation patients: a cohort study. Heart Lung. 2019;48(3):236–9. https://doi.org/10.1016/j.hrtlng.2019.01.004 .
Ko RE, Huh K, Kim DH, Na SJ, Chung CR, Cho YH, et al. Nosocomial infections in in-hospital cardiac arrest patients who undergo extracorporeal cardiopulmonary resuscitation. PLoS ONE. 2020. https://doi.org/10.1371/journal.pone.0243838 .
Wang J, Huang J, Hu W, Cai X, Hu W, Zhu Y. Risk factors and prognosis of nosocomial pneumonia in patients undergoing extracorporeal membrane oxygenation: a retrospective study. J Int Med Res. 2020. https://doi.org/10.1177/0300060520964701 .
Wang J, Wang L, Jia M, Du Z, Hou X. Extracorporeal membrane oxygenation-related nosocomial infection after cardiac surgery in adult patients. Braz J Cardiovasc Surg. 2021;36(6):743–51. https://doi.org/10.21470/1678-9741-2020-0068 .
Quintana MT, Mazzeffi M, Galvagno SM, Herrera D, Boyajian GP, Hays NM, et al. A retrospective study of infection in patients requiring extracorporeal membrane oxygenation support. Ann Thorac Surg. 2021;112(4):1168–75. https://doi.org/10.1016/j.athoracsur.2020.12.012 .
Selçuk ÜN, Sargın M, Baştopçu M, Mete EMT, Erdoğan SB, Öcalmaz Ş, et al. Microbiological spectrum of nosocomial ECMO infections in a tertiary care center. Braz J Cardiovasc Surg. 2021;36(3):338–45. https://doi.org/10.21470/1678-9741-2020-0077 .
Lee EH, Lee KH, Lee SJ, Kim J, Baek YJ, Ahn JY, et al. Clinical and microbiological characteristics of and risk factors for bloodstream infections among patients with extracorporeal membrane oxygenation: a single-center retrospective cohort study. Sci Rep. 2022. https://doi.org/10.1038/s41598-022-19405-z .
Manerikar A, Watanabe S, Kandula V, Karim A, Thakkar S, Saine M, et al. Indwelling central venous catheters drive bloodstream infection during veno-venous extracorporeal membrane oxygenation support. ASAIO J. 2022;68(6):859–64. https://doi.org/10.1097/MAT.0000000000001575 .
Xu W, Fu Y, Yao Y, Zhou J, Zhou H. Nosocomial infections in nonsurgical patients undergoing extracorporeal membrane oxygenation: a retrospective analysis in a Chinese hospital. Infect Drug Resist. 2022;15:4117–26. https://doi.org/10.2147/IDR.S372913 .
Zang F, Zhang X, Liu J, Li S, Zhang Y, Li Z. Hospital expenses of nosocomial infection associated with extracorporeal membrane oxygenation in China: a retrospective cohort study. Ann Palliat Med. 2022;11(2):431–41. https://doi.org/10.21037/apm-21-1825 .
Austin DE, Kerr SJ, Al-Soufi S, Connellan M, Spratt P, Goeman E, et al. Nosocomial infections acquired by patients treated with extracorporeal membrane oxygenation. Crit Care Resusc. 2017;19:68–75.
Li B, Sun G, Cheng Z, Mei C, Liao X, Li J, et al. Analysis of nosocomial infections in post-cardiac surgery extracorporeal membrane oxygenation support therapy. Heart Surg Forum. 2018;21(5):E387–91. https://doi.org/10.1532/hsf.1789 .
Bougle A, Bombled C, Margetis D, Lebreton G, Vidal C, Coroir M, et al. Ventilator-associated pneumonia in patients assisted by veno-arterial extracorporeal membrane oxygenation support: epidemiology and risk factors of treatment failure. PLoS ONE. 2018. https://doi.org/10.1371/journal.pone.0194976 .
Juthani BK, Macfarlan J, Wu J, Misselbeck TS. Incidence of nosocomial infections in adult patients undergoing extracorporeal membrane oxygenation. Heart Lung. 2018;47(6):626–30. https://doi.org/10.1016/j.hrtlng.2018.07.004 .
Kim DW, Cho HJ, Kim GS, Song SY, Na KJ, Oh SG, et al. Predictive value of procalcitonin for infection and survival in adult cardiogenic shock patients treated with extracorporeal membrane oxygenation. Chonnam Med J. 2018;54(1):48–54. https://doi.org/10.4068/cmj.2018.54.1.48 .
Na SJ, Chung CR, Choi HJ, Cho YH, Yang JH, Suh GY, et al. Blood stream infection in patients on venovenous extracorporeal membrane oxygenation for respiratory failure. Infect Control Hosp Epidemiol. 2018;39(7):871–4. https://doi.org/10.1017/ice.2018.90 .
Solla-Buceta M, Gonzalez-Vilchez F, Almenar-Bonet L, Lambert-Rodriguez JL, Segovia-Cubero J, Gonzalez-Costello J, et al. Infectious complications associated with short-term mechanical circulatory support in urgent heart transplant candidates. Rev Esp Cardiol. 2022;75(2):141–9. https://doi.org/10.1016/j.rec.2020.11.019 .
Seidelman JL, Lewis SS, Huslage K, Strittholt N, Vereen S, Sova C, et al. To be a CLABSI or not to be a CLABSI-that is the question: the epidemiology of BSI in a large ECMO population. Infect Control Hosp Epidemiol. 2018;39(3):362–5. https://doi.org/10.1017/ice.2017.320 .
Fu KX, MacLaren G. Infectious complications of postcardiotomy extracorporeal membrane oxygenation. Pediatr Critic Care Med. 2020;21(11):1019–20. https://doi.org/10.1097/pcc.0000000000002447 .
Bizzarro MJ, Conrad SA, Kaufman DA, Rycus P. Infections acquired during extracorporeal membrane oxygenation in neonates, children, and adults. Pediatr Critic Care Med. 2011;12(3):277–81. https://doi.org/10.1097/PCC.0b013e3181e28894 .
Vogel AM, Lew DF, Kao LS, Lally KP. Defining risk for infectious complications on extracorporeal life support. J Pediatr Surg. 2011;46(12):2260–4. https://doi.org/10.1016/j.jpedsurg.2011.09.013 .
Peña-López Y, Machado MC, Rello J. Infection in ECMO patients: changes in epidemiology, diagnosis and prevention. Anaesth Critic Care Pain Med. 2024;43(1): 101319. https://doi.org/10.1016/j.accpm.2023.101319 .
Peitz GJ, Murry DJ. The influence of extracorporeal membrane oxygenation on antibiotic pharmacokinetics. Antibiotics. 2023;12(3):500.
Maclaren G, Butt W. Extracorporeal membrane oxygenation and sepsis. Crit Care Resusc. 2007;9(1):76–80.
MacLaren G, Schlapbach LJ, Aiken AM. Nosocomial infections during extracorporeal membrane oxygenation in neonatal, pediatric, and adult patients: a comprehensive narrative review. Pediatr Critic Care Med J Soc Critic Care Med World Federation Pediatr Intensiv Critic Care Soc. 2020;21(3):283–90. https://doi.org/10.1097/pcc.0000000000002190 .
Download references
Acknowledgements
Thanks to guidance and advice from the “Clinical Research Development Unit" of Baqiyatallah Hospital.
This research did not receive any specific grant from funding agencies in the public, commercial, or not-for-profit sectors.
Author information
Authors and affiliations.
Medical Intensive Care Unit, Hamad General Hospital, Doha, Qatar
Ali Ait Hssain, Abdulsalam Saif Ibrahim & Ibrahim Fawzy Hassan
Department of Medicine, Weill Cornell Medical College, Doha, Qatar
College of Health and Life Science, Hamad Bin Khalifa University, Doha, Qatar
Ali Ait Hssain
Trauma Research Center, Nursing Faculty, Baqiyatallah University of Medical Sciences, Sheykh Bahayi Street, Vanak Square, P.O. Box 19575-174, Tehran, Iran
Amir Vahedian-Azimi
Médecine Intensive et Réanimation, Hôpital Saint-Louis, Assistance Publique-Hôpitaux de Paris, University of Paris, Paris, France
Elie Azoulay & Michael Darmon
You can also search for this author in PubMed Google Scholar
Contributions
A.AH, A.VA and M.D contributed to the conception and design of the study. A.AH, A.VA and A.SI performed the title and abstract screening. A.SI, I.FH and E.A performed the data extraction. A.SI and M.D organized the data and created the characteristic tables. A.VA and A.AH performed the data analysis. A.AH, I.FH and A.SI wrote the first draft of the manuscript. All authors contributed to manuscript revision, read, and approved the submitted version.
Corresponding author
Correspondence to Amir Vahedian-Azimi .
Ethics declarations
Ethics approval and consent to participate.
The predefined protocol was registered in the International Prospective Register of Systematic Reviews (PROSPERO) database (CRD42023372412).
Consent for publication
Not applicable.
Competing interests
The authors have no conflicts of interest to disclose related to this study.
Additional information
Publisher's note.
Springer Nature remains neutral with regard to jurisdictional claims in published maps and institutional affiliations.
Supplementary Information
Additional file 1 (docx 793 kb), additional file 2 (xlsx 72 kb), additional file 3 (docx 217 kb), additional file 4 (docx 2245 kb), rights and permissions.
Open Access This article is licensed under a Creative Commons Attribution 4.0 International License, which permits use, sharing, adaptation, distribution and reproduction in any medium or format, as long as you give appropriate credit to the original author(s) and the source, provide a link to the Creative Commons licence, and indicate if changes were made. The images or other third party material in this article are included in the article's Creative Commons licence, unless indicated otherwise in a credit line to the material. If material is not included in the article's Creative Commons licence and your intended use is not permitted by statutory regulation or exceeds the permitted use, you will need to obtain permission directly from the copyright holder. To view a copy of this licence, visit http://creativecommons.org/licenses/by/4.0/ . The Creative Commons Public Domain Dedication waiver ( http://creativecommons.org/publicdomain/zero/1.0/ ) applies to the data made available in this article, unless otherwise stated in a credit line to the data.
Reprints and permissions
About this article
Cite this article.
Ait Hssain, A., Vahedian-Azimi, A., Ibrahim, A.S. et al. Incidence, risk factors and outcomes of nosocomial infection in adult patients supported by extracorporeal membrane oxygenation: a systematic review and meta-analysis. Crit Care 28 , 158 (2024). https://doi.org/10.1186/s13054-024-04946-8
Download citation
Received : 26 March 2024
Accepted : 08 May 2024
Published : 10 May 2024
DOI : https://doi.org/10.1186/s13054-024-04946-8
Share this article
Anyone you share the following link with will be able to read this content:
Sorry, a shareable link is not currently available for this article.
Provided by the Springer Nature SharedIt content-sharing initiative
- Meta-analysis
- Nosocomial infection
- Risk factors
Critical Care
ISSN: 1364-8535
- Submission enquiries: [email protected]
- Open access
- Published: 18 February 2020
A proposal for a comprehensive approach to infections across the surgical pathway
- Massimo Sartelli ORCID: orcid.org/0000-0003-3202-7542 1 ,
- Leonardo Pagani 2 ,
- Stefania Iannazzo 3 ,
- Maria Luisa Moro 4 ,
- Pierluigi Viale 5 ,
- Angelo Pan 6 ,
- Luca Ansaloni 7 ,
- Federico Coccolini 8 ,
- Marcello Mario D’Errico 9 ,
- Iris Agreiter 10 ,
- Giorgio Amadio Nespola 11 ,
- Francesco Barchiesi 12 ,
- Valeria Benigni 13 ,
- Raffaella Binazzi 2 ,
- Stefano Cappanera 14 ,
- Alessandro Chiodera 15 ,
- Valentina Cola 16 ,
- Daniela Corsi 17 ,
- Francesco Cortese 18 ,
- Massimo Crapis 19 ,
- Francesco Cristini 20 ,
- Alessandro D’Arpino 21 ,
- Belinda De Simone 22 ,
- Stefano Di Bella 23 ,
- Francesco Di Marzo 24 ,
- Abele Donati 25 ,
- Daniele Elisei 26 ,
- Massimo Fantoni 27 ,
- Anna Ferrari 28 ,
- Domitilla Foghetti 29 ,
- Daniela Francisci 30 ,
- Gianni Gattuso 31 ,
- Andrea Giacometti 32 ,
- Guido Cesare Gesuelli 1 ,
- Cristina Marmorale 33 ,
- Enrica Martini 34 ,
- Marcello Meledandri 35 ,
- Rita Murri 27 ,
- Daniela Padrini 36 ,
- Dalia Palmieri 37 ,
- Paola Pauri 38 ,
- Carla Rebagliati 39 ,
- Enrico Ricchizzi 4 ,
- Vittorio Sambri 40 , 41 ,
- Anna Maria Schimizzi 42 ,
- Walter Siquini 1 ,
- Loredana Scoccia 43 ,
- Giancarlo Scoppettuolo 44 ,
- Gabriele Sganga 45 ,
- Nadia Storti 46 ,
- Marcello Tavio 47 ,
- Giulio Toccafondi 48 ,
- Fabio Tumietto 5 ,
- Bruno Viaggi 49 ,
- Marco Vivarelli 50 ,
- Cristian Tranà 1 ,
- Melina Raso 51 ,
- Francesco Maria Labricciosa 52 ,
- Sameer Dhingra 53 &
- Fausto Catena 54
World Journal of Emergency Surgery volume 15 , Article number: 13 ( 2020 ) Cite this article
12k Accesses
17 Citations
5 Altmetric
Metrics details
Despite evidence supporting the effectiveness of best practices in infection prevention and management, many healthcare workers fail to implement them and evidence-based practices tend to be underused in routine practice. Prevention and management of infections across the surgical pathway should always focus on collaboration among all healthcare workers sharing knowledge of best practices. To clarify key issues in the prevention and management of infections across the surgical pathway, a multidisciplinary task force of experts convened in Ancona, Italy, on May 31, 2019, for a national meeting. This document represents the executive summary of the final statements approved by the expert panel.
Prevention and management of infections across the surgical pathway should always focus on collaboration among all healthcare professionals with shared knowledge and widespread diffusion of best practices.
Leading international organizations, such as the World Health Organization (WHO), acknowledge that collaborative practice is essential for achieving a concerted approach to providing care that is appropriate to meet the needs of patients, thus optimizing individual health outcomes and overall service delivery of healthcare [ 1 ].
To clarify key issues in the prevention and management of infections across the surgical pathway, a multidisciplinary task force of national experts convened in Ancona, Italy, on May 31, 2019, for a national meeting. The multifaceted nature of these infections has led to a multidisciplinary collaboration involving epidemiologists and infection control specialists, infectious disease specialists, hospital pharmacists, microbiologists, intensivists, general and emergency surgeons, and nurses. During the meeting, the panelists presented the statements developed for each of the main questions regarding the prevention and management of infections in surgery. An agreement on the statements was reached by the Delphi method. Statements were approved with an agreement of ≥ 80%. After the meeting, the expert panel met via email to prepare and revise the consensus paper resulting from the meeting. The manuscript was successively reviewed by all members and ultimately revised as the present manuscript. This document represents the executive summary of the final statements approved by the expert panel.
Healthcare-associated infections and patient safety
Improving patient safety in hospitals worldwide presently requires a systematic approach to preventing healthcare-associated infections (HAIs) and antimicrobial resistance (AMR). The two go together. HAIs are infections that occur while receiving healthcare. Patients with medical devices (central lines, urinary catheters, ventilators) or who undergo surgical procedures are at risk of acquiring HAIs.
The occurrence of HAIs continues to escalate at an alarming rate. These infections result in significant patient illnesses and deaths, prolong the duration of hospital stay, and necessitate additional diagnostic and therapeutic interventions, which generate supplementary costs to those already sustained due to the patient’s underlying disease. However, the phenomenon is not yet sufficiently perceived among both healthcare workers (HCWs) and patients, thus resulting in a low level of intervention request and relative inadequate responses [ 2 ]. Although HAIs are the most frequent adverse events in healthcare, their true global burden remains unknown because of the difficulty in gathering reliable data: most countries lack surveillance systems for HAIs, and those do have them struggle with the complexity and the lack of uniformity of criteria [ 3 ].
HAIs are considered adverse events, and as many are preventable, they are considered an indicator of the quality of patient care and a patient safety issue. In 2018, a systematic review and meta-analysis of studies between 2005 and 2016 evaluated the results of multifaceted interventions to reduce catheter-associated urinary tract infections (CAUTIs), central line-associated bloodstream infections (CLABSIs), surgical site infections (SSIs), ventilator-associated pneumonia, and hospital-acquired pneumonia not associated with mechanical ventilation in acute care or long-term care settings [ 4 ]. Of the 5226 articles identified, 144 studies were included in the final analysis. Published evidence suggested a sustained potential for the significant reduction of HAI rates in the range of 35–55% associated with multifaceted interventions irrespective of a country’s income level.
Question 1. How can you implement global guidelines for the prevention of surgical site infections (SSIs)?
Statement 1.1. Recent global guidelines for the prevention of SSIs can support healthcare workers to develop or strengthen infection prevention and control programs, with a focus on surgical safety, as well as AMR action plans. All healthcare workers should adopt these evidence-based recommendations in their clinical practice.
Statement 1.2. A safer surgical care requires a range of precautions aimed at reducing the risk of SSIs before, during and after surgery.
Statement 1.3. To support local implementation of guidelines for the prevention of SSIs, 5 steps of a multimodal strategy, including system change, training and education, evaluation and feedback, communications for awareness raising and institutional safety climate and culture are suggested.
Improving behavior in infection prevention and control (IPC) practices remains a challenge. Despite progress in preventive knowledge, SSIs remain the most common HAI among surgical patients and one of the most frequent adverse events in hospitals. They represent a major clinical problem in terms of morbidity, mortality, length of hospital stay, and overall direct and not direct costs worldwide. It is obviously important to improve patient safety by reducing the occurrence of SSIs. Preventing SSIs is a global priority, also because bacteria are becoming increasingly resistant to antibiotics, making SSI prevention even more important nowadays. On the other hand, SSI prevention is complex and requires the integration of a range of measures before, during, and after surgery.
Both WHO [ 5 , 6 ] and the Centers for Disease Control and Prevention (CDC) [ 7 ] have published guidelines for the prevention of SSIs. The 2016 WHO Global guidelines for the prevention of SSI [ 5 , 6 ] are evidence-based including systematic reviews presenting additional information in support of actions to improve practice. The first-ever global guidelines for the prevention of SSIs were published on November 3, 2016, then were updated in some parts and published in a new edition in December 2018. The guidelines include 13 recommendations for the preoperative period and 16 for preventing infections during and after surgery. They range from simple precautions such as ensuring that patients bathe or shower before surgery, appropriate way for surgical teams to clean their hands, guidance on when to use prophylactic antibiotics, which disinfectants to use before incision, and which sutures to use.
The proposed recommendations are classified as follows:
“Strong”: Expert panel was confident that benefits outweighed risks, considered to be adaptable for implementation in most (if not all) situations, and patients should receive intervention as a course of action.
“Conditional”: Expert panel considered that benefits of intervention probably outweighed the risks; a more structured decision-making process should be undertaken, based on stakeholder consultation and involvement of patients and healthcare professionals.
In 2018, WHO published a document about the implementation approaches for these evidence-based recommendations [ 8 ]. The purpose of this document is to present a range of tested approaches to achieve successful SSI prevention implementation at the facility level, including in the context of a broader surgical safety climate, using an evidence- and team-based approach and a multimodal strategy for achieving sustainable change based on system change, training and education, evaluation and feedback, communications for awareness raising, and institutional safety climate and culture. The manual is aimed at all those concerned with the prevention of SSIs. A multidisciplinary team is necessary to successfully implement preventive measures. This should include at least IPC and associated staff, such as those working in epidemiology, decontamination/sterilization, quality improvement and patient safety, hospital administration, and the surgical teams (including surgeons, anesthesiologists, and perioperative nurses).
Question 2. Why do you have to survey HAIs?
Statement 2.1. Surveillance of HAIs improves the quality of care because it reduces the risk of infection. It should be supported by all healthcare workers.
IPC program should be in place to prevent HAIs in all hospitals worldwide, and one of the main cornerstones is the presence of a formal system to monitor IPC and ensure that appropriate actions are taken to minimize infection rates [ 9 ]. HAI surveillance is a challenging task also because it requires particular expertise after obtaining epidemiological data to assess the quality of the information produced and to interpret its meaning and root cause in order to tailor intervention and prevention measures.
Program surveying SSIs have been implemented throughout the world and are associated with a reduction in SSI rates. Data on non-prosthetic surgery from the Italian SSI surveillance program for the period 2009 to 2011 [ 10 ] demonstrated that implementation of a national surveillance program was helpful in reducing SSI rates and should be prioritized in all healthcare systems. A 17% decrease in SSI related to ten selected procedures was reported between 2008 and 2013 in the USA following improvement programs [ 11 ]. In African hospitals, a 60% SSI risk reduction was observed following the implementation of a WHO multimodal strategy in the context of the WHO Surgical Unit-based Safety Program (SUSP) including SSI surveillance [ 12 ]. Surveillance also allows hospitals and clinicians to measure the effectiveness of strategies that are implemented to decrease infection rates. Infection rate data should be used in a positive way to improve the quality and safety of healthcare.
HAI surveillance is conventionally conducted by two methods. Passive surveillance (self-reporting of suspected HAIs by the treating physicians) is a very poor and inefficient method to track HAIs as there is a risk of bias and underreporting. Active surveillance, on the other hand, is the systematic collection of data by a designated unbiased surveillance team. This is the method recommended by the main surveillance networks. Following the data extraction, analysis of the collected information should be done. Feedback and reports after the analysis should be disseminated by infection control committees, keeping the confidentiality of individuals. The importance of surveillance systems for HAI control has been accepted globally, and some countries have established national surveillance systems with the aim to prevent HAIs.
Question 3. How can you implement the prevention of HAIs?
Statement 3.1. It is necessary to set up a solid and branched surveillance network gathering alert signals, verifying their severity and initiating the organizational response via “warnings”.
Statement 3.2. The collection and analysis of monitoring data serve to identify vulnerabilities in the system. This is the basis for organizational improvement, risk reduction, and damage control.
HAIs affect around 5–15% of all hospital patients worldwide. Despite the availability of standard procedures and evidence-based guidelines aiming at reducing the impact of HAIs, the implementation of those into routine practice appears as the biggest challenge [ 13 ].
HAI surveillance and timely feedback of results are strongly recommended by WHO as part of the core components of effective IPC programs [ 14 ]. Every healthcare facility should be committed to provide quality and safe care. Surveillance is not to be undertaken in isolation, but as integrated into a comprehensive and multimodal IPC strategy. Conducting high-quality IPC and surveillance is crucial to assess the safety level of the surgical workflow, detect criticalities, and diffuse warnings to trigger the response capability of healthcare organizations. Feedback on IPC achievements should be constantly monitored and timely disseminated throughout the levels of the organization by the hospital IPC [ 15 ]. Surveillance of HAIs is a fundamental aspect of the IPC program, in particular, when SSIs are identified as a target for improvement.
Particularly in surgical care, SSI surveillance provides feedback to surgical teams on the HAI risks patients are exposed to. Cooperation of surgical teams in surveillance efforts is crucial to make visible to them the effect on patients’ care, if they have confidence in the methods being used. Thus, it is important for surgeons to comprehend the opportunities of the surveillance process for surgical care improvements [ 15 ]. In this regard, the support of human factors and ergonomics paired with implementation science is crucial to embed the knowledge gained through an epidemiological into the daily routine of HCWs [ 16 ].
Question 4. How can you prevent and manage Clostridioides difficile infection (CDI)?
Statement 4.1. Key points for CDI prevention are:
Antimicrobial stewardship.
Contact precautions.
Hand washing (soap, not alcohol).
Avoid unnecessary gastric acid suppressants.
Statement 4.2. Key points for CDI treatment are:
Stop unnecessary antibiotics.
Metronidazole (mild episodes).
Oral/intracolonic vancomycin.
Oral fidaxomicin.
IV bezlotoxumab (recurrent episodes).
Fecal microbiota transplantation.
Prompt surgery when indicated.
In the last two decades, CDI has become a major global public health problem, with a dramatic increase in the incidence and severity of episodes. CDI may be a particular concern in surgical patients, as surgery may predispose patients to CDI and surgery itself could be necessary to treat severe cases of CDI [ 17 ].
Risk factors for CDI may be divided into three general categories [ 17 ]:
Host factors (immune status, co-morbidities)
Exposure to C. difficile spores (hospitalizations, community sources, long-term care facilities)
Factors that disrupt normal colonic microbiome (antibiotics, other medications, surgery)
The main risk factors are antibiotic exposure, age more than 65 years, comorbidity or underlying conditions, inflammatory bowel diseases, immunodeficiency (including human immunodeficiency virus infection), malnutrition, and low serum albumin level. Antibiotics play a central role in the pathogenesis of CDI, presumably by disrupting the normal gut flora, thereby providing a perfect setting for C. difficile to proliferate and produce toxins. Although nearly all antibiotics have been associated with CDI, clindamycin, third-generation cephalosporins, penicillins, and fluoroquinolones have usually been considered at greatest risk [ 16 ].
A prompt and precise diagnosis is an important aspect of effective management of CDI. Early identification of CDI allows the establishment of an early treatment and can improve outcomes. Rapid isolation of infected patients is fundamental to limit C. difficile transmission. This is particularly important in reducing environmental contamination as spores can survive for months in the environment, despite regular use of environmental cleaning agents. Patients with CDI should be maintained in contact (enteric) precautions until the resolution of diarrhea (passage of formed stool for at least 48 h). Patients with known or suspected CDI should ideally be placed in a private room with en suite hand washing and toilet facilities. If a private room is not available, as often occurs, known CDI patients may be cohort, nursed in the same area, though the theoretical risk of transfection with different strains exists. Hand hygiene with soap and water and the use of contact precautions along with a good cleaning and disinfection of the environment and patient equipment should be used by all HCWs contacting any patient with known or suspected CDI. Alcohol-based hand sanitizers are highly effective against non-spore-forming organisms, but they may not kill C. difficile spores or remove C. difficile from the hands. The most effective way to remove them from the hands is through handwashing with soap and water.
In cases of suspected severe CDI, antibiotic agents should be discontinued, if possible [ 18 ]. A meta-analysis addressing factors associated with prolonged symptoms and severe disease due to C. difficile showed that continued use of antibiotics for infections other than CDI is significantly associated with an increased risk of CDI recurrence [ 18 ]. If continued antibiotic therapy is required for the treatment of the primary infection, antimicrobial therapy with agents that are less frequently implicated with antibiotic-associated CDI should be used; these include parenteral aminoglycosides, sulfonamides, macrolides, vancomycin, or tetracycline/tigecycline.
Although there is a clinical association between proton pump inhibitor (PPI) use and CDI [ 19 ], no randomized controlled trial (RCT) studies have studied the relationship between discontinuing or avoiding PPI use and the risk of CDI. Thus, a strong recommendation to discontinue PPIs in patients at high risk for CDI regardless of the need for PPIs will require further evidence. However, stewardship activities to discontinue unneeded PPIs are strongly warranted.
Regarding treatment, antibiotic therapy is the first choice for CDI treatment and molecule choice should be based according to the severity of the disease. When antibiotic therapy is indicated for symptomatic cases with a positive stool C. difficile test, options include metronidazole, oral or intraluminal vancomycin, and oral fidaxomicin [ 20 , 21 , 22 , 23 , 24 ]. Metronidazole should be limited to the treatment of an initial episode of mild-moderate CDI. Vancomycin orally 125 mg four times daily for 10 days is considered superior to metronidazole in severe CDI [ 25 , 26 , 27 ]. Doses of up to 500 mg have been used in patients with severe or fulminant, as defined as hypotension or shock, ileus, or megacolon, CDI [ 28 ], although there is little evidence for this in the literature. Fidaxomicin orally 200 mg twice daily for 10 days may be a valid alternative to vancomycin in patients with CDI [ 29 , 30 ]. Fidaxomicin may be useful for treating patients who are considered at high risk for recurrence (elderly patients with multiple comorbidities who are receiving concomitant antibiotics).
Fecal microbiota transplantation (FMT) is an effective option for patients with multiple CDI recurrences who have failed appropriate antibiotic treatments [ 31 ]. FMT involves infusing intestinal microorganisms (in a suspension of healthy donor stool) into the intestine of patients to restore the intestinal microbiota. The rationale of FMT is that disruption of the normal balance of colonic flora allows C. difficile strains to grow and produce CDI. By reintroducing normal flora via donor feces, the imbalance may be corrected and normal bowel function re-established [ 31 ].
Coadjuvant treatment with monoclonal antibodies (bezlotoxumab) may prevent recurrences of CDI, particularly in patients with CDI due to the 027 epidemic strain, in immunocompromised patients and in patients with severe CDI. Bezlotoxumab (MK-6072), approved in 2016 by Food and Drug Administration (FDA), is a human monoclonal antibody which reduces recurrent CDI by blocking the binding of C. difficile toxin B to host cells, thus limiting epithelial damage and facilitating recovery of the microbiome [ 32 ].
Patients with severe CDI who progress to systemic toxicity should undergo early surgical consultation and should be evaluated for potential surgical intervention. Resection of the entire colon should be considered to treat patients with fulminant colitis. However, diverting loop ileostomy with colonic lavage is a useful alternative to resection of the entire colon.
Question 5. How can you prevent central-venous catheter-related infections?
Statement 5.1. The most effective means to reduce to the minimum possible central-venous catheter-related infections are represented by a «bundles» management, based on the guidelines, implemented with training and motivational meetings aimed at increasing compliance of healthcare workers (better if organized in a dedicated team) and applied by checklist.
In order to guarantee a correct management of central venous catheter-related infections, a correct diagnostic framework is essential, to be obtained by a standardized execution of blood cultures from a peripheral vein and central venous catheter (CVC), in order to be able to implement a correct interpretation of the results and take timely decisions on a possible removal/conservative strategy towards the catheter.
About half of nosocomial bloodstream infections occur in intensive care units (ICUs), and the majority of them are associated with an intravascular device. Central venous catheter-related bloodstream infections (CRBSIs) are an important cause of HAIs. CVCs are integral to modern clinical practices and are inserted in critically ill patients for the administration of fluids, blood products, medication, and nutritional solutions and for hemodynamic monitoring. They are the main source of bacteremia in hospitalized patients and therefore should be used only if necessary.
Risk factors for CRBSIs include patient-, catheter-, and operator-related factors. Several factors have been proposed to participate in the pathogenesis of CRBSI. Hospitalized patients with neutropenia are at higher risk. However, other host risk factors also include immune deficiencies in general, chronic illness, and malnutrition. The diagnosis of CRBSI is often suspected clinically in a patient using a CVC who presents with fever or chills, unexplained hypotension, and no other localizing sign. Diagnosis of CRBSI requires establishing the presence of bloodstream infection and demonstrating that the infection is related to the catheter. However, blood cultures should not be drawn solely from the catheter port as these are frequently colonized with skin contaminants, thereby increasing the likelihood of a false-positive blood culture. Indeed, according to IDSA guidelines [ 33 ], a definitive diagnosis of CRBSI requires a culture of the same organism from both the catheter tip and at least one percutaneous blood culture. Alternatively, the culture of the same organism from at least two blood samples (one from a catheter hub and the other from a peripheral vein or second lumen) meeting criteria for quantitative blood cultures or differential time to positivity. Most laboratories do not perform quantitative blood cultures, but many laboratories are able to determine the differential time to positivity. Quantitative blood cultures demonstrating a colony count from the catheter hub sample ≥ 3-fold higher than the colony count from the peripheral vein sample (or a second lumen) supports a diagnosis of CRBSI. Differential time to positivity refers to growth detected from the catheter hub sample at least 2 h before growth detected from the peripheral vein sample. The CVC and arterial catheter, if present, should be cultured and removed as soon as possible if the patient has unexplained sepsis or erythema overlying the catheter insertion site or purulence at the catheter insertion site in immunocompromised patients.
Antibiotic therapy for catheter-related infection is often initiated empirically. The initial choice of antibiotics will depend on the severity of the patient’s clinical disease, the risk factors for infection, and the likely pathogens associated with the specific intravascular device. Resistance to antibiotic therapy due to biofilm formation also has an important role in the management of bacteremia. In fact, the nature of the biofilm structure makes microorganisms difficult to eradicate and confer an inherent resistance to antibiotics.
CRBSIs can be reduced by a range of interventions including closed infusion systems, aseptic technique during insertion and management of the central venous line, early removal of central venous lines, and appropriate site selection. Different measures have been implemented to reduce the risk for CRBSI, including the use of maximal barrier, precautions during catheter insertion, effective cutaneous anti-sepsis, and preventive strategies based on inhibiting microorganisms originating from the skin or catheter hub from adhering to the catheter [ 34 ]. The simultaneous application of multiple recommended best practices to manage CVCs has been associated with significant declines in the rates of CRBSI. Bundles can be defined as the systematic implementation of a set of evidence-based practices, usually three to five, that when performed properly and collectively can improve patient outcomes. Research on CRBSI prevention demonstrated the effectiveness of bundles, which reduce the incidence of CRBSI by up to 80% [ 35 , 36 , 37 ], reaching a rate of 0 in some cases [ 38 ]. Education and training of healthcare workers and adherence to standardized protocols for insertion and maintenance of intravascular catheters significantly reduced the incidence of catheter-related infections and represent the most important preventive measures.
The global burden of antimicrobial resistance
AMR has emerged as one of the principal public health problems of the twenty-first century. This has resulted in a public health crisis of international concern. Combating resistance has become a top priority for global policymakers and public health authorities. New mechanisms of resistance continue to emerge and spread globally, challenging our ability to manage common infections. Antibacterial and antifungal use in animal and agricultural industries aggravates selective pressure on microbes. A One Health approach is required urgently. Addressing the rising threat of AMR requires a holistic and multisectoral approach—referred to as One Health—because antimicrobials used to treat various infectious diseases in animals may be the same or similar to those used for humans. Resistant bacteria arising in humans, animals, or the environment may spread from one to another and from one country to another. AMR does not recognize geographic or human-animal borders [ 39 ].
The worldwide impact of AMR is significant, in terms of economic and patient outcomes, because of untreatable infections or those necessitating antibiotic agents of last resort leading to increased length of hospital stay, morbidity, death, and treatment cost. Raising awareness of AMR and promoting behavioral change through public communication programs that target different audiences in human health, animal health, and agricultural practice, as well as consumers, are critical to tackling this issue.
HCWs play a central role in preventing the emergence and spread of resistance. An effective and cost-effective strategy to reduce AMR should involve a multifaceted approach aimed at optimizing antibiotic use, strengthening surveillance and IPC, and improving patient and clinician education regarding the appropriate use of antibiotic agents.
Although the phenomenon of AMR can be attributed to many factors, there is a well-established relationship between antimicrobial prescribing practices and the emergence of antimicrobial-resistant pathogens. However, after they have emerged, resistant pathogens may be transmitted from one individual to another. Every infection prevented is one that needs no treatment. Prevention of infection can be cost-effective and implemented in all settings and sectors, even where resources are limited. A range of factors such as diagnostic uncertainty, fear of clinical failure, time pressure, or organizational contexts can complicate both antibiotic prescribing decisions and preventing measures. Because of cognitive dissonance (recognizing that action is necessary but not implementing it), however, changing behavior is extremely challenging, and awareness of AMR is still low.
Every hospital worldwide should utilize the existing resources to create an effective multidisciplinary team for combating AMR. The best strategies for combating AMR are not definitively established and are likely to vary based on local culture, policy, and routine clinical practice despite several guidelines on the topic.
The Italian situation
In a study published in January 2019 in The Lancet Infectious Diseases, the European Center for Disease Prevention and Control (ECDC) assessed the weight of infections due to multiresistant bacteria in invasive isolates in Europe [ 40 ].
Elaborating the 2015 data contained in the European Antimicrobial Resistance Surveillance Network (EARS-Net) and crossing them with a conversion factor, the authors arrived at the first estimate of the impact of antibiotic resistance on the European population. The authors estimate that infections caused by multiresistant bacteria can cause at least 33,000 deaths each year in Europe (equal to the sum of deaths caused by influenza, AIDS, and tuberculosis) and almost 880.000 cases of disability. Italy and Greece have the most infections from multiresistant bacteria. Although we consider that the Italian population is of a medium-high age, it is noteworthy that about a third of deaths due to antibiotic-resistant bacterial infections in Europe have been in Italy. Not surprisingly, in December 2017, the ECDC published a report on the Italian situation and activities for the prevention and control of antibiotic resistance [ 41 ]. The report summarizes visits and meetings that ECDC experts had in Italy from January 9 to 13, 2017, to discuss and specifically assess the situation in the country regarding antibiotic-resistance prevention and control in our country. Observations of this visit by the ECDC confirm that the antibiotic resistance situation in Italian hospitals will represent a serious threat to public health for the country in the near future.
ECDC experts noted the following:
Little sense of urgency about the current AMR situation from most stakeholders and a tendency by many stakeholders to avoid taking charge of the problem
Lack of institutional support at the national, regional, and local level
Lack of professional leadership at each level
Lack of accountability at each level
Lack of coordination of the activities between and within levels
According to a report by the Organization for Economic Co-operation and Development (OECD) [ 42 ], in Italy, the proportion of antibiotic-resistant infections have grown from 17% in 2005 to 30% in 2015 and will reach 32% in 2030, if antibiotic consumption will continue to follow the same trends. The proportion of antibiotic resistance in Italy is substantially higher than that in the 17% average resistance of OECD countries in 2015.
On November 2, 2017, the Ministry of Health published the national anti-microbial resistance plan (PNCAR) 2017–2020 [ 43 ], which identified strategies and actions to be implemented at different levels: national, regional, and local. The PNCAR is developed according to a One Health approach. The actions set out in the plan, at the level of central, regional, and local institutions, pursue specific objectives:
Improve awareness and education of health professionals, citizens, and stakeholders
Monitor the phenomenon of antibiotic resistance and the use of antibiotics
Improve IPC
Optimize the use of antimicrobials in the field of human and animal health
Increase and support research and innovation.
Appropriate management of infections across the surgical pathway
Antibiotics can be life-saving when treating bacterial infections but are often used inappropriately. Although most clinicians are aware of the problem of AMR, most underestimate this problem in their own hospital. Clinicians should always optimize antimicrobial management to maximize the clinical outcome of the patients and minimize the emergence of AMR. The necessity of formalized systematic approaches to the optimization of antibiotic therapy in the setting of surgical units worldwide, both for elective and emergency admissions, has become increasingly urgent.
Below, we report 11 strategies for a correct antibiotic therapy.
Communication and education.
Updating local epidemiological data stratifying them for specific settings.
Start and choice treatment always using a severity driven approach.
Drafting local algorithms / bundles.
Avoid redundant prescriptions.
Not being impulsive in starting antimicrobial therapy.
Being parsimonious with combination regimens.
Strict collaboration with microbiology laboratory in daily life.
Being aware about PK/PD issues.
Shortening therapy.
Creating a multidisciplinary team for specific setting, syndromes, etc.
Hospital-based programs dedicated to improving antibiotic use, commonly referred to as Antimicrobial stewardship programs (ASPs), can both optimize the treatment of infections and minimize adverse events associated with antibiotic use and AMR [ 44 , 45 ]. Every hospital worldwide should utilize the existing resources to create an effective multidisciplinary team. The preferred means of improving antibiotic stewardship should involve a comprehensive program that incorporates collaboration between various specialties within a healthcare institution including infectious disease specialists, hospital pharmacists, clinical pharmacologists, administrators, epidemiologists, IPC specialists, microbiologists, surgeons, anesthesiologists, intensivists, and underutilized but pivotal stewardship team members, the surgical, anesthetic, and intensive care nurses in our hospitals.
Antimicrobial stewardship policies should be based on both international and national antibiotic guidelines and tailored to local microbiology and resistance patterns. Facility-specific treatment recommendations, based on the guidelines and local formulary options promoted by the antimicrobial stewardship team, can guide clinicians in antibiotic selection and duration of the therapy for the most common indications. Standardizing a shared protocol of antibiotic prophylaxis should represent the first step of any ASP. Since physicians are primarily responsible for the decision to use antibiotics, educating them and changing the attitudes and knowledge that underlie their prescribing behavior are crucial for improving antibiotic prescription. Education is fundamental to every ASP; however, due to cognitive dissonance (recognizing that action is necessary but not implementing it), changing the prescribing behavior is extremely challenging [ 46 ]. Efforts to improve educational programs are thus required, and this should preferably be complemented by active interventions such as prospective audits and feedback to clinicians to stimulate further change [ 47 ]. It is also crucial to incorporate fundamental ASP and IPC principles in under- and post-graduate training at medical faculties to equip young doctors and other healthcare professionals with the required confidence, skills, and expertise in the field of antibiotic management.
Question 6. The clinical microbiology laboratory: which is its role in the control of infections with multidrug-resistant bacteria?
Statement 6.1. The implementation of microbiological diagnostic activities improves the diagnostic capacity towards infections caused by multidrug-resistant organisms (MDROs).
Statement 6.2. The three main challenges of a modern microbiology laboratory are: to maintain high-quality services, to consolidate laboratory medical care into large hospital systems and, as a consequence of consolidation to reach a full automation possibly in all the analytical steps of the diagnosis.
ASPs and prescribing physicians depend on the information and guidance from the clinical microbiology laboratory, thus making the laboratory vital to patient care and the success of ASPs. The three most relevant challenges of a modern microbiology laboratory are the following.
Maintaining high-quality and cost-effective services
ASPs aid physicians in providing optimal antimicrobial therapy to their patients, prescribing the right antimicrobial regimen to the right patient for the right period of time, and avoiding the unnecessary use of antimicrobial. Ultimately, ASPs aim to improve patient outcomes while limiting adverse drug events and reducing AMR. The clinical microbiology laboratory plays a critical role in the success of the antimicrobial stewardship efforts by providing essential information for accurately diagnosing and treating patients with infectious diseases [ 48 ].
Clinical microbiology laboratories (CMLs) conduct surveillance on the local AMR trends among microbial pathogens. The collection, organization, and communication of resistance data culminates could be summarized in the preparation of the antibiogram. Antibiograms provide critical information to ASPs and to prescribing physicians on local institution susceptibility patterns. CMLs provide patient-specific information by identifying the microbial pathogens and performing the antimicrobial susceptibility testing. This information is necessary so that empiric antimicrobial therapy can be shortened and substituted by a pathogen-driven approach. Over the past decade, there have been several advances in rapid microbiological diagnostic testing. Compared to standard techniques that require 48–72 h for final results, these methods can greatly reduce the time to pathogen identification by providing final organism identification within hours from the sample collection or, less efficiently, from the availability of an isolated bacterial colony by culture-based methods.
Consolidation of laboratory medical care into large hospital systems: consolidated hospital network systems open the possibility to merger microbiology diagnostic activities into larger laboratories
Many new innovative microbiological diagnostic approaches have been made available during the last 10 years with a major impact on patient care and public health surveillance [ 49 ]. In parallel, to enhance the cost-effectiveness of the CMLs, European laboratory professionals have streamlined their organization leading to the amalgamation of diagnostic activities and thus restructuring of their professional relationships with clinicians and public health specialists. Through this consolidation process, an operational model has emerged that combines large centralized CMLs performing a large panel and number of tests within a high-throughput analytical platform connected to several distal laboratories dealing locally with urgent analyses at the near point of care testing. The centralization of diagnostic services so that encompassing a large geographical region has given rise to the concept of regional-scale “microbiology laboratories network” or, in another word to “geographically spread laboratories.” Although the volume-driven cost savings associated with such laboratory networks seem self-evident, the consequences for the quality of patient care and infectious disease surveillance and control remain a challenge even if the fast-changing landscape of CMLs in Europe may give a range of opportunities to contribute to improving the quality of patient care but also the early detection and enhanced surveillance of public health threats caused by infectious diseases.
Full automation is currently being required to meet the needs of a changing healthcare system based on consolidated geographically spread microbiology laboratories
During the last decade, most CMLs have encountered many management and financial difficulties, mainly resulting from the gradual and continuous increase in sample volume with limited budgets and personnel shortages. Thus, laboratories have been forced to optimize their workflow to raise their productivity: this improvement must be accompanied by at least a maintained analytical quality, but possibly by an improved clinical value of the generated data. Automation was introduced many years ago in several diagnostic disciplines such as chemistry, clinical pathology, and hematology to increase laboratory productivity and quality. The automation process was by far more complicated in molecular biology and bacteriology settings: this was due to several reasons, including the complexity and variability of sample types, the many different analytical processes, and the insufficient volume of samples. However, the introduction of automation was considered to be also applicable in microbiology in more recent years thanks to the technological improvements currently available. Recently, these new technologies have triggered the development of automated solutions specifically designed for microbiology. In particular, the automation process has been applied to all the pre-analytical steps and to the evaluation of the results by using sophisticated artificial intelligence algorithms. The complete clinical laboratory automation is currently the main organizational challenge for microbiologists [ 50 ].
Question 7. How can you manage the patient with infection/colonization of multidrug-resistant organisms (MDROs)?
Statement 7.1. The application of contact isolation precautions is always recommended for patients known or highly suspected for MDROs.
MDROs including methicillin-resistant Staphylococcus aureus (MRSA), vancomycin-resistant Enterococcus (VRE), extended-spectrum β-lactamase (ESBL) producers, and Klebsiella pneumoniae -producing carbapenemase (KPC) pose significant public health challenges. The prevention and control of MDROs are a global priority.
Traditionally, hospitals have been considered the main reservoir of MDROs. Around 20–40% of nosocomial infections can be mainly attributed to cross-infection via the hands of healthcare personnel. Less frequently, patients can become colonized with nosocomial pathogens by direct contact with contaminated patient care equipment or contaminated surfaces in the healthcare environment [ 51 ].
Current strategies to address MDROs consist of the three following strategies [ 52 ]:
Developing new antimicrobial agents
Increasing antimicrobial stewardship efforts
Interrupting MDROs cross-transmission
Bacteria tend to inhabit specific sites on either in the human body or in the hospital environment which serve as reservoirs for transmission. The reservoirs of resistant organisms include niches in the human microbiome. The microbiota of the skin, respiratory epithelium, and the gastrointestinal tract are altered within a few days in the hospital. Patients’ flora can be deranged by antibiotics, chemotherapy, or acquisition of nosocomial organisms. Patients who are colonized with resistant bacteria serve inadvertently as potential reservoirs for transmission. Colonization pressure, or the proportion of patients in a given unit who are colonized with resistant bacteria, is an independent risk factor for transmission. Surveillance cultures for carbapenem-resistant Enterobacteriaceae (CRE) have been advocated in a number of reports and recommendations as part of an overall strategy to combat it. Active screening for CRE using rectal surveillance cultures has been shown to be highly effective, when part of a comprehensive infection control program, in halting the spread of CRE in healthcare facilities [ 53 ].
Isolation or cohorting of colonized/infected patients is a cornerstone of IPC. Its purpose is to prevent the transmission of microorganisms from infected or colonized patients to other patients, hospital visitors, and HCWs, who may subsequently transmit them to other patients or become infected or colonized themselves. Isolating a patient with highly resistant bacteria is beneficial in stopping the patient-to-patient spread. Isolation measures should be an integral part of any IPC program; however, they are often not applied consistently and rigorously, because they are expensive, time-consuming, and often uncomfortable for patients.
Facilities should have written policies and procedures that identify patients with MDROs and should require that contact precautions are implemented in all practice settings. Communication is a vital component for successful implementation of contact precautions and must occur at all points in the perioperative process.
Question 8. Antimicrobial stewardship: Is a multidisciplinary approach necessary?
Statement 8.1. The three basic requirements of an Antimicrobial Stewardship program are:
The existence of a multidisciplinary antimicrobial stewardship team.
A microbiological report on a fixed basis on the bacterial resistance of the hospital at least annually, if possible stratified by departments or at least for some key departments (e.g. Intensive Care Units).
A report on fixed consumption of antibiotics in the hospital.
Statement 8.2. A multidisciplinary antimicrobial stewardship team should be coordinated by an infectious disease specialist, or by another specialist with documented infectious skills.
Every hospital worldwide should utilize the existing resources to create an effective multidisciplinary team for combating AMR. The best strategies for combating AMR are not definitively established and are likely to vary based on local culture, policy, organization, and routine clinical practice despite several guidelines on the topic [ 44 ].
We propose that the best means of improving programs to contain AMR should involve collaboration among various specialties within a healthcare institution. They should focus on collaboration between all healthcare professionals to shared knowledge and widespread diffusion of practice. The involvement of HCWs may raise their awareness of AMR [ 44 ]. It is essential for any team to have at least one member who is an infectious disease specialist. Pharmacists with advanced training or long-standing clinical experience in infectious diseases are also key actors for the design and implementation of the stewardship program interventions monitoring consumption data of antibiotics. In any healthcare setting, a significant amount of energy should be spent on IPC. Infection control specialists and hospital epidemiologists should be always included in these programs to coordinate efforts on monitoring and preventing HAIs. Microbiologists should actively guide the proper use of tests and the flow of laboratory results. Being involved in providing surveillance data on AMR, they should provide periodic reports on AMR data allowing the multidisciplinary team to determine the ongoing burden of AMR in the hospital. Moreover, timely and accurate reporting of microbiology susceptibility test results allows the selection of more appropriate targeted therapy and may help reduce broad-spectrum antimicrobial use. Surgeons with adequate knowledge in surgical infections and surgical anatomy when involved may audit both antibiotic prescriptions and prevention practices, provide feedback to the prescribers and integrate best practices of antimicrobial use among surgeons, and act as champions among colleagues implementing change within their own sphere of influence. Infections are the main factors contributing to mortality in ICUs. Intensivists have a critical role in treating multidrug-resistant organisms in ICUs in critically ill patients. They have a crucial role in prescribing antimicrobial agents for the most challenging patients and are at the forefront of successful antibiotic prescribing policies. Emergency departments (EDs) represent a particularly important setting for addressing inappropriate antimicrobial prescribing practices, given the frequent use of antibiotics in this setting that sits at the interface of the community and the hospital. Therefore, also ED practitioners should be involved. Without adequate support from hospital management, these programs will be inadequate or inconsistent since the programs do not generate revenue. Engagement of hospital management has been confirmed as a key factor for both developing and sustaining. Finally, an essential participant who has been often unrecognized and underutilized is the “staff nurse” as nurses perform numerous functions that are integral to success.
Question 9. Why and how do you have to monitor antibiotics consumption in the hospitals?
Statement 9.1. It is important to monitor antibiotics consumption. The data of the consumption data of antibiotics should be expressed in specific reports in defined daily doses.
Pharmacy’s contribution to ASPs has significantly evolved over the course of the twenty-first century. Although microbiologists and infection specialist physicians have been conventionally responsible for providing advice on clinical management of infected patients, many pharmacists in clinical practice have now established roles complementing the expertise in multidisciplinary antimicrobial stewardship teams.
Pharmacists’ responsibilities for antimicrobial stewardship include promoting the optimal use of antimicrobial agents. Typical interventions include patient-specific recommendations on therapy; the implementation of policies, education, therapeutic drug monitoring, and participation in antimicrobial stewardship ward rounds [ 54 , 55 ].
Antibiotics are prescribed in up to a third of hospital inpatients, often inappropriately [ 56 ], and more than two thirds of critically ill patients are on antibiotics at any given time during their hospital admission [ 57 ]. Antibiotic use is one of the most important parameters for assessing the impact that an ASP has on a hospital and its patient population, although microbiological resistance and clinical outcomes are also important measures. Antimicrobial measures looking at consumption are the most commonly used measures and are focused on defined daily dose (DDD), usually standardized per 1000 patient-days.
DDD is a metric that was developed in the 1970s and has been further refined and promoted by the WHO Collaborating Centre for Drug Statistics Methodology. DDD is described as “the assumed average maintenance dose per day for a drug used for its main indication in adults” [ 58 ]. In simple terms, a DDD is the amount of drug that a typical patient might receive on any day for therapeutic purposes. An important advantage of using DDDs is the relative ease of hospital systems to report consumption using DDDs: most pharmacy departments have a mechanism to calculate overall prescription, dispensing, or consumption of a quantity of antimicrobials, allowing DDDs/1000 patient-days to be relatively easy to calculate if bed utilization is also available. Additionally, institution-wide consumption can be benchmarked against similar institutions. The landmark guidelines on antimicrobial stewardship by the Infectious Diseases Society of America and Society for Healthcare Epidemiology of America advocated for DDDs/1000 patient-days as a universal metric for hospital-based ASPs [ 59 ].
Question 10. What is the role for the nurse in preventing HAIs?
Statement 10.1. The nurse is an integral part of the multidisciplinary team for the prevention of infections across the surgical pathway.
Statement 10.2. It is important to implement educational and training interventions concerning the prevention of SSIs by following a modality appropriate to the level of education of the patient/caregiver.
The role of the professional nurse in preventing HAIs is significant. The nurse is a member of the healthcare team who leads the rest of the team in practicing prevention strategies to protect the patient from infection [ 60 ]. Some of the most basic strategies resulting in positive patient outcomes include the following:
The practice and promotion of hand hygiene
Consistent use of aseptic technique
Cleaning and disinfection practices
Use of standard precautions
Patient assessment and additional precautions
Patient education
Use of safety devices
Removal of unnecessary invasive devices
Use of bundle strategies for infection prevention
Fit for duty
Nurses play a pivotal role in preventing hospital-acquired infections (HAIs), not only by ensuring that all aspects of their nursing practice are evidence-based, but also through patient education. One of the most important roles nurses have today is patient education. This was once reserved for the physician, but no longer. Today nurses assume more and more responsibility for educating patients and helping them to become responsible for their own health status. Patients need to take a proactive role in their own healthcare. This means they need to comprehend their health status and work to stabilize and prevent or minimize complications such as HAIs. Demographic variables, such as formal education level, reading ability, and barriers to participation in education, must be considered to maximize the effectiveness of self-management education outcomes. Hospital nurses can best educate patients by understanding that discharge planning begins with admission. Nurses have to ensure patients are effectively educated throughout their hospitalization so that they are prepared to care for themselves and participate in the care pathway.
Question 11. Which are the principles of antibiotic prophylaxis?
Statement 11.1. Prolonging antibiotic prophylaxis after surgery is generally not associated with better clinical results.
Statement 11.2. There is no universally recognized intervention for improving the appropriateness of antibiotic prophylaxis in surgery. These interventions must be tailored to the type of surgeon and team to which they are addressed.
Preoperative antibiotic prophylaxis (PAP) has been demonstrated in multiple randomized controlled trials and meta-analyses to reduce the risk of SSIs across different types of surgical procedures [ 61 ].
Given the evidence, systemic PAP is considered to be a key component of perioperative infection prevention bundles [ 62 ]. Although compliance with appropriate timing and spectrum of PAP has improved as a result of quality improvement initiatives, there remain significant deficiencies in compliance with other aspects of PAP such as duration of postoperative antibiotics [ 63 , 64 ]. Given that approximately 15% of all antibiotics in hospitals are prescribed for surgical prophylaxis [ 65 , 66 ], perioperative antibiotic prescribing patterns can be a major driver of some emerging infections (such as C. difficile ) [ 67 , 68 ] and selection of antibiotic resistance, increasing healthcare costs.
Although appropriate PAP plays a pivotal role in reducing the rate of SSIs [ 69 ], other factors that impact SSI rates should not be ignored. PAP should never substitute for good medical practices, such as those of IPC. Perioperative SSI prevention strategies should include attention to basic IPC strategies, surgical technique, hospital and operating room environments, instrument sterilization processes, and perioperative optimization of patient risk factors [ 70 ].
The key elements of appropriate surgical antimicrobial prophylaxis prescribing include the correct antimicrobial indication, drug dose, route, the timing of administration, and duration.
Joint guidelines for PAP in surgery were revised and updated in 2013 by the American Society of Health-System Pharmacists, Infectious Diseases Society of America, Surgical Infection Society, and Society for Healthcare Epidemiology of America [ 68 ]. These guidelines focus on the effective and safe use of AP. Therapeutic serum and tissue concentrations of antimicrobial agents should be present during the period of potential contamination. Additional antibiotic doses may need to be administered intraoperatively for prolonged procedures or for agents with short half-lives. In order to be safe, PAP should have no or few adverse effects and should have the narrowest spectrum of activity necessary to prevent postoperative infections.
There is no evidence that prolonging PAP after surgery can reduce the risk of SSIs. A single preoperative dose is adequate for the majority of procedures. Post-procedural doses of intravenous antibiotics (up to 24 h) may be only required in defined circumstances, such as some cardiac and vascular surgeries.
Below, seven practices for a correct surgical antibiotic prophylaxis are illustrated [ 71 ]:
Antibiotics alone are unable to prevent SSIs. Strategies to prevent SSIs should always include attention to the following:
IPC strategies including correct and compliant hand hygiene practices
Meticulous surgical techniques and minimization of tissue trauma
Hospital and operating room environments
Instrument sterilization processes
Perioperative optimization of patient risk factors
Perioperative temperature, fluid, and oxygenation management
Targeted glycemic control
Appropriate management of surgical wounds
Antibiotic prophylaxis should be administered for operative procedures that have a high rate of postoperative SSI, or when foreign materials are implanted.
Antibiotics given as prophylaxis should be effective against the aerobic and anaerobic pathogens most likely to contaminate the surgical site, i.e., Gram-positive skin commensals or normal flora colonizing the incised mucosae.
Antibiotic prophylaxis should be administered within 120 min prior to the incision. However, administration of the first dose of antibiotics beginning within 30–60 min before the surgical incision is recommended for most antibiotics (e.g., cefazolin), to ensure adequate serum and tissue concentrations during the period of potential contamination. Obese patients ≥ 120 kg require higher doses of antibiotics.
A single dose is generally sufficient. Additional antibiotic doses should be administered intraoperatively for procedures > 2–4 h (typically where duration exceeds two half-lives of the antibiotic) or with associated significant blood loss (> 1.5 L).
There is no evidence to support the use of postoperative antibiotic prophylaxis.
Each institution is encouraged to develop guidelines for the proper surgical prophylaxis.
Knox and Edye [ 63 ] demonstrated that an educational ASP was ineffective in changing surgical prophylactic antibiotic prescribing in an Australian hospital. Although that study was disappointing as far as showing improved behaviors, others have shown that ASPs may have a significant impact on optimizing antibiotic use in surgical prophylaxis practices [ 72 , 73 ]. Van Kasteren et al. [ 73 ] in a prospective multisite study of elective procedures in 13 Dutch hospitals evaluated the quality of prophylaxis auditing before and after an intervention consisting of performance feedback and implementation of national clinical practice guidelines. Antimicrobial use decreased from 121 to 79 DDD/100 procedures, and costs reduced by 25% per procedure. After the intervention, antibiotic choice was inappropriate in only 37.5% of the cases instead of 93.5% expected cases in the absence of any intervention. Prolonged prophylaxis was observed in 31.4% instead of 46.8% expected cases and inappropriate timing in 39.4% instead of the expected 51.8%. Time series analysis showed that all improvements were statistically significant ( P < 0.01). The overall SSI rates before and after the intervention were 5.4% (95% CI 4.3–6.5) and 4.6% (95% CI 3.6–5.4), respectively [ 73 ]. Huh et al. [ 72 ] performed an interrupted time-series study of an ASP relating to surgical prophylaxis in a tertiary care hospital. The ASP consisted of monitoring of performance indicators and implementation of a computerized decision support system. The program was effective in improving multiple measures including the total use of antibiotics, the use of third-generation cephalosporins and aminoglycosides, trends in proportions of resistant bacterial strains such as meropenem-resistant Pseudomonas aeruginosa , and length of stay. Saied et al. [ 12 ] implemented ASPs in 5 tertiary, acute-care surgical hospitals. The ASPs consisted of education aimed at surgeons and anesthesiologists, audit and feedback, and selection of surgeon champions. The efficacy of the intervention on timing and duration of antibiotic prophylaxis varied across hospitals when measured pre- and post-ASP implementation. Local factors such as available resources and stakeholder engagement likely play a role in the conflicting results of ASPs addressing surgical prophylaxis across different settings, as seen in these studies.
Question 12. Which are the principles of antibiotic therapy?
Statement 12.1. It is important to know the local epidemiological context to define therapeutic protocols / guidelines for surgical infections treatment.
Statement 12.2. It is important to frame clinical conditions, in particular to differentiate between critical and non-critical patients.
Statement 12.3. It is important to pursue as much as possible targeted therapy or in any case a de-escalation in order to preserve some molecules: e.g.: carbapenems.
Statement 12.4. It is important to assess properly the duration of therapy based on source control.
Statement 12.5. In the setting of uncomplicated intra-abdominal infections including uncomplicated acute cholecystitis and acute appendicitis post-operative antimicrobial therapy is not necessary.
Statement 12.6. In patients with complicated intra-abdominal infections, when patients are not severely ill and when source control is complete, a short course (3-5 days) of post-operative therapy is suggested.
Statement 12.7. In patients with ongoing or persistent intra-abdominal infections, the decision to continue, revise, or stop antimicrobial therapy should be made on the basis of clinician judgment and laboratory information.
Empirical antibiotic therapy should be based on local epidemiology, individual patient risk factors for difficult-to-treat pathogens, clinical severity of infection, and infection source. Initial antibiotic therapy for surgical infections is empirical in nature because microbiological data (culture and susceptibility results) may require > 24/48 h before they are available for a more detailed analysis. However, the results direct expansion of antimicrobial regimen if it is too narrow and perform a de-escalation if it is too broad [ 74 , 75 ], particularly in critically ill patients where de-escalation strategy is one of the cornerstones of ASPs [ 76 ]. The principles of empiric antibiotic treatment should be defined according to the most frequently isolated microbes, always taking into consideration the local trend of antibiotic resistance. In this era of prevalent drug-resistant microorganisms, the threat of resistance is a source of major concern that cannot be ignored [ 76 ].
In the past 20 years, the incidence of intra-abdominal infections (IAIs) caused by MDROs has risen dramatically [ 76 ]. Quinolone resistance, prevalence of ESBL-producing bacteria, prevalence and mechanisms of carbapenem resistance in the local environment, and the place of recent traveling should be always taken into account when antibiotic therapy is administered empirically. Generally, the most important factors in predicting the presence of resistant pathogens are acquisition in a healthcare setting (particularly if the patient becomes infected in the ICU or has been hospitalized for more than 1 week), corticosteroid use, organ transplantation, baseline pulmonary or hepatic disease, and previous antimicrobial therapy [ 76 , 77 ].
Previous antibiotic therapy is one of the most important risk factors for resistant pathogens [ 78 ]. Inappropriate therapy in critically ill patients may have a strong negative impact on the outcome. An ineffective or inadequate antimicrobial regimen is one of the variables more strongly associated with unfavorable outcomes in critically ill patients. Broad empiric antibiotic therapy should be started as soon as possible in patients with organ dysfunction (sepsis) and septic shock [ 79 , 80 , 81 , 82 , 83 ]. International guidelines for the management of sepsis and septic shock (the Surviving Sepsis Campaign) recommend intravenously administered antibiotics as soon as possible and in any case within the first hour of onset of sepsis and the use of broad-spectrum agents with adequate penetration of the presumed site of infection [ 84 ].
The results of microbiological testing may have great importance for the choice of therapeutic strategy of every patient, in particular, in the adaptation of targeted antibiotic treatment. They provide an opportunity to expand the antibiotic regimen if the initial choice was too narrow but also allow de-escalation of antibiotic therapy if the empirical regimen was too broad. Antibiotic de-escalation has been associated with lower mortality rates in ICU patients and is now considered a key practice for antimicrobial stewardship purposes [ 75 ]. The duration of antibiotic therapy has been studied appropriately in the setting of intra-abdominal infections (IAIs).
In the event of uncomplicated IAIs, the infection involves a single organ and does not extend to the peritoneum. When the source of infection is treated effectively by surgical excision, postoperative antibiotic therapy is not necessary, as demonstrated in managing uncomplicated acute appendicitis or cholecystitis [ 85 , 86 , 87 ].
In 2015, an important prospective study on the appropriate duration of antibiotic therapy in patients with complicated IAIs was published [ 88 ]. The study randomized 518 patients with complicated IAIs and adequate source control to receive antibiotics until 2 days after the resolution of fever, leukocytosis, and ileus, with a maximum of 10 days of therapy (control group), or to receive a fixed course of antibiotics (experimental group) for 4 ± 1 calendar days. In patients with complicated IAIs who had undergone an adequate source control procedure, the outcomes after fixed-duration antibiotic therapy (approximately 4 days) were similar to those after a long course of antibiotics (approximately 8 days) that extended until after the resolution of physiological abnormalities. In this study, most patients were not severely ill.
The high mortality associated with abdominal sepsis requires clinicians to maintain a high index of clinical suspicion of treatment failure and the early diagnosis of ongoing infections. These patients should always be monitored carefully including the potential use of inflammatory response markers.
Below, we report 13 practices in an appropriate antibiotic therapy across the surgical pathway [ 71 ]:
The source of infection should always be identified and controlled as soon as possible.
Antibiotic empiric therapy should be initiated after a treatable surgical infection has been recognized, since microbiological data (culture and susceptibility results) may not be available for up to 48–72 h to guide targeted therapy.
In critically ill patients, empiric broad-spectrum therapy to cover all likely pathogens should be initiated as soon as possible after a surgical infection has been recognized . Empiric antimicrobial therapy should be narrowed once culture and susceptibility results are available and/or adequate clinical improvement is noted.
Empirical therapy should be chosen on the basis of local epidemiology, individual patient risk factors for MDR bacteria and Candida spp., clinical severity, and infection source.
Specimens for microbiological evaluation from the site of infection are always recommended for patients with hospital-acquired or with community-acquired infections at risk for resistant pathogens (e.g., previous antimicrobial therapy, prior infection or colonization with a multidrug-resistant, extensively drug-resistant, and pan-drug-resistant pathogens) and in critically ill patients. Blood cultures should be performed before the administration of antibiotics in critically ill patients.
Antibiotics dose should be optimized to ensure that pharmacokinetic-pharmacodynamic (PK-PD) targets are achieved. This involves prescribing of an adequate dose, according to the most appropriate and right method and schedule to maximize the probability of target attainment.
The appropriateness and need for antimicrobial treatment should be reassessed daily.
Once source control is established, short courses of antibiotic therapy are as effective as longer courses regardless of signs of inflammation.
Intra-abdominal infection: 4 days are as effective as 8 days in moderately ill patients.
Bloodstream infection: 5–7 days are as effective as 7–21 days for most patients.
Ventilator-associated pneumonia: 8 days are as effective as 15 days.
Failure of antibiotic therapy in patients having continued evidence of active infection may require a re-operation for a second source control intervention.
Biomarkers such as procalcitonin (PCT) may be useful to guide duration and/or cessation of antibiotic therapy in critically ill patients.
Clinicians with advanced training and clinical experience in surgical infections should be included in the care of patients with severe infections.
IPC measures, combined with ASPs, should be implemented in surgical departments. These interventions and programs require regular, systematic monitoring to assess compliance and efficacy.
Monitoring of antibiotic consumption should be implemented and feedback provided to all ASP team members regularly (e.g., every 3–6 months) along with resistance surveillance data and outcome measures.
Question 13. How can you manage invasive candidiasis in surgical patients?
Statement 13.1. It is important the knowledge of the risk of developing invasive candidiasis, improve microbiological diagnostics and optimize treatment .
Invasive candidiasis (IC) has a significant impact on morbidity, mortality, length of hospital stay, and healthcare costs in critically ill patients [ 89 ]. The overall mortality for these patients is high. Candidemia increases mortality rates in the range of 20–49% [ 90 ], and the attributable mortality has been calculated to be around 15% [ 91 ]. The severity of illness (APACHE II score > 10, ventilator use for > 48 h), antibiotics, central venous lines, total parenteral nutrition, burns, and immunosuppression are the most common risk factors [ 92 ].
The risk factors for IC are so numerous that most patients could be considered as exhibiting risk factors for IC. But, the use of excessive antifungal agents would be associated with substantially increased overall healthcare costs and might lead to the emergence of resistance. Unfortunately, early diagnosis of IC remains a challenge, and criteria for starting empirical antifungal therapy in ICU patients are poorly defined. To both ensure appropriate and timely antifungal therapy and to avoid unnecessary use of antifungal agents, some authors have developed clinical prediction rules to identify patients at high risk of candidiasis and for whom initiation of empirical antifungal therapy could be justified. However, there are many concerns about these rules: high specificity but low sensitivity. In 2006, a Spanish group, using the database of the Estudio de Prevalencia de CANdidiasis project, identified four predictors of proven invasive Candida infection. Based on these predictors, a score named “Candida score” (CS) was built. In 2009, the same group demonstrated a significant linear association between increasing values of the CS and the rate of invasive Candida infections [ 93 ]. The factors to predict IC were surgery, multifocal colonization, total parenteral nutrition, and severe sepsis. To each risk factor, 1 point was given, and for clinical sepsis, a score of 2 was given. The cutoff value of 2.5 had sensitivity of 81% and specificity of 74% [ 93 ]. Although blood cultures are still considered the gold standard for diagnosis, it has been shown that they are negative in up to 50% of cases [ 94 ]. Thus, non-culture diagnostic techniques based on serological biomarkers detecting fungal cell components and/or antibodies directed against these components have been investigated. All these diagnostic tests may diagnose IC earlier than clinical or culture-based measures.
Among the biomarkers, mannan antigen and antigen-antibody complex showed higher sensitivity and specificity when combined together [ 95 ]. In a meta-analysis of 14 studies, 7 of which were performed in non-neutropenic critically ill patients; the sensitivity and specificity of mannan and anti-mannan IgG were 58% and 93%, and 59% and 83%, respectively. Values for the combined assay were 83 and 86%, with the best performances for C. albicans , C. glabrata , and C. tropicalis infection [ 95 ]. The 1,3-beta- d -glucan (BG) is a fungal cell wall antigen that can be detected in the blood of patients with a sensitivity of 56–93% and a specificity of 71–100% for IC [ 96 ]. Thanks to its high negative predictive value, BG is potentially useful for the therapy decision-making process and discontinuing of empirical antifungal therapy. An integrated strategy with BG and CS helped to withhold or discontinue treatment, saving health costs without increasing mortality in 198 severely ill patients admitted to ICU with sepsis and a CS > 3 [ 97 ]. Once the diagnosis is made, early systemic treatment is warranted. The armamentarium of drugs for the treatment of candidiasis currently comprises 3 major drug classes: the polyenes, azoles, and echinocandins.
The majority of patients with candidemia have indwelling CVCs when the diagnostic blood culture is obtained [ 98 ], but differentiating between CVC- and non-CVC-related candidemia is not always straightforward. C. parapsilosis is particularly frequent as a cause of CVC infection. There is compelling evidence that CVC removal is associated with higher rates of treatment success and lower mortality rates as compared with CVC retention [ 98 ]. Despite contradictory data from a post hoc analysis of 2 clinical trials [ 98 ], it is generally accepted that indwelling CVCs should be removed as early as possible in all patients with candidemia [ 99 ]. CVCs should be urgently removed in patients with septic shock. For clinically stable patients for whom immediate CVC removal presents significant difficulties, for example, due to limited vascular access, establishing a diagnosis of CVC infection may be of importance.
Question 14. Which are the principles of antibiotic therapy in critically ill patients?
Statement 14.1. In critically ill patients, antibiotic therapy should be prescribed using a severity and risk stratification driven approach.
Statement 14.2. It is important to support the need for better identification of patients at risk of MDROs infection, more accurate diagnostic tools enabling a rule-in/rule-out approach for bacterial sepsis, the use of adequate dosing and administration schemes to ensure the attainment of pharmacokinetics/pharmacodynamics targets, concomitant source control when appropriate, and a systematic reappraisal of initial therapy in an attempt to minimize collateral damage on commensal ecosystems through de-escalation and treatment-shortening whenever conceivable.
The rapid global spread of multiresistant bacteria and loss of antibiotic effectiveness increases the risk of initial inappropriate antibiotic therapy (IAT) and poses a serious threat to patient safety especially in critically ill patients. A systematic review and meta-analysis of published studies to summarize the effect of appropriate antibiotic therapy or IAT against Gram-negative bacterial infections in the hospital setting was published in 2014 [ 100 ]. Using a large set of studies, the authors found that IAT is associated with a number of serious consequences, including an increased risk of hospital mortality.
Infections caused by drug-resistant, Gram-negative organisms represent a considerable financial burden to healthcare systems due to the increased costs associated with the resources required to manage the infection, particularly longer hospital stays. However, given the impact of early and broad-spectrum empirical therapy and the emphasis on this in international guidelines, there is a low threshold for initiating antibiotics in many patients with suspected infection. This has led to the widespread use of antibiotics in critically ill patients, which is often unnecessary or inappropriate [ 101 ].
The massive consumption of antibiotics in the ICU is responsible for substantial ecological side effects that promote the dissemination of MDROs. Strikingly, up to half of ICU patients receiving empirical antibiotic therapy have no definitively confirmed infection, while de-escalation and shortened treatment duration are insufficiently considered in those with documented sepsis.
Published data notably support [ 102 ] the following:
The need for better identification of patients at risk of MDROs infection
More accurate diagnostic tools enabling a rule-in/rule-out approach for bacterial sepsis
An individualized reasoning for the selection of single-drug or combination empirical regimen
The use of adequate dosing and administration schemes to ensure the attainment of PK-PD targets
Concomitant source control when appropriate
A systematic reappraisal of initial therapy in an attempt to minimize collateral damage on commensal ecosystems through de-escalation and treatment shortening whenever conceivable
Several trials found PCT-guided antibiotic stewardship to reduce antibiotic exposure and associated side effects among patients with respiratory infection and sepsis [ 103 ]. Decisions regarding antibiotic use in an individual patient are complex and should be based on the pre-test probability for bacterial infection, the severity of presentation, and the results of the PCT. In the context of a low pre-test probability for bacterial infections and a low-risk patient, a low PCT level helps to rule out bacterial infection and empiric antibiotic therapy can be avoided. In the context of a high pre-test probability for bacterial infections and/or a high-risk patient with sepsis, monitoring of PCT over time helps to track the resolution of infection and decisions regarding the early stop of antibiotic treatment. Although these concepts have been successful in several respiratory infection and sepsis trials, some studies failed to show an added benefit of PCT due to factors such as low protocol adherence and relying on single rather than repeat PCT measurements.
In this era of AMR, another interesting strategy is a therapeutic approach based on patient risk stratification. Especially for Gram-negative MDRO infections, an approach based on the patient risk stratification could improve outcomes and avoid antibiotic misuse.
This approach could help physicians to avoid antibiotic overuse as well as to start promptly with the most appropriate antibiotic regimen. Several risk factors for Gram-negative MDRO infections have been identified. These include prior infection or colonization with Gram-negative MDROs, antibiotic therapy in the past 90 days, poor functional status performance, hospitalization for more than 2 days in the past 90 days, occurrence five or more days after admission to an acute hospital, receiving hemodialysis, and immunosuppression [ 104 ]. Moreover, prior receipt of carbapenems, broad-spectrum cephalosporins, and fluoroquinolones has been associated specifically with MDR Pseudomonas aeruginosa [ 105 ].
Recently, the high mortality and mortality associated with multidrug-resistant Gram-negative bacteria along with limited treatment options have led to a resurgence in the use of the nephrotoxic drug colistin. Fortunately, several new antibiotic agents with activity against Gram-negative MDROs, including ceftazidime/avibactam and ceftolozane/tazobactam, have become available. Further studies are needed to elucidate their place in therapy and their impact on real-world outcomes such as length of stay and mortality, especially for ICU patients; however, these are the few resources we have and should not be wasted unnecessarily.
Question 15. Who is the surgeon champion?
Statement 15.1. To be a champion in preventing and managing infections in surgery means to create a culture of collaboration in which infection prevention, antimicrobial stewardship and correct surgical approach are of high importance.
There is sometimes a false impression that HAIs are adequately controlled. However, with multidrug-resistant bacteria increasing, such as MRSA, VRE, carbapenem-resistant Enterobacteriaceae (CRE), such infections are more than ever a public health threat. It is well known that HAIs tend to show higher resistance rates to antibiotics than community-acquired infections.
Patients in hospitals are often exposed to multiple risk factors for the acquisition of multidrug-resistant bacteria. Acute care facilities are important sites for the development of AMR. The intensity of care together with populations highly susceptible to infection creates an environment that facilitates both the emergence and transmission of resistant organisms.
Surgeons with satisfactory knowledge in surgical infections involved in both IPC team and in the ASPs may integrate the best practice among surgeons. Although the surgeon’s impact on the incidence of SSIs has not yet been examined in a comprehensive manner, some reports have reported that the incidence of SSIs varies widely between hospitals and between surgeons [ 106 , 107 ], suggesting that working practices play a critical role in the prevention of these infections and that more may be done to improve infection control in routine surgical practice.
Very few studies have focused on the relationship between ASPs and surgeons. In 2015, Çakmakçi [ 108 ] suggested that the engagement of surgeons in ASPs might be crucial to their success. However, in 2013, Duane et al. [ 109 ] showed poor compliance with surgical services with ASP recommendations. A retrospective study by Sartelli et al. [ 110 ] showed that implementation of an education-based ASP achieved a significant improvement in all antimicrobial agent prescriptions and a reduction in antimicrobial drug consumption. In a surgical unit performing mainly elective major abdominal surgery and emergency surgery, both a local protocol of surgical prophylaxis and a set of guidelines for the management of intra-abdominal infections (IAIs) and control of antimicrobial agent use were introduced. Comparing the pre-intervention and post-intervention periods, the mean total monthly antimicrobial use decreased by 18.8%, from 1074.9 defined DDDs per 1000 patient-days to 873.0 DDDs per 1000 patient-days after the intervention. The model was based on the concept of the “surgeon champion.” The “champion” was a surgeon who on a day-to-day basis worked within the surgical unit, promoting and maintaining a culture in which IPC appropriate use of antibiotics was of high importance. Identifying a local opinion leader to serve as a champion may be important because the “surgeon champion” may integrate the best clinical practices and drive the colleagues in changing behaviors. We believe that the concept of the “surgeon champion” can be a crucial way to improve IPC across the surgical pathway.
Question 16. Which are the principles of source control?
Statement 16.1. The timing and adequacy of source control are important in the management of surgical infections; late and/or incomplete procedures may have severely adverse consequences on outcome especially in critically ill patients.
Source control encompasses all measures undertaken to eliminate the source of infection, reduce the bacterial inoculum, and correct or control anatomic derangements to restore normal physiologic function [ 111 , 112 ].
As a general principle, every verified source of infection should be controlled as soon as possible. The level of urgency of treatment is determined by the affected organs, the relative speed at which clinical symptoms progress and worsen, and the underlying physiological stability of the patient. Non-operative interventional procedures include percutaneous drainages of abscesses. Ultrasound- and CT-guided percutaneous drainage of abdominal and extraperitoneal abscesses in selected patients are safe and effective. The principal cause for failure of percutaneous drainage is misdiagnosis of the magnitude, extent, complexity, and location of the abscess [ 113 ].
Surgery is the most important therapeutic measure to control surgical infections. In the setting of intra-abdominal infections, the primary objectives of surgical intervention include determining the cause of peritonitis, draining fluid collections, and controlling the origin of the abdominal sepsis. In patients with intra-abdominal infections, surgical source control entails resection or suture of a diseased or perforated viscus (e.g., diverticular perforation, gastro-duodenal perforation), removal of the infected organ (e.g., appendix, gallbladder), debridement of necrotic tissue, resection of ischemic bowel, and repair/resection of traumatic perforations with primary anastomosis or exteriorization of the bowel.
In certain circumstances, infection not completely controlled may trigger an excessive immune response and local infection may progressively evolve into sepsis, septic shock, and organ failure. Such patients can benefit from immediate and aggressive surgical re-operations with subsequent re-laparotomy strategies, to curb the spread of organ dysfunctions caused by ongoing peritonitis. Surgical strategies following an initial emergency laparotomy include subsequent “re-laparotomy on demand” (when required by the patient’s clinical condition) as well as planned re-laparotomy in the 36–48h postoperative period [ 114 ].
On-demand laparotomy should be performed only when absolutely necessary and only for those patients who would clearly benefit from additional surgery. Planned re-laparotomies, on the other hand, are performed every 36–48 h for purposes of inspection, drainage, and peritoneal lavage of the abdominal cavity. The concept of a planned re-laparotomy for severe peritonitis has been debated for over 30 years. Re-operations are performed every 48 h for reassessing the peritoneal inflammatory process until the abdomen is free of ongoing peritonitis; then the abdomen is closed. The advantages of the planned re-laparotomy approach are optimization of resource utilization and reduction of the potential risk for gastrointestinal fistulas and delayed hernias. The results of a clinical trial published in 2007 by Van Ruler et al. [ 115 ] investigating the differences between on-demand and planned re-laparotomy strategies in patients with severe peritonitis found few advantages for the planned re-laparotomy strategy; however, the study mentioned that this latter group exhibited a reduced need for additional re-laparotomies, decreased patient dependency on subsequent healthcare services, and decreased overall medical costs.
An open abdomen (OA) procedure is the best way of implementing re-laparotomies. Open abdomen (OA) procedure is defined as intentionally leaving the fascial edges of the abdomen unapproximated (laparostomy). The abdominal contents are exposed and protected with a temporary coverage. The OA technique, when used appropriately, may be useful in the management of surgical patients with severe abdominal sepsis [ 116 ]. However, the role of the OA in the management of severe peritonitis is still being debated. The role of the OA in the management of severe peritonitis has been a controversial issue [ 116 ]. Although guidelines suggest not to routinely utilize the open abdomen approach for patients with severe intra-peritoneal contamination undergoing emergency laparotomy for intra-abdominal sepsis, OA has now been accepted as a strategy in treating physiologically deranged patients with acute peritonitis.
Question 17. What is the role of the biomarkers in surgery?
Statement 17.1. C-reactive protein (CRP) and procalcitonin (PCT) can help clinicians to diagnose surgical infections.
Statement 17.2. PCT can help clinicians in early discontinuation of antibiotics in critically ill patients and in patients undergoing intervention for acute peritonitis.
Although more than a hundred biomarkers have been studied, only a limited number of them became routinely available in clinical practice. CRP and PCT are the more frequently studied and used biomarkers.
Serum CRP is an acute-phase protein synthesized exclusively in the liver. Its secretion is initiated 4 to 6 h after an inflammatory insult (effect mediated by cytokines namely interleukin-6), and its concentration doubles every 8 h with a peak at 36–50 h [ 117 ]. The sole determinant of CRP plasmatic levels is its synthesis rate, which is proportional to the intensity of the inflammatory insult. Its production and elimination are not influenced by renal replacement therapy or immunosuppression (both systemic steroids and neutropenia). It has a sensitivity of 68–92% and a specificity of 40–67% as a marker of bacterial infection. Its low specificity and inability to differentiate bacterial infections from non-infectious causes of inflammation make CRP of limited diagnostic value [ 118 ]. The available assays for CRP measurement are reliable, stable, reproducible, rapid, inexpensive, and present an acceptable limit of detection (0.3–5 mg/L). CRP has been analyzed in multiple clinical contexts, but only a small number of studies have focused on its use for optimizing antibiotic therapy [ 119 ]. In primary care, CRP improves the assessment of the severity of infection and extent of inflammation [ 120 ] and performs better in predicting the diagnosis of pneumonia than any individual or combination of clinical signs and symptoms. A Cochrane review [ 121 ] demonstrated reduced antibiotic prescription with CRP testing, which led to its incorporation in the National Institute for Health and Care Excellence (NICE) guidelines for the diagnosis of pneumonia. CRP has been reported to be useful in the diagnosis of appendicitis; however, it lacks specificity. Multiple studies have examined the sensitivity of CRP level alone for the diagnosis of appendicitis in patients selected to undergo appendectomy. Gurleyik et al. noted a CRP sensitivity of 96.6% in 87 of 90 patients with a histologically proven disease [ 122 ]. Similarly, Shakhatreh found a CRP sensitivity of 95.5% in 85 of 89 patients with histologically proven appendicitis [ 123 ]. Asfar et al. reported a CRP sensitivity of 93.6% in 78 patients undergoing appendectomy [ 124 ].
PCT is a precursor protein of calcitonin that can be produced ubiquitously throughout the body. It is released 3–4 h after an inflammatory stimulus with a plasmatic peak within 6–24 h and a half-life ranging from 22 to 35 h. Its plasmatic levels are markedly influenced by renal function, different techniques of renal replacement therapy, and neutropenia. It showed a sensitivity of 77% and a specificity of 79% for early diagnosis of sepsis in critically ill patients [ 117 , 118 ]. PCT is the most widely studied biomarker for antibiotic stewardship. It has been tested as an aid to the initiation and/or discontinuation of antibiotics, both in children and adults presenting with distinct sources of infection and in different scenarios. Multiple trials have investigated the benefits of using serum PCT levels to guide whether and for how long antibiotic therapy is used—a process referred to as a PCT-guided antibiotic stewardship—in patients with infection in the ICU [ 125 , 126 , 127 , 128 , 129 , 130 , 131 , 132 , 133 , 134 , 135 ]. Several studies have demonstrated the benefits of using serum PCT levels to guide antibiotic therapy in patients undergoing intervention for acute peritonitis [ 136 , 137 ].
Question 18. Who are the patients at high risk for surgical site infections?
Statement 18.1. A number of risk factors are known to increase the incidence of SSIs, they can be effective at three different levels: patient, operative (surgical procedure-related) and institutional level (hospital related).
Statement 18.2. Although multiple strategies exist for identifying surgical patients at high risk for SSIs, no one strategy is superior for all patients and further efforts are necessary to determine if risk stratification in combination with risk modification can reduce SSIs in this patients’ population.
SSIs are a significant healthcare quality issue, resulting in increased morbidity, disability, length of stay, mortality, resource utilization, and costs. Identification of high-risk patients may improve preoperative counseling, inform resource utilization, and allow modifications in perioperative management to optimize outcomes.
Many risk factors are beyond practitioner control, but optimizing perioperative conditions can certainly help decrease infection risk [ 138 ]. High-risk surgical patients may be identified on the basis of individual risk factors or combinations of factors. In particular, statistical models and risk calculators may be useful in predicting infectious risks, both in general and for SSIs. These models differ in the number of variables: inclusion of preoperative, intraoperative, or postoperative variables; ease of calculation; and specificity for particular procedures. Furthermore, the models differ in their accuracy in stratifying risk.
Although multiple strategies exist for identifying surgical patients at high risk for SSIs, no strategy is superior for all patients, and further efforts are necessary to determine if the risk stratification in combination with risk modification can reduce SSIs in this patient population [ 138 ].
Early evaluation of perioperative SSI risk factors and patient risk stratification could be of great value in the development of predictive risk models [ 139 ]. Predictive risk models could, in turn, assist surgeons and their patients in the clinical decision-making process (e.g., counseling patients on the appropriateness and risks of surgery). In addition, risk models could be used to develop targeted perioperative prevention strategies and diagnostic care process models and improve risk adjustment for risk modeling used in the public reporting of SSI as a quality metric [ 139 ].
However, a study reviewing SSIs in patients undergoing colorectal resections (C-SSIs), identified from an institutional ACS-NSQIP dataset (2006 to 2014), showed that published risk prediction models do not accurately predict C-SSI in their own independent institutional dataset [ 140 ]. Application of externally developed prediction models to any individual practice must be validated or modified to account for the institution and case-mix specific factors. This questions the validity of using externally or nationally developed models for “expected” outcomes and interhospital comparisons.
Question 19. How can you care post-operative wounds to prevent surgical site infections?
Statement 19.1. Advanced dressing of any type should not be used for primarily closed surgical wounds for the purpose of preventing SSI.
Statement 19.2. The surgical wound dressing can be removed for a minimum of 48 hours after surgery unless leakage occurs. There is no evidence that extending medication time implies a reduction in SSIs.
Appropriate surgical wound and incision management in the postoperative time period is imperative to prevent SSIs. The term “surgical wound” used in this document refers to a wound created when an incision is made with a scalpel or other sharp cutting device and then closed in the operating room by suture, staple, adhesive tape, or glue and resulting in a close approximation of the skin edges [ 141 ]. It is traditional to cover such wounds with a dressing, which acts as a physical barrier to protect the wound from contamination from the external environment until it becomes impermeable to microorganisms.
To assess the effects of wound dressings compared with no wound dressings, and the effects of alternative wound dressings, in preventing SSIs in surgical wound healing by primary intention, a Cochrane review was published in 2016 [ 142 ]. The authors concluded that it is uncertain whether covering surgical wound healing by primary intention with wound dressings reduces the risk of SSI, or whether any particular wound dressing is more effective than others in reducing the risk of SSI, improving scarring, reducing pain, improving acceptability to patients, or is easier to remove. Most studies in this review were small and at a high or unclear risk of bias. Based on the current evidence, decision-makers may wish to base decisions about how to dress a wound following surgery on dressing costs as well as patient preference.
The WHO Global Guidelines for the Prevention of SSIs [ 5 , 6 ] suggest not using any type of advanced dressing over a standard dressing on primarily closed surgical wounds for the purpose of preventing SSI. Low-quality evidence from ten RCTs shows that advanced dressings applied on primarily closed incisional wounds do not significantly reduce SSI rates compared to standard wound dressings. Postoperative care bundles recommend that surgical dressings be kept undisturbed for a minimum of 48 h after surgery unless leakage occurs [ 143 , 144 ].
Question 20. How can we engage surgeons in appropriate infection prevention and management?
Statement 20.1. Active education techniques, such as academic detailing, consensus building sessions and educational workshops, should be implemented in each hospital worldwide according to its own resources.
Statement 20.2. Surgeons with satisfactory knowledge in surgical infections should be involved in the infection control team and recognized as “champions” by the hospital's administration.
Surgeons should be involved in guideline development, and their implementation should translate practice recommendations into a protocol or pathway that specifies and coordinates responsibilities and timing for particular actions among a multidisciplinary team. Although both the WHO [ 5 , 6 ] and CDC [ 7 ] have recently published guidelines for the prevention of SSIs, knowledge and awareness of IPC measures among surgeons are often inadequate and a great gap exists between the best evidence and clinical practice with regards to SSI prevention.
Education is crucial in improving HCW behaviors towards HAIs. Effective prevention and management of HAIs is a process requiring a fundamental understanding of the evolving relationship between inappropriate prevention and management and the prevalence of HAIs and the emergence of AMR. However, because medical professionals have already established their knowledge, attitudes, and behaviors, it is difficult to change their deeply established views and behaviors. Increasing knowledge may influence their perceptions and motivate them to change behavior. Education and training represent an important component for the accurate implementation of recommendations. Education of all health professionals in preventing HAIs should begin at the undergraduate level and be consolidated with further training throughout the postgraduate years. Hospitals are responsible for educating clinical staff about IPC programs. Active education techniques, such as academic detailing, consensus building sessions and educational workshops, should be implemented in each hospital worldwide according to its own resources. Efforts to improve educational programs are required, and it is necessary that every hospital worldwide develops appropriate educational programs to drive HCWs towards correct behaviors in the prevention and management of HAIs. The purpose of training and educating healthcare professionals should be to ensure both individual understanding and a team approach with shared knowledge, skills, and attitudes towards the prevention and management of HAIs.
Peer-to-peer role modeling and champions on an interpersonal level have been shown to positively influence the implementation of infection control practices. Many practitioners use educational materials or didactic continuing medical education sessions to keep up to date. However, these strategies might not be very effective in changing practice, unless education is interactive and continuous and includes discussion of evidence, local consensus, feedback on performance (by peers), and making personal and group learning plans. Identifying a local opinion leader to serve as a champion may be important because the “champion” may integrate best clinical practices and drive the colleagues in changing behaviors, working on a day-to-day basis, and promoting a culture in which IPC is of high importance. Surgeons with satisfactory knowledge in surgical infections may provide feedback to the prescribers, integrate the best practices among surgeons and implement changes within their own sphere of influence interacting directly with the IPC team.
Raising awareness of IPC to stakeholders is another crucial factor in changing behaviors. Probably, clinicians are more likely to comply with the guidelines when they have been involved in developing the recommendations. One way to engage health professionals in guideline development and implementation is to translate practice recommendations into a protocol or pathway that specifies and coordinates responsibilities and timing for particular actions among a multidisciplinary team.
Conclusions
Leading international organizations, such as the WHO, acknowledge that collaborative practice is essential for achieving a concerted approach to providing care [ 1 ]. Prevention and management of infections across the surgical pathway should always focus on the collaboration between all healthcare professionals with shared knowledge and widespread diffusion of best practice. In the Appendix, the statements approved with an agreement ≥ 80% are reported.
Availability of data and materials
Not applicable.
Abbreviations
- Antimicrobial resistance
Antimicrobial stewardship program
Catheter-associated urinary tract infection
Centers for Disease Control and Prevention
Clostridioides difficile infection
Central line-associated bloodstream infection
Clinical microbiology laboratory
Central venous catheter-related bloodstream infection
C-reactive protein
Candida score
Central venous catheter
Defined daily dose
European Center for Disease Prevention and Control
Fecal microbiota transplantation
Healthcare-associated infection
Healthcare worker
Inappropriate antibiotic therapy
Invasive candidiasis
Intensive care unit
Infection prevention and control
Multidrug-resistant organism
Perioperative antibiotic prophylaxis
Procalcitonin
Proton pump inhibitor
Surgical site infection
World Health Organization
World Health Organization. In: Framework for action on interprofessional education & collaborative practice. 2010. https://apps.who.int/iris/handle/10665/70185 . Accessed 23 Oct 2019.
Haque M, Sartelli M, McKimm J, Abu BM. Health care-associated infections - an overview. Infect Drug Resist. 2018;11:2321–33.
Article PubMed PubMed Central Google Scholar
World Health Organization. In: Report on the burden of endemic health care-associated infection worldwide. 2011. https://apps.who.int/iris/handle/10665/80135 . Accessed 23 Oct 2019.
Schreiber PW, Sax H, Wolfensberger A, Clack L, Kuster SP; Swissnoso. The preventable proportion of healthcare-associated infections 2005-2016: systematic review and meta-analysis. Infect Control Hosp Epidemiol. 2018;39:1277-1295.
Article Google Scholar
Allegranzi B, Zayed B, Bischoff P, Kubilay NZ, de Jonge S, de Vries F, et al. New WHO recommendations on intraoperative and postoperative measures for surgical site infection prevention: an evidence-based global perspective. Lancet Infect Dis. 2016;16:e288–303.
Article PubMed Google Scholar
Allegranzi B, Bischoff P, de Jonge S, Kubilay NZ, Zayed B, Gomes SM, et al. New WHO recommendations on preoperative measures for surgical site infection prevention: an evidence-based global perspective. Lancet Infect Dis. 2016;16:e276–87.
Berríos-Torres SI, Umscheid CA, Bratzler DW, Leas B, Stone EC, Kelz RR, et al. Centers for disease control and prevention guideline for the prevention of surgical site infection, 2017. JAMA Surg. 2017;152:784–91.
World Health Organization. In: Implementation manual to support the prevention of surgical site infections at the facility level - turning recommendations into practice. 2018. https://www.who.int/infection-prevention/publications/implementation-manual-prevention-surgical-site-infections.pdf . Accessed 23 Oct 2019.
Abbas M, Tartari E, Allegranzi B, Pittet D, Harbarth S. The effect of participating in a surgical site infection (SSI) surveillance network on the time trend of SSI rates: a systematic review. Infect Control HospEpidemiol. 2017;38:1364–6.
Google Scholar
Marchi M, Pan A, Gagliotti C, Morsillo F, Parenti M, Resi D. The Italian national surgical site infection surveillance programme and its positive impact, 2009 to 2011. Euro Surveill. 2014;19(21).
Centers for Disease Control and Prevention. In: National and state healthcare-associated infections progress report. 2016. https://www.cdc.gov/hai/progress-report . Accessed 23 Oct 2018.
Allegranzi B, Aiken AM, Kubilay Z, Nthumba P, Barasa J, Okumu G, et al. A multimodal infection control and patient safety intervention to reduce surgical site infections in Africa: a multicentre, before-after cohort study. Lancet Infect Dis. 2018;18:507–15.
Zingg W, Storr J, Park BJ, Ahmad R, Tarrant C, Castro-Sanchez E, et al. Implementation research for the prevention of antimicrobial resistance and healthcare-associated infections; 2017 Geneva infection prevention and control (IPC)-think tank (part 1). Antimicrob Resist Infect Control. 2019;8:87.
Storr J, Twyman A, Zingg W, Damani N, Kilpatrick C, Reilly J, et al. Core components for effective infection prevention and control programmes: new WHO evidence-based recommendations. Antimicrob Resist Infect Control. 2017;6:6.
World Health Organization. In: Protocol for surgical site infection surveillance with a focus on settings with limited resources.2018. https://www.who.int/infection-prevention/tools/surgical/SSI-surveillance-protocol.pdf . Accessed 23 Oct 2019.
Saint S, Krein S and Stock R. Preventing hospital infections: real-world problems, realistic solutions. Oxford, UK: Oxford University Press. Accessed 23 Oct 2019. https://doi.org/10.1093/med/9780199398836.001.0001/med-9780199398836
Sartelli M, Di Bella S, McFarland LV, Khanna S, Furuya-Kanamori L, Abuzeid N, et al. 2019 update of the WSES guidelines for management of Clostridioides (Clostridium) difficile infection in surgical patients. World J Emerg Surg. 2019;14:8.
Bagdasarian N, Rao K, Malani PN. Diagnosis and treatment of Clostridium difficile in adults: a systematic review. JAMA. 2015;313:398–408.
Article PubMed PubMed Central CAS Google Scholar
Kwok CS, Arthur AK, Anibueze CI, Singh S, Cavallazzi R, Loke YK. Risk of Clostridium difficile infection with acid suppressing drugs and antibiotics: meta-analysis. Am J Gastroenterol. 2012;107:1011–9.
Article CAS PubMed Google Scholar
Bartlett JG. The case for vancomycin as the preferred drug for treatment of Clostridium difficile infection. Clin Infect Dis. 2008;46:1489–92.
Teasley DG, Gerding DN, Olson MM, Peterson LR, Gebhard RL, Schwartz MJ, et al. Prospective randomised trial of metronidazole vs vancomycin for Clostridium difficile-associated diarrhea and colitis. Lancet. 1983;2:1043–6.
Fekety R, Silva J, Buggy B, Deery HG. Treatment of antibiotic-associated colitis with vancomycin. J Antimicrob Chemother. 1984;14:97–102.
Louie TJ, Miller MA, Mullane KM, Weiss K, Lentnek A, Golan Y, et al. Fidaxomicin versus vancomycin for Clostridium difficile infection. N Engl J Med. 2011;364:422–31.
Cornely OA, Crook DW, Esposito R, Poirier A, Somero MS, Weiss K, et al. Fidaxomicin versus vancomycin for infection with Clostridium difficile in Europe, Canada, and the USA: a double-blind, non-inferiority, randomised controlled trial. Lancet Infect Dis. 2012;12:281–9.
Zar FA, Bakkanagari SR, Moorthi KM, Davis MB. A comparison of vancomycin and metronidazole for the treatment of Clostridium difficile-associated diarrhea, stratified by disease severity. Clin Infect Dis. 2007;45:302–7.
Al-Nassir WN, Sethi AK, Nerandzic MM, Bobulsky GS, Jump RL, Donskey CJ. Comparison of clinical and microbiological response to treatment of Clostridium difficile-associated disease with metronidazole and vancomycin. Clin Infect Dis. 2008;47:56–62.
Johnson S, Louie TJ, Gerding DN, Cornely OA, Chasan-Taber S, Fitts D, et al. Vancomycin, metronidazole, or tolevamer for Clostridium difficile infection: results from two multinational, randomized, controlled trials. Clin Infect Dis. 2014;59:345–54.
Debast SB, Bauer MP, Kuijper EJ. European Society of Clinical Microbiology and Infectious Diseases. European Society of Clinical Microbiology and Infectious Diseases: update of the treatment guidance document for Clostridium difficile infection. Clin Microbiol Infect. 2014;20(Suppl 2):1–26.
Eiland EH 3rd, Sawyer AJ, Massie NL. Fidaxomicin use and clinical outcomes for Clostridium difficile-associated diarrhea. Infect Dis Clin Pract (Baltim Md). 2015;23:32–5.
Vargo CA, Bauer KA, Mangino JE, Johnston JE, Goff DA. An antimicrobial stewardship program’s real-world experience with fidaxomicin for treatment of Clostridium difficile infection: a case series. Pharmacotherapy. 2014;34:901–9.
Bakken JS, Borody T, Brandt LJ, Brill JV, Demarco DC, Franzos MA, et al. Treating Clostridium difficile infection with fecal microbiota transplantation. Clin Gastroenterol Hepatol. 2011;9:1044–9.
Bartlett JG. Bezlotoxumab - a new agent for Clostridium difficile infection. N Engl J Med. 2017;376:381–2.
Mermel LA, Allon M, Bouza E, Craven DE, Flynn P, O’Grady NP, et al. Clinical practice guidelines for the diagnosis and management of intravascular catheter-related infection: 2009 Update by the Infectious Diseases Society of America. Clin Infect Dis. 2009;49:1–45.
Callister D, Limchaiyawat P, Eells SJ, Miller LG. Risk factors for central line-associated bloodstream infections in the era of prevention bundles. Infect Control Hosp Epidemiol. 2015;36:214–6.
Pronovost P, Needham D, Berenholtz S, Sinopoli D, Chu H, Cosgrove S, et al. An intervention to decrease catheter-related bloodstream infections in the ICU. N Engl J Med. 2006;355:2725–32.
Entesari-Tatafi D, Orford N, Bailey MJ, Chonghaile MNI, Lamb-Jenkins J, Athan E. Effectiveness of a care bundle to reduce central line-associated bloodstream infections. Med J Aust. 2015;202:247–9.
Exline MC, Ali NA, Zikri N, Mangino JE, Torrence K, Vermillion B. Beyond the bundle - journey of a tertiary care medical intensive care unit to zero central line-associated bloodstream infections. Crit Care. 2013;17:R41.
Hakko E, Guvenc S, Karaman I, Cakmak A, Erdem T, Cakmakci M. Long-term sustainability of zero central-line associated bloodstream infections is possible with high compliance with care bundle elements. East Mediterr Health J. 2015;21:293–8.
McEwen SA, Collignon PJ. Antimicrobial resistance: a One Health perspective. Microbiol Spectr. 2018;6(2).
Cassini A, Högberg LD, Plachouras D, Quattrocchi A, Hoxha A, Simonsen GS, et al. Attributable deaths and disability-adjusted life-years caused by infections with antibiotic-resistant bacteria in the EU and the European Economic Area in 2015: a population-level modelling analysis. Lancet Infect Dis. 2019;19:56–66.
European Centre for Disease Prevention and Control. In: ECDC country visit to Italy to discuss antimicrobial resistance issues. 2017. https://ecdc.europa.eu/en/publications-data/ecdc-country-visit-italy-discuss-antimicrobial-resistance-issues . Accessed 23 Oct 2019.
Organization for Economic Co-operation and Development. In: Antimicrobial resistance - policy insights.2016. https://www.oecd.org/health/health-systems/AMR-Policy-Insights-November2016.pdf . Accessed 23 Oct 2019.
Italian Ministry of Health. In: Piano nazionale di contrasto all’antibiotico resistenza (PNCAR) 2017-2020.2017. http://www.salute.gov.it/imgs/C_17_pubblicazioni_2660_allegato.pdf . Accessed 23 Oct 2019.
Sartelli M, Labricciosa FM, Barbadoro P, Pagani L, Ansaloni L, Brink AJ, et al. The Global Alliance for Infections in Surgery: defining a model for antimicrobial stewardship-results from an international cross-sectional survey. World J Emerg Surg. 2017;12:34.
Goff DA, Kullar R, Goldstein EJC, Gilchrist M, Nathwani D, Cheng AC, et al. A global call from five countries to collaborate in antibiotic stewardship: united we succeed, divided we might fail. Lancet Infect Dis. 2017;17:e56–63.
Brink AJ, Messina AP, Feldman C, Richards GA, van den Bergh D. Netcare Antimicrobial Stewardship Study Alliance. From guidelines to practice - a pharmacist-driven prospective audit and feedback improvement model for peri-operative antibiotic prophylaxis in 34 South African hospitals. J Antimicrob Chemother. 2017;72:1227–34.
CAS PubMed Google Scholar
Shafiq N, Praveen Kumar M, Gautam V, Negi H, Roat R, Malhotra S, et al. Antibiotic stewardship in a tertiary care hospital of a developing country: establishment of a system and its application in a unit-GASP Initiative. Infection. 2016;44:651–9.
Bouza E, Muñoz P, Burillo A. Role of the clinical microbiology laboratory in antimicrobial stewardship. Med Clin North Am. 2018;102:883–98.
Vandenberg O, Kozlakidis Z, Schrenzel J, Struelens MJ, Breuer J. Control of infectious diseases in the era of European clinical microbiology laboratory consolidation: new challenges and opportunities for the patient and for public health surveillance. Front Med (Lausanne). 2018;5:15.
Croxatto A, Prod’hom G, Faverjon F, Rochais Y, Greub G. Laboratory automation in clinical bacteriology: what system to choose? Clin Microbiol Infect. 2016;22:217–35.
Cantón R, Horcajada JP, Oliver A, Garbajosa PR, Vila J. Inappropriate use of antibiotics in hospitals: the complex relationship between antibiotic use and antimicrobial resistance. Enferm Infecc Microbiol Clin. 2013;31(Suppl 4):3–11.
Tosh PK, McDonald LC. Infection control in the multidrug-resistant era: tending the human microbiome. Clin Infect Dis. 2012;54:707–13.
Tomczyk S, Zanichelli V, Grayson ML, Twyman A, Abbas M, Pires D, et al. Control of carbapenem-resistant Enterobacteriaceae, Acinetobacter baumannii, and Pseudomonas aeruginosa in healthcare facilities: a systematic review and reanalysis of quasi-experimental studies. Clin Infect Dis. 2019;68:873–84.
Waters CD. Pharmacist-driven antimicrobial stewardship program in an institution without infectious diseases physician support. Am J Health Syst Pharm. 2015;72:466–8.
von Gunten V, Reymond J-P, Beney J. Clinical and economic outcomes of pharmaceutical services related to antibiotic use: a literature review. Pharm World Sci. 2007;29:146–63.
Dunagan WC, Woodward RS, Medoff G, Gray JL 3rd, Casabar E, Smith MD, et al. Antimicrobial misuse in patients with positive blood cultures. Am J Med. 1989;87:253–9.
Fridkin SK, Steward CD, Edwards JR, Pryor ER, McGowan JE Jr, Archibald LK, et al. Surveillance of antimicrobial use and antimicrobial resistance in United States hospitals: project ICARE phase 2. Project Intensive Care Antimicrobial Resistance Epidemiology (ICARE) hospitals. Clin Infect Dis. 1999;29:245–52.
WHO Collaborating Centre for Drug Statistics Methodology. In: Guidelines for ATC classification and DDD assignment, 2013.2012. https://www.whocc.no/filearchive/publications/1_2013guidelines.pdf . Accessed 23 Oct 2019.
Morris AM. Antimicrobial Stewardship Programs: appropriate measures and metrics to study their impact. Curr Treat Options Infect Dis. 2014;6:101–12.
Burnett E. Effective infection prevention and control: the nurse’s role. Nurs Stand. 2018;33:68–72.
Bowater RJ, Stirling SA, Lilford RJ. Is antibiotic prophylaxis in surgery a generally effective intervention? Testing a generic hypothesis over a set of meta-analyses. Ann Surg. 2009;249:551–6.
Sinha B, Van Assen S, Friedrich AW. Important issues for perioperative systemic antimicrobial prophylaxis in surgery. Curr Opin Anaesthesiol. 2014;27:377–81.
Knox MC, Edye M. Educational antimicrobial stewardship intervention ineffective in changing surgical prophylactic antibiotic prescribing. Surg Infect (Larchmt). 2016;17:224–8.
Ozgun H, Ertugrul BM, Soyder A, Ozturk B, Aydemir M. Peri-operative antibiotic prophylaxis: adherence to guidelines and effects of educational intervention. Int J Surg. 2010;8:159–63.
Ansari F, Erntell M, Goossens H, Davey P. The European surveillance of antimicrobial consumption (ESAC) point-prevalence survey of antibacterial use in 20 European hospitals in 2006. Clin Infect Dis. 2009;49:1496–504.
Robert J, Pean Y, Varon E, Bru JP, Bedos JP, Bertrand X, et al. Point prevalence survey of antibiotic use in French hospitals in 2009. J Antimicrob Chemother. 2012;67:1020–6.
Slimings C, Riley TV. Antibiotics and hospital-acquired Clostridium difficile infection: update of systematic review and meta-analysis. J Antimicrob Chemother. 2014;69:881–91.
Owens RC Jr, Donskey CJ, Gaynes RP, Loo VG, Muto CA. Antimicrobial-associated risk factors for Clostridium difficile infection. Clin Infect Dis. 2008;46:S19–31.
Enzler MJ, Berbari E, Osmon DR. Antimicrobial prophylaxis in adults. Mayo Clin Proc. 2011;86:686–701.
Article CAS PubMed PubMed Central Google Scholar
Bratzler DW, Dellinger EP, Olsen KM, Perl TM, Auwaerter PG, Bolon MK, et al. Clinical practice guidelines for antimicrobial prophylaxis in surgery. Am J Health Syst Pharm. 2013;70:195–283.
A global declaration on appropriate use of antimicrobial agents across the surgical pathway. Surg Infect (Larchmt). 2017;18:846–53.
Huh K, Chung DR, Park HJ, Kim MJ, Lee NY, Ha YE, et al. Impact of monitoring surgical prophylactic antibiotics and a computerized decision support system on antimicrobial use and antimicrobial resistance. Am J Infect Control. 2016;44:e145–52.
Van Kasteren ME, Mannien J, Kullberg BJ, de Boer AS, Nagelkerke NJ, Ridderhof M, et al. Quality improvement of surgical prophylaxis in Dutch hospitals: evaluation of a multi-site intervention by time series analysis. J Antimicrob Chemother. 2005;56:1094–102.
Montravers P, Augustin P, Grall N, Desmard M, Allou N, Marmuse JP, et al. Characteristics and outcomes of anti-infective de-escalation during health care-associated intra-abdominal infections. Crit Care. 2016;20:83.
Tabah A, Cotta MO, Garnacho-Montero J, Schouten J, Roberts JA, Lipman J, et al. A systematic review of the definitions, determinants, and clinical outcomes of antimicrobial de-escalation in the intensive care unit. Clin Infect Dis. 2016;62:1009–17.
Sartelli M, Weber DG, Ruppé E, Bassetti M, Wright BJ, Ansaloni L, et al. Antimicrobials: a global alliance for optimizing their rational use in intra-abdominal infections (AGORA). World J Emerg Surg. 2016;11:33.
Swenson BR, Metzger R, Hedrick TL, McElearney ST, Evans HL, Smith RL, et al. Choosing antibiotics for intra-abdominal infections: what do we mean by “high risk”? Surg Infect (Larchmt). 2009;10:29–39.
Sartelli M, Catena F, Abu-Zidan FM, Ansaloni L, Biffl WL, Boermeester MA, et al. Management of intra-abdominal infections: recommendations by the WSES 2016 consensus conference. World J Emerg Surg. 2017;12:22.
Shani V, Muchtar E, Kariv G, Robenshtok E, Leibovici L. Systematic review and meta-analysis of the efficacy of appropriate empiric antibiotic therapy for sepsis. Antimicrob Agents Chemother. 2010;54:4851–63.
Ferrer R, Artigas A, Suarez D, Palencia E, Levy MM, Arenzana A, et al. Effectiveness of treatments for severe sepsis: a prospective, multicenter, observational study. Am J Respir Crit Care Med. 2009;180:861–6.
Castellanos-Ortega A, Suberviola B, García-Astudillo LA, Holanda MS, Ortiz F, Llorca J, et al. Impact of the Surviving Sepsis Campaign protocols on hospital length of stay and mortality in septic shock patients: results of a three-year follow-up quasi-experimental study. Crit Care Med. 2010;38:1036–43.
Puskarich MA, Trzeciak S, Shapiro NI, Arnold RC, Horton JM, Studnek JR, et al. Association between timing of antibiotic administration and mortality from septic shock in patients treated with a quantitative resuscitation protocol. Crit Care Med. 2011;39:2066–71.
Dellinger RP, Levy MM, Rhodes A, Annane D, Gerlach H, Opal SM, et al. Surviving Sepsis Campaign: international guidelines for management of severe sepsis and septic shock, 2012. Intensive Care Med. 2013;39:165–228.
Rhodes A, Evans LE, Alhazzani W, Levy MM, Antonelli M, Ferrer R, et al. Surviving sepsis campaign: international guidelines for management of sepsis and septic shock: 2016. Intensive Care Med. 2017;43:304–77.
Andersen BR, Kallehave FL, Andersen HK. Antibiotics versus placebo for prevention of postoperative infection after appendicectomy. Cochrane Database Syst Rev. 2005;3:CD001439.
Mazeh H, Mizrahi I, Dior U, Simanovsky N, Shapiro M, Freund HR, et al. Role of antibiotic therapy in mild acute calculus cholecystitis: a prospective randomized controlled trial. World J Surg. 2012;36:1750–9.
Regimbeau JM, Fuks D, Pautrat K, Mauvais F, Haccart V, Msika S, et al. Effect of postoperative antibiotic administration on postoperative infection following cholecystectomy for acute calculous cholecystitis: a randomized clinical trial. JAMA. 2014;312:145–54.
Sawyer RG, Claridge JA, Nathens AB, Rotstein OD, Duane TM, Evans HL, et al. Trial of short-course antimicrobial therapy for intraabdominal infection. N Engl J Med. 2015;372:1996–2005.
Leleu G, Aegerter P. Guidet B; Collège des Utilisateurs de Base de Donnéesen Réanimation. Systemic candidiasis in intensive care units: a multicenter, matched-cohort study. J Crit Care. 2002;17:168–75.
Arendrup MC, Sulims S, Holm A, Nielsen L, Nielsen SD, Knudsen JD, et al. Diagnostic issues, clinical characteristics, and outcomes for patients with fungemia. J Clin Microbiol. 2011;49:3300–8.
Zaoutis TE, Argon J, Chu J, Berlin JA, Walsh TJ, Feudtner C. The epidemiology and attributable outcomes of candidemia in adults and children hospitalized in the United States: a propensity analysis. Clin Infect Dis. 2005;41:1232–9.
Dean DA, Burchard KW. Surgical perspective on invasive Candida infections. World J Surg. 1998;22:127–34.
León C, Ruiz-Santana S, Saavedra P, Almirante B, Nolla-Salas J, Alvarez-Lerma F, et al. A bedside scoring system (Candida score) for early antifungal treatment in non-neutropenic critically ill patients with Candida colonization. Crit Care Med. 2006;34:730–7.
Scudeller L, Viscoli C, Menichetti F, del Bono V, Cristini F, Tascini C, et al. An Italian consensus for invasive candidiasis management (ITALIC). Infection. 2014;42:263–79.
Mikulska M, Calandra T, Sanguinetti M, Poulain D, Viscoli C. The use of mannan antigen and anti-mannan antibodies in the diagnosis of invasive candidiasis: recommendations from the Third European Conference on Infections in Leukemia. Crit Care. 2010;14:R222.
Karageorgopoulos DE, Vouloumanou EK, Ntziora F, Michalopoulos A, Rafailidis PI, Falagas ME. β- d -Glucan assay for the diagnosis of invasive fungal infections: a meta-analysis. Clin Infect Dis. 2011;52:750–70.
Posteraro B, Tumbarello M, De Pascale G, Liberto E, Vallecoccia MS, De Carolis E, et al. (1,3)-β- d -Glucan-based antifungal treatment in critically ill adults at high risk of candidaemia: an observational study. J Antimicrob Chemother. 2016;71:2262-2269.
Article CAS Google Scholar
Nucci M, Anaissie E, Betts RF, Dupont BF, Wu C, Buell DN, et al. Early removal of central venous catheter in patients with candidemia does not improve outcome: analysis of 842 patients from 2 randomized clinical trials. Clin Infect Dis. 2010;51:295–303.
Ben-Ami R. Treatment of invasive candidiasis: a narrative review. J Fungi (Basel). 2018;4(3).
Article CAS PubMed Central Google Scholar
Raman G, Avendano E, Berger S, Menon V. Appropriate initial antibiotic therapy in hospitalized patients with gram-negative infections: systematic review and meta-analysis. BMC Infect Dis. 2015;15:395.
De Waele JJ, Dhaese S. Antibiotic stewardship in sepsis management: toward a balanced use of antibiotics for the severely ill patient. Expert Rev Anti Infect Ther. 2019;17:89–97.
Timsit JF, Bassetti M, Cremer O, Daikos G, de Waele J, Kallil A, et al. Rationalizing antimicrobial therapy in the ICU: a narrative review. Intensive Care Med. 2019;45:172–89.
Neeser O, Branche A, Mueller B, Schuetz P. How to: implement procalcitonin testing in my practice. Clin Microbiol Infect. 2019;25:1226–30.
Watkins RR, Van Duin D. Current trends in the treatment of pneumonia due to multidrug-resistant Gram-negative bacteria. Version 2. F1000Res. 2019 Jan 30 [revised 2019 Jan 1];8.
Montero M, Sala M, Riu M, Belvis F, Salvado M, Grau S, et al. Risk factors for multidrug-resistant Pseudomonas aeruginosa acquisition. Impact of antibiotic use in a double case-control study. Eur J Clin Microbiol Infect Dis. 2010;29:335–9.
Muilwijk J, van den Hof S, Wille JC. Associations between surgical site infection risk and hospital operation volume and surgeon operation volume among hospitals in the Dutch nosocomial infection surveillance network. Infect Control Hosp Epidemiol. 2007;28:557–63.
Beldi G, Bisch-Knaden S, Banz V, Mühlemann K, Candinas D. Impact of intraoperative behavior on surgical site infections. Am J Surg. 2009;198:157–62.
Çakmakçi M. Antibiotic stewardship programmes and the surgeon’s role. J Hosp Infect. 2015;89:264–6.
Duane TM, Zuo JX, Wolfe LG, Bearman G, Edmond MB, Lee K, et al. Surgeons do not listen: evaluation of compliance with antimicrobial stewardship program recommendations. Am Surg. 2013;79:1269–72.
PubMed Google Scholar
Sartelli M, Labricciosa FM, Scoccia L, Bellesi J, Mazzoccanti MR, Scaloni G, et al. Non-restrictive antimicrobial stewardship program in a general and emergency surgery unit. Surg Infect (Larchmt). 2016;17:485–90.
Marshall JC. Principles of source control in the early management of sepsis. Curr Infect Dis Rev. 2010;12:345–53.
Marshall JC. al Naqbi A. Principles of source control in the management of sepsis. Crit Care Clin. 2009;25:753–68.
Jaffe TA, Nelson RC, DeLong D, Paulson EK. Practice patterns in percutaneous image-guided intra-abdominal abscess drainage: survey of academic and private practice centres. Radiology. 2004;233:750–6.
Sartelli M. A focus on intra-abdominal infections. World J Emerg Surg. 2010;5:9.
Van Ruler O, Mahler CW, Boer KR, Reuland EA, Gooszen HG, Opmeer BC, et al. Comparison of on-demand vs planned relaparotomy strategy in patients with severe peritonitis: a randomized trial. JAMA. 2007;298:865–72.
Sartelli M, Abu-Zidan FM, Ansaloni L, Bala M, Beltrán MA, Biffl WL, et al. The role of the open abdomen procedure in managing severe abdominal sepsis: WSES position paper. World J Emerg Surg. 2015;10:35.
Póvoa P, Salluh JIF. Biomarker-guided antibiotic therapy in adult critically ill patients: a critical review. Ann Intensive Care. 2012;2:32.
Cho S-Y, Choi J-HJ. Biomarkers of sepsis. Infect Chemother. 2014;46:1–12.
Nora D, Salluh J, Martin-Loeches I, Póvoa P. Biomarker-guided antibiotic therapy-strengths and limitations. Ann Transl Med. 2017;5:208.
Cals JW, Butler CC, Hopstaken RM, Hood K, Dinant GJ. Effect of point of care testing for C reactive protein and training in communication skills on antibiotic use in lower respiratory tract infections: cluster randomized trial. BMJ. 2009;338:b1374.
Aabenhus R, Jensen JU, Jørgensen KJ, Hróbjartsson A, Bjerrum L. Biomarkers as point-of-care tests to guide prescription of antibiotics in patients with acute respiratory infections in primary care. Cochrane Database Syst Rev. 2014;11:CD010130.
Gurleyik E, Gurleyik G, Unalmiser S. Accuracy of serum C-reactive protein measurements in diagnosis of acute appendicitis compared with surgeon’s clinical impression. Dis Colon Rectum. 1995;38:1270–4.
Shakhatreh HS. The accuracy of C-reactive protein in the diagnosis of acute appendicitis compared with that of clinical diagnosis. Med Arh. 2000;54:109–10.
Asfar S, Safar H, Khoursheed M, Dashti H, al-Bader A. Would measurement of C-reactive protein reduce the rate of negative exploration for acute appendicitis? J R Coll Surg Edinb. 2000;45:21-24.
Annane D, Maxime V, Faller JP, Mezher C, Clec’h C, Martel P, et al. Procalcitonin levels to guide antibiotic therapy in adults with non-microbiologically proven apparent severe sepsis: a randomised controlled trial. BMJ Open. 2013;3:112–5.
Bloos F, Trips E, Nierhaus A, Briegel J, Heyland DK, Jaschinski U, et al. Effect of sodium selenite administration and procalcitonin-guided therapy on mortality in patients with severe sepsis or septic shock: a randomized clinical trial. JAMA Intern Med. 2016;176:1266–76.
Bouadma L, Luyt CE, Tubach F, Cracco C, Alvarez A, Schwebel C, et al. Use of procalcitonin to reduce patients’ exposure to antibiotics in intensive care units (PRORATA trial): a multicenter randomised controlled trial. Lancet. 2010;375:463–74.
de Jong E, van Oers JA, Beishuizen A, Vos P, Vermeijden WJ, Haas LE, et al. Efficacy and safety of procalcitonin guidance in reducing the duration of antibiotic treatment in critically ill patients: a randomised, controlled, open-label trial. Lancet Infect Dis. 2016;16:819–27.
Article PubMed CAS Google Scholar
Deliberato RO, Marra AR, Sanches PR, Martino MD, Ferreira CE, Pasternak J, et al. Clinical and economic impact of procalcitonin to shorten antimicrobial therapy in septic patients with proven bacterial infection in an intensive care setting. Diagn Microbiol Infect Dis. 2013;76:266–71.
Hochreiter M, Schroeder S. Procalcitonin-based algorithm. Management of antibiotic therapy in critically ill patients. Anaesthesist. 2011;60:661–73.
Schroeder S, Hochreiter M, Koehler T, Schweiger AM, Bein B, Keck FS, von Spiegel T. Procalcitonin (PCT)-guided algorithm reduces length of antibiotic treatment in surgical intensive care patients with severe sepsis: results of a prospective randomized study. Langenbecks Arch Surg. 2009;394(2):221–6.
Hochreiter M, Kohler T, Schweiger AM, Keck FS, Bein B, von Spiegel T, et al. Procalcitonin to guide duration of antibiotic therapy in intensive care patients: a randomized prospective controlled trial. Crit Care. 2009;13:R83.
Layios N, Lambermont B, Canivet JL, Morimont P, Preiser JC, Garweg C, et al. Procalcitonin usefulness for the initiation of antibiotic treatment in intensive care unit patients. Crit Care Med. 2012;40:2304–9.
Oliveira CF, Botoni FA, Oliveira CR, Silva CB, Pereira HA, Serufo JC, et al. Procalcitonin versus C-reactive protein for guiding antibiotic therapy in sepsis: a randomized trial. Crit Care Med. 2013;41:2336–43.
Nobre V, Harbarth S, Graf JD, Rohner P, Pugin J. Use of procalcitonin to shorten antibiotic treatment duration in septic patients: a randomized trial. Am J Respir Crit Care Med. 2008;177:498–505.
Maseda E, Suarez-de-la-Rica A, Anillo V, Tamayo E, García-Bernedo CA, Ramasco F, et al. Procalcitonin-guided therapy may reduce length of antibiotic treatment in intensive care unit patients with secondary peritonitis: a multicenter retrospective study. J Crit Care. 2015;30:537–42.
Huang TS, Huang SS, Shyu YC, Lee CH, Jwo SC, Chen PJ, et al. A procalcitonin-based algorithm to guide antibiotic therapy in secondary peritonitis following emergency surgery: a prospective study with propensity score matching analysis. PLoS One. 2014;9:e90539.
Mueck KM, Kao LS. Patients at high-risk for surgical site infection. Surg Infect (Larchmt). 2017;18:440–6.
Florschutz AV, Fagan RP, Matar WY, Sawyer RG, Berrios-Torres SI. Surgical siteinfection risk factors and risk stratification. J Am Acad Orthop Surg. 2015;23(Suppl):S8–S11.
Bergquist JR, Thiels CA, Etzioni DA, Habermann EB, Cima RR. Failure of colorectal surgical site infection predictive models applied to an independent dataset: do they add value or just confusion? J Am Coll Surg. 2016;222:431–8.
Dumville JC, Gray TA, Walter CJ, Sharp CA, Page T. Dressings for the prevention of surgical site infection. Cochrane Database Syst Rev. 2014;9:CD003091.
Dumville JC, Gray TA, Walter CJ, Sharp CA, Page T, Macefield R, et al. Dressings for the prevention of surgical site infection. Cochrane Database Syst Rev. 2016;12:CD003091.
Owens P, McHugh S, Clarke-Moloney M, Healy D, Fitzpatrick F, McCormick P, et al. Improving surgical site infection prevention practices through a multifaceted educational intervention. Ir Med J. 2015;108:78–81.
Department of Health, London. In: High impact intervention. Care bundle to prevent surgical site infection. 2011. http://webarchive.nationalarchives.gov.uk/20120118164404/hcai.dh.gov.uk/files/2011/03/2011-03-14-HII-Prevent-Surgical-Site-infection-FINAL.pdf . Accessed 23 Oct 2019.
Download references
Acknowledgements
Author information, authors and affiliations.
Department of Surgery, Macerata Hospital, ASUR Marche, Macerata, Italy
Massimo Sartelli, Guido Cesare Gesuelli, Walter Siquini & Cristian Tranà
Infectious Diseases Unit, Bolzano Central Hospital, Bolzano, Italy
Leonardo Pagani & Raffaella Binazzi
Ministry of Health, Rome, Italy
Stefania Iannazzo
Regional Agency for Health and Social Care, Emilia-Romagna Region–ASSR, Bologna, Italy
Maria Luisa Moro & Enrico Ricchizzi
Department of Medical and Surgical Sciences, Clinics of Infectious Diseases, S. Orsola-Malpighi Hospital, “Alma Mater Studiorum”-University of Bologna, Bologna, Italy
Pierluigi Viale & Fabio Tumietto
Infectious Diseases, ASST di Cremona, Cremona, Italy
General, Emergency and Trauma Surgery Department, Bufalini Hospital, Cesena, Italy
Luca Ansaloni
Emergency Surgery Unit, New Santa Chiara Hospital, University of Pisa, Pisa, Italy
Federico Coccolini
Department of Biomedical Sciences and Public Health, Marche Polytechnic University, Ancona, Italy
Marcello Mario D’Errico
Bone Marrow Transplant Unit, Denis Burkitt, St. James’s Hospital, Dublin, Ireland
Iris Agreiter
Infectious Diseases Unit, Fermo Hospital, ASUR Marche, Marche, Italy
Giorgio Amadio Nespola
Infectious Diseases Unit, Azienda Ospedaliera Ospedali Riuniti Marche Nord, Pesaro, Italy
Francesco Barchiesi
Clinical Administration, Senigallia Hospital, ASUR Marche, Senigallia, AN, Italy
Valeria Benigni
Infectious Diseases Clinic, Department of Medicine, “S. Maria” Hospital, Terni, University of Perugia, Perugia, Italy
Stefano Cappanera
Infectious Diseases Unit, Macerata Hospital, ASUR Marche, Macerata, Italy
Alessandro Chiodera
Department of Hospital Pharmacy, Ospedali Riuniti di Ancona, Ancona, Italy
Valentina Cola
Department of Anesthesiology and Intensive Care Unit, Civitanova Marche Hospital, ASUR Marche, Civitanova Marche, MC, Italy
Daniela Corsi
Emergency Surgery and Trauma Care Unit, San Filippo Neri Hospital, Rome, Italy
Francesco Cortese
Infectious Diseases Unit, Pordenone Hospital, Pordenone, Friuli-Venezia Giulia, Italy
Massimo Crapis
Infectious Diseases Unit, Rimini Hospital, Rimini, Italy
Francesco Cristini
Hospital Pharmacy Unit, Santa Maria della Misericordia Hospital, Azienda Ospedaliera di Perugia, Perugia, Italy
Alessandro D’Arpino
Operative Unit of General Surgery, Azienda USL IRCCS Reggio Emilia, Reggio Emilia, Italy
Belinda De Simone
Infectious Diseases Department, Trieste University Hospital, Trieste, Italy
Stefano Di Bella
Department of Surgery, Versilia Hospital, Lido di Camaiore, Italy
Francesco Di Marzo
Department of Anesthesiology and Intensive Care Unit, Department of Biomedical Sciences and Public Health, Università Politecnica delle Marche, Ancona, Italy
Abele Donati
Department of Anesthesiology and Intensive Care Unit, Macerata Hospital, ASUR Marche, Macerata, Italy
Daniele Elisei
Department of Infectious Diseases, Fondazione Policlinico A. Gemelli IRCCS, Istituto di Clinica delle Malattie Infettive, Università Cattolica S. Cuore, Rome, Italy
Massimo Fantoni & Rita Murri
Department of Critical Care Medicine Unit, San Filippo Neri Hospital, Rome, Italy
Anna Ferrari
Department of Surgery, Azienda Ospedaliera Ospedali Riuniti Marche Nord, Pesaro, Italy
Domitilla Foghetti
Infectious Diseases Clinic, University of Perugia, Perugia, Italy
Daniela Francisci
Infectious Diseases Unit, Carlo Poma Hospital, Mantua, Italy
Gianni Gattuso
Infectious Diseases Clinic, Department of Biological Sciences and Public Health, Marche Polytechnic University, Ancona, Italy
Andrea Giacometti
Department of Surgery, Marche Polytechnic University of Marche Region, Ancona, Italy
Cristina Marmorale
Hospital Hygiene Unit, Azienda Ospedaliero-Universitaria Ospedali Riuniti, Ancona, Italy
Enrica Martini
Unit of Microbiology and Virology, San Filippo Neri Hospital, Rome, Italy
Marcello Meledandri
Clinical Administration Santa Maria Annunziata Hospital, USL Toscana Centro, Florence, Italy
Daniela Padrini
Epidemiology Office, AUSL Pescara, Pescara, Italy
Dalia Palmieri
Unit of Microbiology and Virology, Senigallia Hospital, Senigallia, AN, Italy
Paola Pauri
Surgical Unit, Savona Hospital, Savona, Italy
Carla Rebagliati
Department of Experimental, Diagnostic and Specialty Medicine (DIMES), University of Bologna, Bologna, Italy
Vittorio Sambri
Unit of Microbiology, The Great Romagna Area Hub Laboratory, Pievesestina, Cesena, Italy
Department of Internal Medicine, Jesi Hospital, Ancona, Italy
Anna Maria Schimizzi
Unit of Hospital Pharmacy, Macerata Hospital, ASUR Marche, Macerata, Italy
Loredana Scoccia
Infectious Diseases Unit, Fondazione Policlinico Universitario A. Gemelli IRCCS, Rome, Italy
Giancarlo Scoppettuolo
Division of Emergency Surgery, Fondazione Policlinico Universitario A. Gemelli IRCCS, Università Cattolica del Sacro Cuore, Rome, Italy
Gabriele Sganga
General Directory, ASUR Marche, Ancona, Italy
Nadia Storti
Infectious Diseases Unit, Azienda Ospedaliero Universitaria Ospedali Riuniti, Ancona, Italy
Marcello Tavio
Clinical Risk Management and Patient Safety Center, Tuscany Region, Florence, Italy
Giulio Toccafondi
Department of Anesthesiology, Neuro Intensive Care Unit, Florence Careggi University Hospital, Florence, Italy
Bruno Viaggi
Unit of Hepato-Pancreato-Biliary and Transplant Surgery, Department of Experimental and Clinical Medicine, Polytechnic University of Marche, Ancona, Italy
Marco Vivarelli
Health First Europe, Brussels, Belgium
Melina Raso
Global Alliance for Infections in Surgery, Porto, Portugal
Francesco Maria Labricciosa
Faculty of Medical Sciences, School of Pharmacy, The University of the West Indies, St. Augustine, Trinidad and Tobago
Sameer Dhingra
Emergency Surgery Department, Parma University Hospital, Parma, Italy
Fausto Catena
You can also search for this author in PubMed Google Scholar
Contributions
MS wrote the first draft of the manuscript. SD performed the first review of the manuscript. All the authors reviewed and approved the final manuscript.
Corresponding author
Correspondence to Massimo Sartelli .
Ethics declarations
Ethics approval and consent to participate.
The paper does not have any information that can identify any patient. There are no clinical images.
Consent for publication
Competing interests.
The authors declare that they have no competing interests.
Additional information
Publisher’s note.
Springer Nature remains neutral with regard to jurisdictional claims in published maps and institutional affiliations.
Statements approved with an agreement ≥80%
Statement 2.1 . Surveillance of HAIs improves the quality of care because it reduces the risk of infection. It should be supported by all healthcare workers.
Statement 4.1. Key points forCDI prevention are:
Question 8. Antimicrobial stewardship. Is a multidisciplinary approach necessary?
Statement 13.1. It is important the knowledge of the risk of developing invasive candidiasis, improve microbiological diagnostics and optimize treatment.
Statement 15.1. To be a champion in preventing and managing infections in surgery means to create a culture of collaboration in which infection prevention, antimicrobial stewardship and correctsurgical approach are of high importance.
Statement 20.2. Surgeons with satisfactory knowledge in surgical infections should be involved in the infection control team and recognized as “champions” by the hospital’s administration.
Rights and permissions
Open Access This article is distributed under the terms of the Creative Commons Attribution 4.0 International License ( http://creativecommons.org/licenses/by/4.0/ ), which permits unrestricted use, distribution, and reproduction in any medium, provided you give appropriate credit to the original author(s) and the source, provide a link to the Creative Commons license, and indicate if changes were made. The Creative Commons Public Domain Dedication waiver ( http://creativecommons.org/publicdomain/zero/1.0/ ) applies to the data made available in this article, unless otherwise stated.
Reprints and permissions
About this article
Cite this article.
Sartelli, M., Pagani, L., Iannazzo, S. et al. A proposal for a comprehensive approach to infections across the surgical pathway. World J Emerg Surg 15 , 13 (2020). https://doi.org/10.1186/s13017-020-00295-3
Download citation
Received : 03 December 2019
Accepted : 10 February 2020
Published : 18 February 2020
DOI : https://doi.org/10.1186/s13017-020-00295-3
Share this article
Anyone you share the following link with will be able to read this content:
Sorry, a shareable link is not currently available for this article.
Provided by the Springer Nature SharedIt content-sharing initiative
- Antimicrobial stewardship
- Healthcare-associated infections
- Infection control
- Multidrug-resistant organisms
- Preoperative antibiotic prophylaxis
- Surgical site infections
World Journal of Emergency Surgery
ISSN: 1749-7922
- Submission enquiries: [email protected]
- Open access
- Published: 01 September 2023
Nurses’ knowledge on nosocomial infections preventive measures and its associated factors in Ghana: a cross-sectional study
- Samuel Salu 1 ,
- Joshua Okyere 2 , 3 ,
- Veronica Okwuchi Charles-Unadike 4 &
- Mark Kwame Ananga 4
BMC Health Services Research volume 23 , Article number: 941 ( 2023 ) Cite this article
4728 Accesses
Metrics details
Nosocomial infections (NCIs) have been associated with several adverse outcomes including extended hospitalization, persistent disability, heightened antimicrobial resistance, amplified socio-economic disruption, and elevated mortality rates. The adoption of infection prevention strategies has the greatest tendency to significantly reduce the risk and occurrence of NCIs among the population, particularly in resource constrained health systems. This study assessed nurses’ knowledge on NCI preventive measures and its associated factors in Ghana.
A cross-sectional study was conducted from July to August 2021. A sample of 237 healthcare workers in the Hohoe Municipality was selected to participate in the study. Data was collected with a questionnaire designed in Google Forms and analyzed using Stata version 16.0.
Overall, most of the participants (69.2%) were not knowledgeable about the preventive measures of NCIs. Nurses who were within the age group of 20–40 years [aOR = 0.25 (95% CI = 0.09–0.69), p = 0.007] and 41–60 years [aOR = 0.05 (95% CI = 0.01–0.29), p = 0.001] were significantly less likely to be knowledgeable about the preventive measures of NCIs compared to those who those aged less than 20 years. Nurses who attended in-service training or workshop were approximately 10 times more likely to be knowledgeable about preventive measures of nosocomial infection compared to those who had never attended in-service training or workshop [aOR = 9.55 (95% CI = 1.23–74.36), p = 0.031].
The study concludes that age and participation in-service training or workshop are significant factors that influence the knowledge of healthcare workers in preventive measures for nosocomial infections. These results highlight the importance of providing ongoing training and professional development opportunities to nurses to enhance their knowledge and improve their ability to prevent and control nosocomial infections. Additionally, the study emphasizes the need for targeted training programs that consider the age of nurses, to ensure that training is tailored to their specific needs.
Peer Review reports
Globally, infections are considered a serious public health concern [ 1 ]. While infections occur in different settings including at home, work and in outdoor settings, infections acquired at the healthcare facility pose a significant threat to the overall quality of healthcare delivery. According to the World Health Organisation [ 2 ], nosocomial infections (NCIs) or hospital-acquired infections (HAIs) refer to “an infection occurring in a patient in a hospital or other health care facility in whom the infection was not present or incubating at the time of admission. This includes infections acquired in the hospital but appearing after discharge, and also occupational infections among staff of the facility” . These infections include urinary tract infections, surgical site infections (e.g., Staphylococcus aureus), bloodstream infections, and lower respiratory tract infections [ 3 ].
There are approximately 1.7 million patients worldwide who contract NCIs each year [ 4 ]. A systematic review [ 5 ] has also estimated NCIs to be increasing worldwide with an annual increasing rate of 0.06, and with the African region having the highest rates of NCIs. Ghana, for instance, has an estimated NCI prevalence of 8.2% [ 6 ]. If left unabated, the existence of NCIs would have serious repercussions for health care delivery, time spent at the hospital, and healthcare expenditure. NCIs have been associated with several adverse outcomes including extended hospitalization, persistent disability, heightened antimicrobial resistance, amplified socio-economic disruption, and elevated mortality rates [ 6 , 7 , 8 ]. However, the adoption of infection prevention strategies has the greatest tendency to significantly reduce the risk and occurrence of NCI among the population, particularly in resource constrained health systems [ 9 ].
Available evidence suggests that adopting NCI prevention involves establishing a protective barrier between vulnerable host and microorganisms [ 10 ]. According to the WHO [ 2 ], NCI can primarily be prevented either by reducing person-to-person transmission or by preventing transmission from the environment. Reducing person-to-person transmission involves implementing various measures to minimize the spread of infections between patients, healthcare workers, and visitors. This includes promoting proper hand hygiene practices (i.e., handwashing or using hand sanitizers), and practicing safe injection practices [ 11 ]. On the other hand, preventing transmission from the environment involves maintaining a clean and hygienic healthcare setting. It encapsulates practices such as sterilization, disinfection of patient equipment, proper waste management and cleaning of the hospital environment [ 2 ].
In Ghana, the Ministry of Health and Ghana Health Service has emphasized the importance of adopting preventive measures to control the burden of NCIs [ 6 ]. This keen interest in NCI prevention in Ghana is evident in the country’s implementation of a national infection prevention policy and guidelines for healthcare settings [ 12 ]. However, it must be noted that having the support of the government agencies and a policy framework is not enough for healthcare workers to implement NCI preventive measures. Their knowledge level is quintessential to the implementation of NCI preventive measures [ 13 ]. Nurses’ knowledge on NCI preventive measures is critical for successful implementation and compliance with infection control protocols. Moreover, adequate knowledge has the potential to empower nurses to identify potential risks, implement preventive measures effectively, and respond promptly to infection control challenges [ 14 ].
Limited research has been conducted in Ghana to assess the current state of nurses’ knowledge with respect to NCI prevention. While the existing body of literature has examined the extent to which knowledge influences the practice of NCI preventive measures [ 15 , 16 ], it fails to assess what factors predict the knowledge level of nurses regarding NCI prevention in Ghana. In terms of geographical boundaries, none of the existing studies have investigated the dynamics of nurses’ knowledge on NCI preventive measures in the Volta Region of Ghana. Understanding the level of knowledge and identifying factors associated with nurses’ knowledge gaps can inform targeted interventions and educational programs aimed at improving infection control practices. By addressing these gaps, healthcare facilities in Ghana can enhance their infection prevention and control efforts, leading to a reduction in the burden of NCI and improved patient outcomes. Hence, we aimed to assess nurses’ knowledge on nosocomial infection (NCI) preventive measures and its associated factors in Ghana.
Study design
A cross-sectional study design was employed in this study. This study was carried out in the Hohoe Municipality, in the Volta Region of Ghana. The Hohoe Municipality is one of the eighteen (18) districts in the Volta Region. The city of Hohoe, of which the district was named, serves as the capital and the administrative or local government centre. It shares borders with the Republic of Togo on the east; on the southeast by the Afadzato district and southwest by Kpando Municipality; on the north with Jasikan district; and on the northwest with the Biakoye districts. Its capital, Hohoe, is about 78 km from Ho, the regional capital and 220 km from Accra, the national capital. The Municipality consists of one hundred and two (102) communities with a population of 167,016 projected from the 2010 National Population Census.
Sample size and sampling procedure
Using Cochrane’s single proportion formula, a sample size of 215 was estimated as follows;
\(n=\frac{{z}^{2} p(1-p)}{{d}^{2}}\) , considering 5% margin of error, 95% confidence interval = 1.96 and a proportion knowledge of 83.21% from a study conducted by [ 17 ]. Where;
n = Estimated sample size.
p = 0.8321.
d = margin of error (0.05).
Z = Test Statistic (1.96).
Adding 10% to cater for non-response increased the estimated sample size to 237.
The Hohoe Municipal comprises of four (4) sub-districts and all these sub-districts have health facilities. A simple random sampling was used to select two health facilities from the four (4) sub-districts. This was done by writing the names of all the health facilities from the four (4) sub-districts on pieces of paper, folded for concealment and placed into a container. These papers were thoroughly mixed, and two neutral persons were asked to pick one piece of paper each from the container without opening. In each of the two facilities sampled, nurses who met the inclusion criteria and consented to participate in the study were conveniently selected.
Inclusion and exclusion criteria
Nurses were selected based on the following inclusion criteria: (1) they must be registered nurses currently working in any of the study sites, (2) they must be present at the time of the survey, and (3) they must express a voluntary interest in participating in the study. Therefore, student nurses and nursing interns were excluded from the study. Additionally, nurses who were on leave during the data collection period were excluded from the study.
Definition of variables
Outcome variable.
Knowledge on preventive measures of nosocomial infections was the study’s outcome variable. This was assessed using eleven (11) items on the questionnaire. These items assessed whether respondents (I) have heard about infection prevention (II) could tell if gloves provide complete protection against acquiring or transmitting infections (III) knows if washing hands with soap or an alcohol-based antiseptic decreases the risk of transmission of nosocomial infections (IV) knows if the use of an alcohol-based antiseptic for hand hygiene is as effective as soap and water if hands are not visibly dirty (V) knows if gloves should be worn if blood or body fluid exposure is anticipated or not (VI) knows if there is a need to wash hands before doing procedures that do not involve bodily fluids or not (VII) knows if there is a need to wear the same pair of gloves for multiple patients as long as there is no visible contamination or not (VIII) knows the specific waste disposal buckets according to the level of their contamination (VIV) knows the written formula for preparing 0.5% chlorine solution (X) knows how long instrument or equipment should be disinfected and (XI) knows disease that are transmitted by needle stick injury.
For each of the items (I-X), respondents were asked to choose from the two responses “yes” or “no” provided. For question (XI), respondents were provided with a list of diseases (HBV, HCV, TB, HIV) to choose from. Respondents were allowed to choose from the list multiple times. A composite knowledge score was obtained by assigning a score of 1 to all the positive responses to the eleven (11) questions. All negative responses on the other hand were assigned the score of 0. A mean score was generated by adding all these responses and nurses who scored below the mean were considered “not knowledgeable” on the preventive measures of nosocomial infections, whereas nurses who scored above the mean were considered “knowledgeable”. The study incorporated the classification of knowledge on the preventive measures of nosocomial infections into “not knowledgeable” and “knowledgeable” from previous studies [ 18 , 19 , 20 ].
Explanatory variable
For the study, five (5) explanatory variables were considered in our estimations. These variables included age, sex, level of education, years of working experience and in-service training or workshop. None of these variables were chosen at random; rather, they were chosen based on the findings of previous studies on knowledge in preventive measures of nosocomial infections among healthcare workers [ 21 , 22 , 23 ]. In assessing these socio-demographic information of the respondents, age and years of working experience were collected as continuous variables and categorized into (< 20 years, 20–40 years, 41–60 years) and (1–10 years, 11–20 years and > 20 years) respectively. Respondents’ sex (male or female), level of education (certificate, diploma, degree, masters or doctoral), and in-service training or workshop (yes or no) were collected as categorical variables.
Data collection
The study covered a period from July 2021 to August 2021. Nurses working in the Hohoe Municipality and whose consents are been sought during the time of the study were recruited to participate in the study. Data was collected using a well-structured questionnaire comprising of both open and close-ended questions which were pretested. The questionnaire was designed in Google Forms. It comprised of 35 items related to the socio-demographic factors, knowledge in preventive measures and practice of preventive measures of NCIs.
Data analysis
The data was extracted from Google Forms to Excel Sheet for cleaning and then exported into STATA V.16.0 (StataCorp. 2019. Stata Statistical Software: Release 16. College Station, TX: StataCorp LLC.) analysis. To ensure the quality of the data extracted, double entry was done to address discrepancies which may have occurred during data extraction. The data was extensively cleaned again in STATA V. 16.0 (StataCorp. 2019. Stata Statistical Software: Release 16. College Station, TX: StataCorp LLC.) before analysis was carried out. Descriptive statistics were performed to interpret the socio-demographic features including age, sex, level of education, work experience, and in-service training or workshop on nosocomial infection prevention. However, inferential statistics were done to test the association between socio-demographic factors and healthcare workers’ knowledge in preventive measures of nosocomial infections. Frequencies and percentages related to the study findings were presented using tables and graphs.
Table 1 provides a distribution of the socio-demographic characteristics of the respondents. The majority of the healthcare workers were females 124 (52.3%) while males were 113 (47.7%). More than two-thirds of them, 195 (82.7%) were within the age group of (20–40) years. Most of the participants, 127 (53.6%) were Diploma holders. Regarding their work experience, almost all of them 204 (86.1%) had work experience ranging between (1–10) years. Out of the total 237 healthcare workers, 218 (92.0%) had attended in-service training or workshop on nosocomial infection prevention while 19 (8.0%) had never attended any in-service training or workshop on nosocomial infection prevention.
Knowledge of nurses on preventive measures of nosocomial infections
Table 2 shows the distribution of nurses’ knowledge on NCI preventive measures. Out of the total 237 nurses who participated in the study, more than 90% 216 (91.1%) indicated that they had heard about infection prevention. More than half 148 (68.5%) out of the 216 (91.1%) who had heard about infection prevention believed that gloves cannot provide complete protection against acquiring or transmitting infections. Almost all of them 213 (98.6%) believed that washing your hands with soap or using an alcohol-based antiseptic decreases the risk of transmission of NCI. More than 80% of them 187 (86.6%) also indicated that the use of an alcohol-based antiseptic for hand hygiene is as effective as soap and water if hands are not visibly dirty. All of them 216 (100%) agreed that there is a need to wash hands before doing procedures that do not involve bodily fluids. Furthermore, 198 (83.5%) of the nurses know the specific waste disposal buckets according to the level of their contamination. Most of the participants, 210 (88.6%), indicated that instruments or equipment should be disinfected for 10 min.
A knowledge mean score was generated using the items used to measure the level of knowledge among the nurses. Those who scored below the mean were considered not knowledgeable on the preventive measures of NCIs, whereas those who scored above the mean were considered knowledgeable. The findings from this study revealed that 164 (69.2%) of the participants were not knowledgeable on the preventive measures of NCIs (Fig. 1 ).

Overall level of knowledge of healthcare workers in preventive measures of nosocomial infections
Factors associated with nurses’ knowledge on preventive measures of nosocomial infections
Age and in-service training or workshop were the only factors that were significantly associated with the participants’ knowledge on NCI preventive measures. Sex, level of education and years of working experience showed no significant association with nurses’ knowledge on NCI preventive measures. Specifically, nurses who were within the age group of 20–40 years [aOR = 0.25 (95% CI = 0.09–0.69), p = 0.007] and 41–60 years [aOR = 0.05 (95% CI = 0.01–0.29), p = 0.001] were significantly less likely to be knowledgeable about the preventive measures of NCIs compared to those who those aged less than 20 years. Nurses who attended in-service training or workshop were approximately 10 times more likely to be knowledgeable about preventive measures of nosocomial infection compared to those who had never attended in-service training or workshop [aOR = 9.55 (95% CI = 1.23–74.36), p = 0.031] (Table 3 ).
Recognizing the importance of NCI prevention to the healthcare system of Ghana [ 6 , 12 ], we assessed nurses’ knowledge on NCI preventive measures and its associated factors in Ghana. Our study revealed that more than two-thirds of nurses (69.2%) were not knowledgeable about NCI preventive measures. The observed proportion of nurses who were knowledge about NCI prevention measures is less when compared a previous study conducted in Ethiopia [ 10 ] where 90% of nurses had good knowledge on NCI preventive measures. Nevertheless, our findings align with a prior study conducted in Tamale, Ghana [ 21 ] which revealed that only 50% of nurses were knowledgeable about infection prevention measures, including NCIs. The observed low knowledge on NCI preventive measures among nurses poses a significant threat to patient safety. This is in the sense that patients often rely on nurses to provide safe and effective care. Therefore, having a low knowledge about NCI preventive measures implies that nurses may be involved in practices that exacerbate the risk of NCI transmission [ 13 , 15 ]. Our findings, thus, underscore a need for the Ministry of Health, Ghana Health Service and hospital administrators to intensify education and sensitization initiatives to improve nurses’ knowledge regarding NCI preventive measures.
The study revealed that having participated in an in-service training or workshop was positively associated with nurses’ knowledge on NCI preventive measures. That is, the likelihood of being knowledgeable about NCI preventive measures was significantly higher among those who had participated in an in-service training or workshop compared to those who had not participated in such initiatives. Similar findings have been reported in North-East Ethiopia [ 23 ] and Nigeria [ 25 ]. A plausible explanation for this result could be that in-service training and workshops serve as reinforcement mechanisms for existing knowledge. Even if nurses have received prior education on infection control, attending training sessions provides an opportunity to refresh their knowledge, identify areas for improvement, and correct any misconceptions or outdated practices. The repetition of key concepts and information during the training sessions has the potential to reinforce the importance of NCI prevention and increases retention of knowledge among nurses [ 25 ].
Another finding from this study was the significant association between age and nurses’ knowledge on NCI prevention. Contrary to previous studies that have shown that nurses’ knowledge on NCI prevention increases with increasing age [ 22 , 23 , 24 ], we found that older age was associated with lower odds of being knowledgeable about NCI prevention compared to those of younger age. That is, the present study challenges the existing literature that posits that older nurses tend to be more knowledgeable about NCI prevention through years of experience and working collaboratively with senior staff [ 22 ]. It is possible that younger nurses, who may have recently completed their education or training, are likely to have been exposed to more up-to-date information and guidelines regarding NCI prevention. They may have received more comprehensive training that includes the latest research, technological advancements, and evidence-based practices. In contrast, older nurses may not have had the same exposure to these updated resources, leading to a knowledge gap between the age groups. We also postulate that older nurses may be less inclined to adopt new practices or update their knowledge base, especially if they have been practicing for a long time without encountering significant issues related to NCI. This resistance to change can result in a slower uptake of new information and guidelines, hence, explaining their lower knowledge levels regarding NCI prevention.
Implications for policy and practice
The study highlights the urgent need for the Ministry of Health, Ghana Health Service, and hospital administrators to prioritize education and sensitization initiatives on NCI preventive measures for nurses. Also, the positive association between participation in in-service training or workshops and nurses’ knowledge on NCI preventive measures emphasizes the importance of these initiatives. Healthcare institutions should provide regular opportunities for nurses to attend such training sessions, as they serve as reinforcement mechanisms for existing knowledge and contribute to improved understanding and implementation of NCI prevention measures. To bridge the knowledge gap observed among older nurses, healthcare institutions should design and implement tailored training programs that specifically address their needs. These programs should focus on updating their knowledge base, addressing resistance to change, and providing them with the necessary skills to adopt current NCI preventive measures.
Strengths and limitations
The strength of this study lies in the use of appropriate methodology to estimate the sample and analyze the data. Nevertheless, there are some limitations that must be taken into consideration. As the study relied on a cross-sectional design, it is not possible to establish a causal pathway between the age and in-service training as determinants of nurses’ knowledge on preventive measure for NCIs. The quantitative approach to this study does not provide an in-depth insight into other underlying factors that might influence the observed associations. Therefore, there is a need for qualitative research to gain a more comprehensive and nuanced understanding of nurses’ knowledge on preventive measures for NCIs. Another limitation of this study is that it focused only on nurses. Hence, the findings may not reflect the current knowledge of other healthcare workers including general medical practitioners, surgeons, and laboratory technicians.
A significant proportion of nurses in Ghana lack knowledge on NCI prevention. The study concludes that age and participation in-service training or workshop are significant factors associated with nurses’ knowledge NCI prevention. These results highlight the importance of providing ongoing training and professional development opportunities to nurses to enhance their knowledge and improve their ability to prevent and control nosocomial infections. Additionally, the study emphasizes the need for targeted training programs that consider the age of nurses, to ensure that training is tailored to their specific needs and learning styles.
Data availability
All data generated or analyzed during this study are included in this published article.
Abbreviations
Adjusted odds ratio
Crude odds ratio
Hospital Acquired Infections
Human Immunodeficiency virus
Hepatitis B Virus
Hepatitis C Virus
Infection prevention and Control
Intensive Care Unit
- Nosocomial infection
Nosocomial infections
World Health Organization
Saleem Z, Godman B, Hassali MA, Hashmi FK, Azhar F, Rehman IU. Point prevalence surveys of health-care-associated infections: a systematic review. Pathogens and Global Health. 2019;113(4):191–205.
Article CAS PubMed PubMed Central Google Scholar
World Health Organization. Prevention of hospital-acquired infections: a practical guide. World Health Organization; 2002.
Jenkins DR. Nosocomial infections and infection control. Medicine. 2017;45(10):629–33.
Article Google Scholar
Haque M, Sartelli M, McKimm J, Bakar MA. Health care-associated infections–an overview. Infection and drug resistance. 2018 Nov 11:2321–33.
Raoofi S, Pashazadeh Kan F, Rafiei S, Hosseinipalangi Z, Noorani Mejareh Z, Khani S, Abdollahi B, Seyghalani Talab F, Sanaei M, Zarabi F, Dolati Y. Global prevalence of nosocomial infection: a systematic review and meta-analysis. PLoS ONE. 2023;18(1):e0274248.
Labi AK, Obeng-Nkrumah N, Owusu E, Bjerrum S, Bediako-Bowan A, Sunkwa-Mills G, Akufo C, Fenny AP, Opintan JA, Enweronu-Laryea C, Debrah S. Multi-centre point-prevalence survey of hospital-acquired infections in Ghana. J Hosp Infect. 2019;101(1):60–8.
Article PubMed Google Scholar
Khan HA, Baig FK, Mehboob R. Nosocomial infections: Epidemiology, prevention, control and surveillance. Asian Pac J Trop Biomed. 2017;7(5):478–82.
Nimer NA. Nosocomial infection and antibiotic-resistant threat in the Middle East. Infection and drug resistance. 2022 Jan 1:631–9.
Hazard D, von Cube M, Kaier K, Wolkewitz M. Predicting potential prevention effects on hospital burden of nosocomial infections: a multistate modeling approach. Value in Health. 2021;24(6):830–8.
Bayleyegn B, Mehari A, Damtie D, Negash M. Knowledge, attitude and practice on hospital-acquired infection prevention and associated factors among healthcare workers at University of Gondar Comprehensive Specialized Hospital, Northwest Ethiopia. Infection and drug resistance. 2021 Jan 27:259–66.
Gilbert GL, Kerridge I. Hospital infection prevention and control (IPC) and antimicrobial stewardship (AMS): dual strategies to reduce antibiotic resistance (ABR) in hospitals. Ethics and Drug Resistance: collective responsibility for global Public Health. Oct. 2020;26:89–108.
Google Scholar
Ministry of Health, Ghana. National Policy and Guidelines for Infection Prevention and Control in Health Care Settings. 2015.
Assefa J, Diress G, Adane S. Infection prevention knowledge, practice, and its associated factors among healthcare providers in primary healthcare unit of Wogdie District, Northeast Ethiopia, 2019: a cross-sectional study. Antimicrob Resist Infect Control. 2020;9(1):1–9.
Chapman HJ, Veras-Estévez BA, Pomeranz JL, Pérez-Then EN, Marcelino B, Lauzardo M. The role of powerlessness among health care workers in tuberculosis infection control. Qual Health Res. 2017;27(14):2116–27.
Ocran I, Tagoe DN. Knowledge and attitude of healthcare workers and patients on healthcare associated infections in a regional hospital in Ghana. Asian Pac J Trop Disease. 2014;4(2):135–9.
Tahiru MM, KNOWLEDGE OF AND, COMPLIANCE TO INFECTION PREVENTION. AND CONTROL AMONG NURSES IN THE NORTHERN REGIONAL HOSPITAL (Doctoral dissertation).
Chitimwango PC. Knowledge, attitudes and practices of nurses in infection prevention and control within a tertiary hospital in Zambia [Thesis]. Stellenbosch University; 2017.
Bayleyegn B, Mehari A, Damtie D, Negash M, Knowledge. Attitude and practice on hospital-acquired infection Prevention and Associated factors among Healthcare Workers at University of Gondar Comprehensive Specialized Hospital, Northwest Ethiopia. Infect Drug Resist. 2021;14:259–66.
Article PubMed PubMed Central Google Scholar
Fawzi SE, Sleem WF, Shahien ES, Mohamed HA. Assessment of knowledge and practice regarding nosocomial infections. Port Said Sci J Nurs. 2019;6(1):83–100.
Qin YL, Bangura HS, Li B, Zhou YS, Yuan Y, Sun Y, et al. Self-reported knowledge and Practices of Healthcare Workers on Occupational exposure and Protection from Infectious Disease at the Military Hospital in Sierra Leone. Glob J Med Res. 2018;9(5):25–32.
Algarni SS, Sofar SM, Wazqar DY. Nurses’ knowledge and Practices toward Prevention of Catheter-Associated urinary tract infection at King Abdulaziz University. J Health Med Nurs. 2019;14:50–73.
Desta M, Ayenew T, Sitotaw N, Tegegne N, Dires M, Getie M. Knowledge, practice and associated factors of infection prevention among healthcare workers in Debre Markos referral hospital, Northwest Ethiopia. BMC Health Serv Res. 2018;18(1):465.
Teshager FA, Engeda EH, Worku WZ. Knowledge, practice, and Associated factors towards Prevention of Surgical Site infection among nurses working in Amhara Regional State Referral Hospitals, Northwest Ethiopia. Surg Res Pract. 2015;736–75.
Alhassan AR, Kuugbee ED, Der EM. Surgical healthcare workers knowledge and attitude on infection prevention and control: A case of tamale teaching hospital, Ghana. Canadian Journal of Infectious Diseases and Medical Microbiology. 2021;2021.
Iliyasu G, Dayyab FM, Habib ZG, Tiamiyu AB, Abubakar S, Mijinyawa MS, Habib AG. Knowledge and practices of infection control among healthcare workers in a Tertiary Referral Center in North-Western Nigeria. Ann Afr Med. 2016;15(1):34.
Download references
Acknowledgements
We would like to acknowledge all our study participants for their cooperation and voluntary participation during the data collection. We also thank the Hohoe Municipal Health Directorate for its support in the study.
This research was self-funded by the corresponding author.
Author information
Authors and affiliations.
Department of Epidemiology and Biostatistics, University of Health and Allied Sciences, Ho, Ghana
Samuel Salu
Department of Population and Health, University of Cape Coast, Cape Coast, Ghana
Joshua Okyere
School of Nursing & Midwifery, College of Health Sciences, Kwame Nkrumah University of Science and Technology, Kumasi, Ghana
Department of Population and Behavioral Sciences, University of Health and Allied Sciences, Ho, Ghana
Veronica Okwuchi Charles-Unadike & Mark Kwame Ananga
You can also search for this author in PubMed Google Scholar
Contributions
SS and VOC conceived and designed the study. VOC and MKA supervised the research work in the field. SS conducted the whole research work and wrote the drafts and revised manuscript. JO thoroughly reviewed and revised the manuscript and checked the references. SS and JO finalized the manuscript. All authors read and approved the final manuscript.
Corresponding author
Correspondence to Samuel Salu .
Ethics declarations
Competing interests.
The authors declare no competing interests.
Ethics approval and consent to participate
All methods were in accordance with the Declaration of Helsinki. The University of Health and Allied Sciences Research Ethics Committee (UHAS-REC) reviewed the study and approved it with a reference [ID: UHAS-REC A.11 {79} 20–21]. Permission was also obtained from the Hohoe Municipal Health Directorate before the commencement of the study. During data collection, permission was also sought from all the in-charges of the facilities before the administration of the questionnaire. Written informed consent was obtained from all of the participants.
Consent for publication
Not applicable.
Additional information
Publisher’s note.
Springer Nature remains neutral with regard to jurisdictional claims in published maps and institutional affiliations.
Electronic supplementary material
Below is the link to the electronic supplementary material.
Supplementary Material 1
Rights and permissions.
Open Access This article is licensed under a Creative Commons Attribution 4.0 International License, which permits use, sharing, adaptation, distribution and reproduction in any medium or format, as long as you give appropriate credit to the original author(s) and the source, provide a link to the Creative Commons licence, and indicate if changes were made. The images or other third party material in this article are included in the article’s Creative Commons licence, unless indicated otherwise in a credit line to the material. If material is not included in the article’s Creative Commons licence and your intended use is not permitted by statutory regulation or exceeds the permitted use, you will need to obtain permission directly from the copyright holder. To view a copy of this licence, visit http://creativecommons.org/licenses/by/4.0/ . The Creative Commons Public Domain Dedication waiver ( http://creativecommons.org/publicdomain/zero/1.0/ ) applies to the data made available in this article, unless otherwise stated in a credit line to the data.
Reprints and permissions
About this article
Cite this article.
Salu, S., Okyere, J., Charles-Unadike, V.O. et al. Nurses’ knowledge on nosocomial infections preventive measures and its associated factors in Ghana: a cross-sectional study. BMC Health Serv Res 23 , 941 (2023). https://doi.org/10.1186/s12913-023-09942-2
Download citation
Received : 26 May 2023
Accepted : 21 August 2023
Published : 01 September 2023
DOI : https://doi.org/10.1186/s12913-023-09942-2
Share this article
Anyone you share the following link with will be able to read this content:
Sorry, a shareable link is not currently available for this article.
Provided by the Springer Nature SharedIt content-sharing initiative
- Prevention Measures
BMC Health Services Research
ISSN: 1472-6963
- General enquiries: [email protected]
- Open access
- Published: 07 May 2024
Impact of interventions to reduce nosocomial transmission of SARS-CoV-2 in English NHS Trusts: a computational modelling study
- Stephanie Evans 1 , 2 ,
- James Stimson 1 , 2 ,
- Diane Pople 1 , 2 ,
- Peter J White 2 , 3 , 4 ,
- Mark H Wilcox 5 , 6 &
- Julie V Robotham 1 , 2 , 4 , 7
BMC Infectious Diseases volume 24 , Article number: 475 ( 2024 ) Cite this article
735 Accesses
81 Altmetric
Metrics details
Prior to September 2021, 55,000–90,000 hospital inpatients in England were identified as having a potentially nosocomial SARS-CoV-2 infection. This includes cases that were likely missed due to pauci- or asymptomatic infection. Further, high numbers of healthcare workers (HCWs) are thought to have been infected, and there is evidence that some of these cases may also have been nosocomially linked, with both HCW to HCW and patient to HCW transmission being reported. From the start of the SARS-CoV-2 pandemic interventions in hospitals such as testing patients on admission and universal mask wearing were introduced to stop spread within and between patient and HCW populations, the effectiveness of which are largely unknown.
Materials/methods
Using an individual-based model of within-hospital transmission, we estimated the contribution of individual interventions (together and in combination) to the effectiveness of the overall package of interventions implemented in English hospitals during the COVID-19 pandemic. A panel of experts in infection prevention and control informed intervention choice and helped ensure the model reflected implementation in practice. Model parameters and associated uncertainty were derived using national and local data, literature review and formal elicitation of expert opinion. We simulated scenarios to explore how many nosocomial infections might have been seen in patients and HCWs if interventions had not been implemented. We simulated the time period from March-2020 to July-2022 encompassing different strains and multiple doses of vaccination.
Modelling results suggest that in a scenario without inpatient testing, infection prevention and control measures, and reductions in occupancy and visitors, the number of patients developing a nosocomial SARS-CoV-2 infection could have been twice as high over the course of the pandemic, and over 600,000 HCWs could have been infected in the first wave alone. Isolation of symptomatic HCWs and universal masking by HCWs were the most effective interventions for preventing infections in both patient and HCW populations. Model findings suggest that collectively the interventions introduced over the SARS-CoV-2 pandemic in England averted 400,000 (240,000 – 500,000) infections in inpatients and 410,000 (370,000 – 450,000) HCW infections.
Conclusions
Interventions to reduce the spread of nosocomial infections have varying impact, but the package of interventions implemented in England significantly reduced nosocomial transmission to both patients and HCWs over the SARS-CoV-2 pandemic.
Peer Review reports
Introduction
Over the course of the COVID-19 pandemic in England, there has been evidence of nosocomial SARS-CoV-2 transmission to both patients and healthcare workers (HCWs) [ 1 , 2 , 3 , 4 ], with 0.5–1.25% of susceptible inpatients (55,000–90,000 patients) identified as having developed a nosocomial infection between 01-March-2020 and 01-Sept-2021 [ 5 , 6 ]. Several interventions have been introduced in hospitals to reduce the transmission rate of SARS-CoV-2 including regular testing of patients and HCWs, increased hand-hygiene, and HCWs wearing masks/face coverings universally (i.e., around both patients and HCWs), in addition to hospital system changes such as reductions in occupancy [ 7 , 8 ]. It is difficult to assess the effectiveness of individual interventions through data-driven approaches alone because several measures were implemented in quick succession. The emergence of vaccines and new variants have also altered nosocomial transmission rates over time, providing further uncertainty [ 5 ]. Computational modelling can help address these issues by providing a framework where individual interventions can be removed/reversed individually or in combination, counterfactual simulations can be executed, and the effectiveness of interventions assessed [ 9 ]. Using a previously developed computational model [ 10 ] we present an estimate of the impact of interventions in place during the pandemic, provide a counterfactual analysis of what might have happened had they not been implemented, and predict which combinations of interventions have the highest impact on transmission to both patients and HCWs.
We estimated the impact of eight hospital interventions/changes on nosocomial infections in both patients and HCW by 1) combining evidence from the literature on the efficacy/effectiveness of individual measures for reducing the spread of respiratory viral infections in hospitals and the community; 2) modifying model parameters to reflect scenarios in which the interventions were ‘reversed’ (both individually and collectively), e.g., through modifying the probability of transmission from inpatients and HCWs; and 3) modelling counterfactual scenarios in which interventions were ‘reversed’, i.e., simulating the hypothetical scenario in which the interventions/changes had not been implemented, to estimate how much virus transmission and resulting infections they averted individually and when combined.
Model development and calibration
We have extended an existing individual-based model (IBM) of nosocomial transmission within and between patient and HCW populations [ 9 , 10 ]. The model simulates transmission through different routes: i) patient-to-patient transmission between those sharing a bay, ii) patient-to-patient transmission between patients residing on the same ward but not necessarily in the same bay, representing transmission through, for example, fomites, shared facilities, or transient asymptomatic carriage by HCWs, among others, iii) patient-to-HCW transmission, iv) HCW-to-patient transmission, v) HCW-to-HCW while present on the same ward, and vi) HCW-to-HCW anywhere in the hospital. Indirect transmission is captured implicitly in the model as a result of transmission occurring on wards between any HCWs that have shared a space within a 4 h time step (and so in practice may not have occupied that space concurrently) and through the indirect transmission route whereby HCWs anywhere in the hospital may infect other staff with no explicit requirement for them to physically share a space at any time. Infected patient cases are imported from the community at a rate calculated from observed hospital admissions in the NHSE Situation Report data [ 6 ]. HCWs can become infected in the community when they are outside of the hospital according to the predicted community prevalence on that date, where the prevalence was calculated from the Cambridge Real-Time Model [ 11 ]. Under the baseline scenario, the IBM is parameterised using multiple national datasets and values from the literature and is calibrated to reproduce the transmission dynamics of SARS-CoV-2 among healthcare workers (HCWs) and patients in an average English hospital. A full model description and details of the calibration procedure are described in Supplementary File 1 .
Counterfactual modelling of ‘reversal’ of interventions
Counterfactual scenarios were simulated in which eight interventions were reversed (individually and collectively) and compared to a baseline where interventions were in place, with scenarios as follows: 1) Baseline – transmission and interventions simulated to reflect observed numbers of infections in the data; 2) removal of lateral flow device tests (LFDs) for HCWs that were introduced in November 2020; 3) reversal of improvements in hand-hygiene from the start of the pandemic; 4) reversal of testing and cohorting of symptomatic patients throughout the simulation period; 5) reversal of reduced occupancy, instead with occupancy remaining at 2019 levels March to May 2020; 6) reversal of suspension of visitors to hospital patients (March to October 2020); 7) removal of universal mask wearing by HCWs (i.e., HCWs wear masks around patients but not around other HCWs) from June 2020; 8) removal of mask wearing by HCWs when treating patients (i.e., HCWs do not wear masks around patients or other HCWs) throughout simulation period; 9) isolation of symptomatic HCWs removed throughout simulation period, 10) reversal of all interventions in 2–9 collectively. The reversal of interventions was parameterised through literature searches and expert elicitation where there was a paucity of evidence (Table 1 , Supplementary File 2 ). A supplementary analysis was also performed exploring a further set of counterfactual scenarios where interventions were in place from the start of the pandemic instead of being introduced at different times as in reality.
After obtaining data for parameterising the reversal of interventions, new parameter sets were generated using the procedure described in Fig. S 1 and the Spartan R package [ 20 ]. The steps are i) 10 unique baseline transmission parameter sets were drawn from previous model calibrations [ 10 ], ii) for each intervention scenario 100 new parameters were generated by sampling 100 unique parameter values from the distribution of the combined study data, iii) each set was mapped to one of the unique 10 transmission parameter sets to produce 100 new parameter sets. To reverse interventions where there was a single value, e.g., increased occupancy, each baseline transmission parameter set was replicated 10 times and then modified to include the single new parameter value in all sets.
These new parameters were used within the IBM to generate estimates of the impact of each intervention individually (in terms of nosocomial infections averted in inpatients and in HCW) as well as an overall estimate of the combined impact of all interventions that were implemented during the COVID-19 pandemic in England.
The model was simulated for 5100 timesteps covering a time period of 850 days from 03-March-2020 to 30-June-2022 (6 steps per simulated day). Individual-level patient and HCW data on infection status and location were recorded at each time step.
Predictive modelling of the impact of combinations of interventions on infection rates
In a further analysis to explore the effect of intervention combinations, a list of all possible combinations of interventions described above was generated (255 total) and then explored. Unlike the previous analysis where vaccines and variants emerged during the pandemic and interventions were put in place at times specified by policy, here we restrict simulation to a 12-week Omicron-like period where the prevalence ranged from 2–4% (similar to January 2022), assuming no protection from previous infection or vaccination. This time period was selected to provide enough time to observe the effect of interventions while maintaining stable prevalence levels in line with community estimates. In this analysis all interventions were removed and then added back either singularly or as part of a package. Simulations were parameterised to reflect the hypothetical removal of all interventions except those included in the combination i.e., in a scenario where patient testing and LFD testing of HCWs were included, all other interventions are reversed as described in Table 1 . Parameter files were generated as described above. The results of these simulations were then fed into a linear regression model to estimate the overall impact of each intervention. A second model was used to estimate the impact of combinations of the interventions that were significantly associated with a decrease in the number of infections in model 1.
Results are presented as the number of susceptible inpatients and patient-facing HCWs becoming infected with SARS-CoV-2 under each intervention scenario across the simulation period. To scale up the single-hospital simulation estimate to a national level, the total number of infections prevented is divided by the total number of beds in the simulated hospital (a proxy for hospital size) and then multiplied by the total number of beds across NHS hospitals in England using national data [ 15 ]. This assumes that occupancy was similar between all NHS hospitals.
Impact of interventions on nosocomial infections in patients: counterfactual modelling
In the baseline scenario where all interventions were in place, a maximum of 12,300 patients were infected in a single week and 240,000 over the simulation period (March 2020-July 2022, Fig. 1 , Table 2 , 3.5% of all susceptible admissions). Removing all interventions results in a worst-case scenario of up to 22,000 patients infected in a single week and 560,000 over the simulation period (6.25% %))of all susceptible admissions), a more than two times increase compared to the baseline. The most effective interventions were patient testing and universal masking by HCWs, and removing these interventions resulted in a total of 326,000 and 310,000 patient infections respectively, over the simulation period. Patient testing and universal masking by HCWs were most effective over the omicron wave (from December 2021), and testing was most effective over the first and second waves (March 2020 to May 2021). The least effective intervention overall was restricting visitation: removing this intervention did not result in an increase in infections; however, this intervention was only in place for a short time and therefore only has a limited opportunity for effectiveness (Fig. S 2 ). In a scenario where visitation was restricted throughout the entire simulation period there were 44% fewer patient infections during the omicron wave (Fig. S 3 ). Interventions were most impactful before July 2020 when the wild-type (WT)wave first hit England and between December 2021 and March 2022 when the omicron wave first started. This is demonstrated by the greatest difference between the simulations with no interventions (yellow lines) compared to the baseline (grey lines).
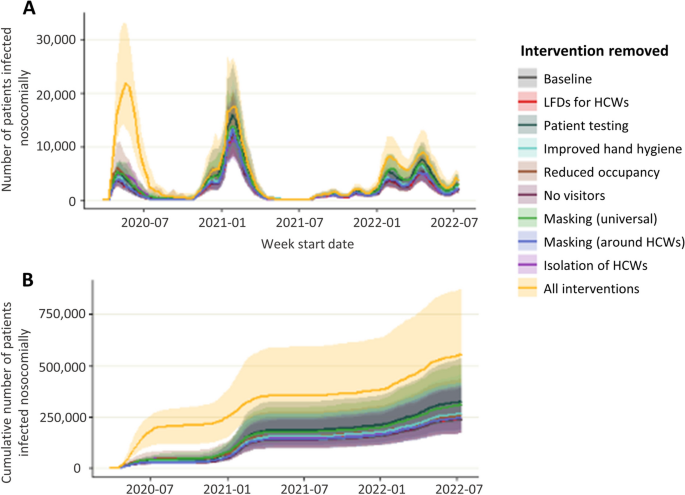
Number of nosocomial patient infections when single or all interventions are removed. Number of patients infected per week ( A ) and cumulative number of patient infections ( B ) when individual or combined interventions are lifted over the course of the pandemic
Impact of interventions on HCW infections: counterfactual modelling
In the baseline scenario where all interventions were in place, a maximum of 17,500 (median, IQR in Table 2 ) HCWs were infected in a single week and there were 595,000 HCW infections over the simulation period (Fig. 2 ). When all interventions were removed simultaneously, a maximum of 65,000 HCWs were infected in a single week (10% of all staff) and there were 1 million (0.9 – 1.1 million) infection events in HCWs over the simulation period. The most effective interventions were isolation of symptomatic HCWs, masking universally, and masking around patients. When HCW isolation was removed, up to 40,000 HCWs were infected in a single week, and there were 780,000 infection events in HCWs. Removing masking universally resulted in a maximum of 24,000 HCWs infected in a single week and 850,000 infection events in HCWs. When masking was only in place around patients and not other HCWs a maximum of 18,000 HCW infections in a single week and there was a total of 750,000 HCW infection events. Masking was most effective during the first wave of the pandemic (March – August 2020), and HCW isolation was most effective both during the first wave and also over the omicron wave (from December 2021). When interventions are removed the main risk to HCWs becomes the hospital, with almost 75% of infections in HCWs being hospital acquired in 2022 (Fig. 3 ). Relaxing interventions switches the balance of infections from the community to the hospital.
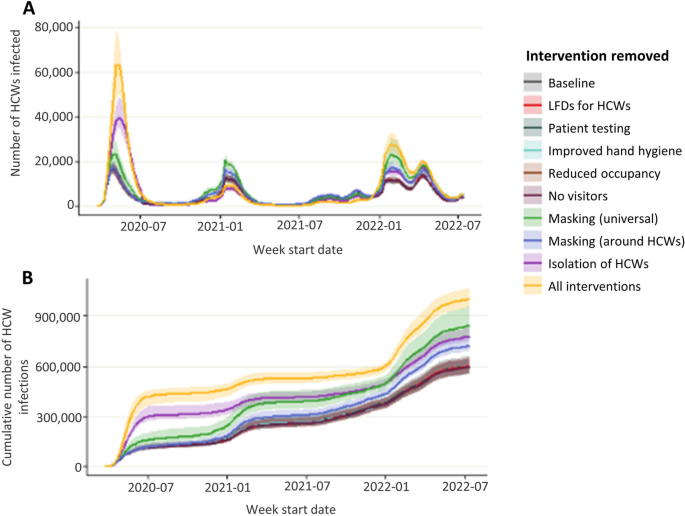
Number of HCW infections when single or all interventions are removed. Number of HCWs infected per week ( A ) and cumulative number of HCW infections ( B ) when individual or combined interventions are lifted over the course of the pandemic
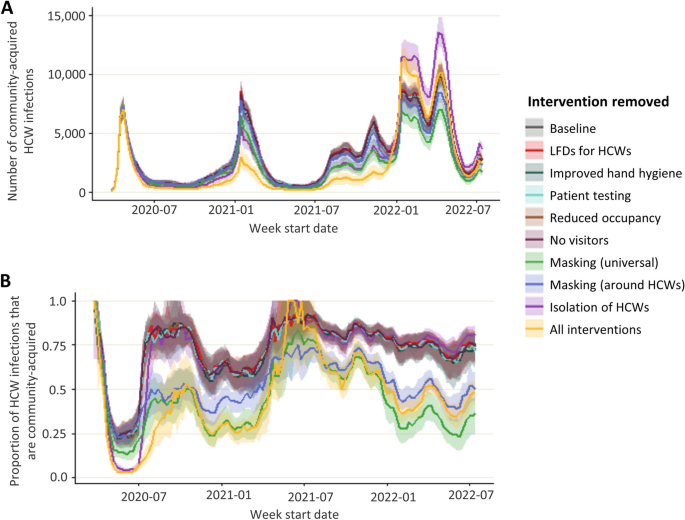
A ) Community HCW infections B ) proportion of community HCW infections
Impact of combined interventions on patient nosocomial infections: predictive modelling
In a scenario with no protection from previous infection or vaccination where all interventions were removed, and then added back in either singularly or as part of a package, restricting visitation as a single intervention was the least effective option, with 270,000 patients nosocomially infected over a three-month period (Fig. 4 A). The most effective single intervention was isolation of symptomatic HCWs preventing 81% of infections compared to restricting visitation alone (50,000 vs 270,000). When HCWs testing and isolation upon developing symptoms are in place, both universal masking and masking around patients significantly further reduce infections by up to 25% (36,000 vs 48,000) compared to restricting visitation alone, but there is no clear advantage to universal masking over masking only around patients for preventing nosocomial patient infections. However, when HCWs do not isolate then universal masking has a slight advantage over masking only around patients with an additional 11% reduction in infections (maximum of 107,000 infections when masks are worn universally vs 121,000 when worn only around patients).
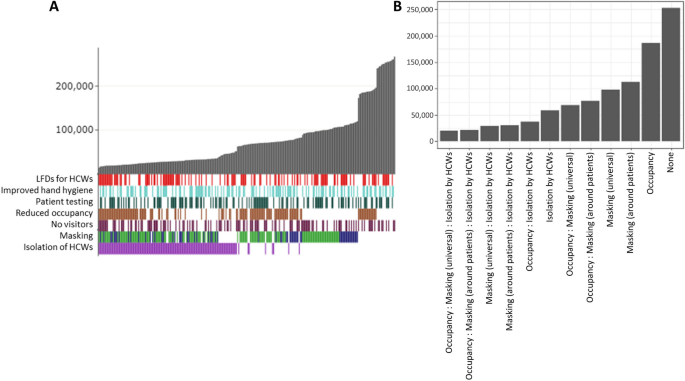
Modelled combined effects of interventions on patients. A ) Simulated number of patients infected over a 12-week omicron-like period (grey bars) under package of interventions (coloured bars = interventions in package, white = intervention not in package) ordered by infection rate. For masking, green bars represent universal masking in package and blue represents only masking around HCWs. Each individual bar = single combination of interventions. B ) Predicted number of patient infections over a 12-week omicron-like period under each combination of interventions from statistical model of simulated data
A regression model on the simulated infection rates further validates these findings and identifies significant reductions in nosocomial patient infections when universal masking (β = -79,394.50, p < 0.001), masking around patients (β = -73,212.08, p < 0.001), isolation by HCWs (β = -89,522.22, p < 0.001) and, with smaller impact, reducing occupancy rates (β = -26,261.75, p < 0.001) (Table 3 ). A secondary analysis exploring the combined effect of the significant interventions (HCW isolation, reduced occupancy, and masking by HCWs either universally or around patients) demonstrates that isolation by HCWs has a larger effect on patient infections than a combination of masking and reducing occupancy and that combining all three interventions has the greatest effective (Table 3 , Fig. 4 B).
Impact of combined interventions on HCW infections: predictive modelling
For HCW infections, increased hand-hygiene alone was the least effective intervention resulting in 572,000 infections over a three-month period compared to a maximum of 206,000 infections under the most effective single intervention, isolation of symptomatic HCWs (Fig. 5 A). Masking (both universal and around patients only) was also effective when HCWs do not isolate, with universal masking more effective than masking around patients (16% reduction in infections, maximum of 538,000 vs 451,000 HCWs infected). When HCWs do isolate, the difference in mask types is less pronounced and the impact appears to be related to other interventions e.g., LFD testing.
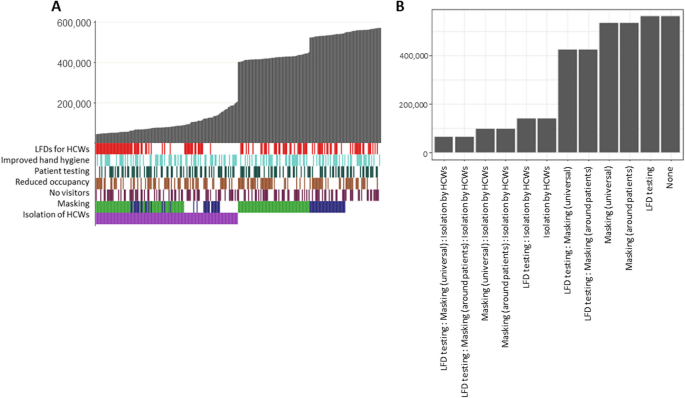
Modelled combined effects of interventions on HCWs . A ) Simulated number of HCWs infected over a 12-week omicron-like period (grey bars) under package of interventions (coloured bars = interventions in package, white = intervention not in package) ordered by infection rate. For masking, green bars represent universal masking in package and blue represents only masking around HCWs. Each individual bar = single combination of interventions. B ) Predicted number of HCW infections over a 12-week omicron-like period under each combination of interventions from statistical model of simulated data
Statistical modelling of the simulated infection rates identifies significant reductions in transmission associated with lateral flow testing of HCWs (β = -22,464.66, p < 0.001, Table 4 ) universal masking (β = -106,902.84, p < 0.001), masking around patients (β = -34,552.93, p < 0.001), and isolation of symptomatic HCWs (β = -394,655.48, p < 0.001). There is also a small association with reduced occupancy (β = -8,2090.0, p = 0.007). A further analysis exploring the impact of the three most significant interventions combined demonstrates that isolation of symptomatic HCWs alone is more impactful than masking or LFD testing combined and that a combination of universal masking, LFD testing and isolation of symptomatic HCWs is the most effective way to prevent transmission (Table 4 , Fig. 5 B).
We evaluated the counterfactual impact of removing any or all of eight interventions that were implemented in NHS England hospitals over the COVID-19 pandemic on rates of nosocomial transmission to patients and HCWs in England. While highly uncertain, given the dependency on variable evidence on the effectiveness of interventions, the model results suggest that interventions in place over the COVID-19 pandemic in England prevented over 50% of potential nosocomial infections in patients and HCWs. Testing and cohorting of patients and isolation of HCWs were the most important interventions for reducing transmission to patients and HCWs preventing up to 34% (30–40%) of infections. We also identified a significant role for masking, with universal masking being more impactful than masking around patients alone (40% (30–52%) vs 17% (14–20%) reduction). Results suggest that restricting visitation could be impactful when community prevalence rates are high. Interventions were most impactful when protection from vaccines or previous infection was low at the start of the wild-type and omicron waves and had little impact in times of high immunity.
To the best of our knowledge this is the first study to attempt to quantify the impact of IPC measures over the COVID-19 pandemic in a hospital setting. A literature search conducted to parameterise the model in this study highlighted a paucity of evidence around the effectiveness of interventions such as improving hand-hygiene, as demonstrated by other studies [ 21 ], and a wide degree of uncertainty in the effectiveness of others such as masking on reducing nosocomial transmission of respiratory viruses. Further there is a lack of evidence around compliance with any interventions that were implemented. While the model used in this study includes the effect of vaccines, as administered during this period, on both patients and HCWs, we do not evaluate the impact of alternative vaccine availability or uptake. The modelling approach used in this study has previously been used to estimate the counterfactual HCW infections assuming absence of vaccines in the second wave of the pandemic and found prioritising HCWs for vaccinations was extremely important for reducing infection rates in HCWs [ 9 ].
While the baseline results are calibrated to high-quality national datasets, the scarcity of reliable evidence on the effectiveness of individual interventions (required for simulating a scenario where they are reversed) is a key challenge. While the model has been parameterised to best reflect the available data, uncertainty remains around the contribution of nosocomial and community sources of SARS-CoV-2 infection. As COVID-19 becomes an endemic disease and pressures on health systems from other seasonal respiratory pathogens increase, there is a critical need for evidence on the effectiveness of such interventions on reducing nosocomial spread in order to design efficient and effective infection prevention and control strategies.
In this study we assumed full compliance with interventions such as testing and isolating (although the compliance in studies used to parameterise masking and hand-hygiene was not reported), and the impact of reversing an intervention was applied to every interaction at every timestep that the intervention was in place. If compliance was poor or changed throughout the study period, then the modelled impact of an intervention would fall. In the absence of data on policy implementation across trusts over time, we assumed uniformity between trusts in changing behaviours according to policy guidelines. When off shift, the infection risk for HCWs was uniform, and we did not account for individual-level differences in risk of infection in the community. Further, we assumed that the effect of interventions was the same regardless of the strain on SARS-CoV-2 that was circulating at the time. We also made the assumption that it is appropriate to scale up the average results from our simulations to a national level using only numbers of beds therefore implying that all trusts were similar in terms of occupancy and admissions rates throughout the pandemic. If this was not the case, the results would need to be viewed at an individual hospital level, and although we expect the general trends to hold, the magnitude of interventions' effects may change. Similarly, for HCWs we scaled the results to a national level using total patient-facing HCW counts alone and assumed that the infection risk for staff off-shift was uniform which may not be true in reality and we could be under or overestimating the impact of interventions such as masking if staff were more or less risk-averse than the general population when off-shift. Due to policies being implemented universally and in quick succession at the start of the pandemic, there is limited opportunity to analyse their efficacy from a data-driven perspective; however, a small number of studies exist that validate the results of this modelling study. A single-site study showed that between the first and second waves the proportion of nosocomial infections in HCWs attributable to HCW-to-HCW transmission fell from 55.3% in wave 1 (01-March-2020 to 25-July-2020) to 37.4% in wave 2 (30-Nov-2020 to 24-Jan-2021) despite the proportion of community-acquired infections remaining at 50% [ 2 ]. This suggests that the introduction of masks in communal spaces in June 2020 potentially played a role in reducing HCW-to-HCW transmission by as much as 32%. This is consistent with our estimate that over wave 1 an additional 50% (27%-57%) of HCWs would have been infected had universal masking not being introduced. Another study examined the impact of removing HCW masking on patient infections and did not see a significant increase in infections when masks were removed during the first 10 months of omicron when immunity from previous infection and/or vaccination was high [ 22 ]. This agrees with simulation results where an increase in patient infections in the absence of masking was observed in the wild-type and early omicron waves, but there is little difference when vaccination and immunity from previous infection has increased protection. We did not find a notable increase in nosocomial patient infections following the withdrawal of asymptomatic testing of patients and HCWs or of masking late in 2022.
This analysis identified testing and isolation of symptomatic HCWs and masking by HCWs around both patients and other HCWs as the most important interventions for reducing infections in the patient and HCW populations. There is evidence from early in the pandemic that HCW-to-HCW transmission commonly occurred and the risk of HCW-to-patient transmission is likely small [ 2 , 3 , 4 , 10 ]. However, there remains a potential for a larger effect of a small number of HCW-to-patient transmissions seeding larger outbreaks on patient wards. This cannot be ignored, and likely contributes to the increased impact of interventions that minimise HCW-to-patient transmission. However, there remains a. This impact. A strength of the IBM is that it captures these ‘knock-on’ benefits associated with prevention of transmission chains both within and across patient and HCW populations; such combined/bundled effects are sometimes referred to as the ‘Swiss Cheese’ infection prevention model [ 23 , 24 ]. This cumulative effect of reducing transmissions is apparent when looking at the impact of removing all interventions in combination, which results in a higher number of nosocomial infections than the sum of the individual interventions. Model findings suggest that collectively the interventions introduced over the SARS-CoV-2 pandemic in England averted 400,000 (240,000 – 500,000) infections in inpatients and 410,000 (370,000 – 450,000) HCW infections.
Masking was found to be a highly effective intervention, but due to a lack of studies exploring the impact of different mask types we consider the overall impact of any masking in this study and do not distinguish respirators from fluid resistant surgical masks (FRSMs). A previous modelling study suggested that respirators may be advantageous for preventing infections in HCWs; however, the authors explicitly state that their study provides evidence that more work should be done to determine the true effectiveness of respirators over FRSMs [ 25 ], and no further convincing evidence has yet emerged. Analysis of intervention reversal only considered those described; additional measures such a double gloving or sessional gown use are not included in this work. The impact of interventions such as increasing bed spacing and improving ventilation were not modelled as their impact could not be included in the existing modelling framework upon which this study was conducted. While we have attempted to estimate the impact of results on infection rates, the adverse impact of interventions such as restricting visitation or mask wearing on patient care and staff wellbeing have been well documented and cannot be ignored [ 26 , 27 , 28 , 29 , 30 , 31 , 32 , 33 , 34 , 35 , 36 ]. This extensive literature should be considered alongside the results of modelling work when making future policy decisions.
This study combines a transmission model with published parameter estimates on the impact of individual measures to evaluate the contribution that the collection of interventions and hospital changes in place over the COVID-19 pandemic in England made to the reduction of nosocomial transmission, quantifying both individual intervention and collective impact. A strength of the modelling approach used here is the ability of the model to capture cumulative effects of interventions through reducing the seeding of new infection clusters. These results highlight the importance of maintaining high levels of compliance to infection prevention and control measures in hospitals and have important implications as hospitals prepare for a surge in demand due to emerging winter pressures and COVID-19.
Availability of data and materials
All UKHSA-held data (from SUS and SGSS as detailed in Supplementary File 1 ) were collected within statutory approvals granted to UKHSA for infectious disease surveillance and control. Information was held securely and in accordance with the Data Protection Act 2018 and Caldicott guidelines. The data that support the findings of this study are available from NHS Digital but restrictions apply to the availability of these data, which were used under license for the current study, and so are not publicly available. The datasets used and/or analysed during the current study are however available from the corresponding author on reasonable request and with permission of NHS Digital.
Bhattacharya A, Collin SM, Stimson J, Thelwall S, Nsonwu O, Gerver S, et al. Healthcare-associated COVID-19 in England: A national data linkage study. J Infect. 2021;83(5):565–72.
Article CAS PubMed PubMed Central Google Scholar
Lindsey BB, Villabona-Arenas ChJ, Campbell F, Keeley AJ, Parker MD, Shah DR, et al. Characterising within-hospital SARS-CoV-2 transmission events using epidemiological and viral genomic data across two pandemic waves. Nat Commun. 2022;13(1):671.
Illingworth CJ, Hamilton WL, Warne B, Routledge M, Popay A, Jackson C, et al. Superspreaders drive the largest outbreaks of hospital onset COVID-19 infections. Walczak AM, Ogbunugafor CB, Cobey SE, editors. eLife. 2021;24(10):e67308.
Article Google Scholar
Ellingford JM, George R, McDermott JH, Ahmad S, Edgerley JJ, Gokhale D, et al. Genomic and healthcare dynamics of nosocomial SARS-CoV-2 transmission. eLife. Available from: https://www.ncbi.nlm.nih.gov/pmc/articles/PMC8009659 /. Cited 2021 May 21;10
Stimson J, Pouwels KB, Hope R, Cooper BS, Presanis AM, Robotham JV. Estimation of the impact of hospital-onset SARS-CoV-2 infections on length of stay in English hospitals using causal inference. BMC Infect Dis. 2022;22(1):1–12.
Digital NHS. Hospital Episode Statistics (HES): Provisional Monthly Statistics for Admitted Patient Care, Outpatient and Accident & Emergency. Available from: https://data.gov.uk/dataset/c914f943-98d1-4dee-aba5-c40a7d08eefa/hospital-episode-statistics-hes-provisional-monthly-statistics-for-admitted-patient-care-outpatient-and-accident-emergency . Cited 2020 May 18.
May R, Powis S. Expansion of patient testing for Covid-19. 2020.
COVID-19: infection prevention and control (IPC). GOV.UK. Available from: https://www.gov.uk/government/publications/wuhan-novel-coronavirus-infection-prevention-and-control . Cited 2021 Mar 12.
Pople D, Monk EJM, Evans S, Foulkes S, Islam J, Wellington E, et al. Burden of SARS-CoV-2 infection in healthcare workers during second wave in England and impact of vaccines: prospective multicentre cohort study (SIREN) and mathematical model. BMJ. 2022;20(378): e070379.
Evans S, Stimson J, Pople D, Bhattacharya A, Hope R, White PJ, et al. Quantifying the contribution of pathways of nosocomial acquisition of COVID-19 in English hospitals. Int J Epidemiol. 2022;51(2):393–403.
Article PubMed Google Scholar
Birrell P, Blake J, van Leeuwen E, Gent N, De Angelis D. Real-time nowcasting and forecasting of COVID-19 dynamics in England: the first wave. Philosophical Transactions of the Royal Society B: Biological Sciences. 1829;2021(376):20200279.
Google Scholar
Coronavirus » Novel coronavirus (COVID-19) standard operating procedure. Available from: https://www.england.nhs.uk/coronavirus/wp-content/uploads/sites/52/2020/11/C0873_i_SOP_LFD-rollout-for-asymptomatic-staff-testing_phase-2-trusts-v1.1_16-nov20.pdf . Cited 2020 Dec 1.
Quilty BJ, Clifford S, Hellewell J, Russell TW, Kucharski AJ, Flasche S, et al. Quarantine and testing strategies in contact tracing for SARS-CoV-2: a modelling study. Lancet Public Health. 2021;6(3):e175–83.
Article PubMed PubMed Central Google Scholar
May R, Powis S, Issar P, Philip P. Healthcare associated COVID-19 infections – further action. 2020. Available from: https://www.england.nhs.uk/coronavirus/wp-content/uploads/sites/52/2020/06/Healthcare-associated-COVID-19-infections--further-action-24-June-2020.pdf
Statistics » Bed Availability and Occupancy. Available from: https://www.england.nhs.uk/statistics/statistical-work-areas/bed-availability-and-occupancy/ . Cited 2022 Dec 21.
NHSE/I updates guidance on inpatient visiting, allowing it only “in a very careful and COVID-secure way”. COVID-19 policy tracker. Available from: https://covid19.health.org.uk/theme/nhsei-updates-guidance-inpatient-visiting-allowing-it-only-very-careful-and-covid-secure-way . Cited 2023 Jul 3.
Temime L, Gustin M-P, Duval A, Buetti N, Crépey P, Guillemot D, et al. A Conceptual Discussion About the Basic Reproduction Number of Severe Acute Respiratory Syndrome Coronavirus 2 in Healthcare Settings. Clin Infect Dis. 2021;72(1):141–3.
CAS PubMed Google Scholar
Face masks and coverings to be worn by all NHS hospital staff and visitors. GOV.UK. Available from: https://www.gov.uk/government/news/face-masks-and-coverings-to-be-worn-by-all-nhs-hospital-staff-and-visitors . Cited 2023 Jul 3.
Hoernke K, Djellouli N, Andrews L, Lewis-Jackson S, Manby L, Martin S, et al. Frontline healthcare workers’ experiences with personal protective equipment during the COVID-19 pandemic in the UK: a rapid qualitative appraisal. BMJ Open. 2021;11(1): e046199.
Alden K, Read M, Timmis J, Andrews PS, Veiga-Fernandes H, Coles M. Spartan: A Comprehensive Tool for Understanding Uncertainty in Simulations of Biological Systems. PLoS Comput Biol. 2013;9(2): e1002916.
Jafari Y, Yin M, Lim C, Pople D, Evans S, Stimson J, et al. Effectiveness of infection prevention and control interventions, excluding personal protective equipment, to prevent nosocomial transmission of SARS-CoV-2: a systematic review and call for action. Infect Prev Pract. 2022;4(1): 100192.
Patterson B, Mehra R, Breathnach A. Unmasking the mask: A time-series analysis of nosocomial COVID-19 rates before and after removal. In Copenhagen: ECCMID; 2023. (conference abstract).
Noh JY, Song JY, Yoon JG, Seong H, Cheong HJ, Kim WJ. Safe hospital preparedness in the era of COVID-19: The Swiss cheese model. Int J Infect Dis. 2020;98:294–6.
Yanke E, Carayon P, Safdar N. Translating evidence into practice using a systems engineering framework for infection prevention. Infect Control Hosp Epidemiol. 2014;35(9):1176–82.
Ferris M, Ferris R, Workman C, O’Connor E, Enoch DA, Goldesgeyme E, et al. Efficacy of FFP3 respirators for prevention of SARS-CoV-2 infection in healthcare workers. Elife. 2021;16:10.
Hugelius K, Harada N, Marutani M. Consequences of visiting restrictions during the COVID-19 pandemic: An integrative review. Int J Nurs Stud. 2021;121: 104000.
Mheidly N, Fares MY, Zalzale H, Fares J. Effect of Face Masks on Interpersonal Communication During the COVID-19 Pandemic. Front Public Health. 2020 Dec. Available from: https://www.frontiersin.org/articles/ https://doi.org/10.3389/fpubh.2020.582191/full . Cited 2023 Jan 10;8.
Nicholls I, Saada L. Communication, Confusion, and COVID-19: the challenges of wearing PPE on a geriatrics ward during the COVID-19 pandemic. Re: Patient perspective: Gordon Sturmey and Matt Wiltshire,. BMJ. 2023 Jan 10; Available from: https://www.bmj.com/content/369/bmj.m1814/rr . Cited 2023 Jan 10.
Bloemberg D, Musters SCW, van der Wal-Huisman H, van Dieren S, Nieveen van Dijkum EJM, Eskes AM. Impact of family visit restrictions due to COVID19 policy on patient outcomes A cohort study. J Adv Nurs. 2022;78(12):4042–53.
Aengst J, Walker-Stevenson G, Harrod T, Ivankovic J, Neilson J, Guise J-M. Uncomfortable yet necessary: The impact of PPE on communication in emergency medicine. Int J Qual Health Care. 2022 Dec 28;34(4). Available from: https://academic.oup.com/intqhc/article/34/4/mzac095/6854825 . Cited 2023 Jan 10.
Muzzi E, Chermaz C, Castro V, Zaninoni M, Saksida A, Orzan E. Short report on the effects of SARS-CoV-2 face protective equipment on verbal communication. Eur Arch Otorhinolaryngol. 2021;278(9):3565–70.
Hampton T, Crunkhorn R, Lowe N, Bhat J, Hogg E, Afifi W, De S, Street I, Sharma R, Krishnan M, Clarke R, Dasgupta S, Ratnayake S, Sharma S. The negative impact of wearing personal protective equipment on communication during coronavirus disease 2019. J Laryngol Otol. 2020;134(7):577–81. https://doi.org/10.1017/S0022215120001437 .
Swaminathan R, Mukundadura BP, Prasad S. Impact of enhanced personal protective equipment on the physical and mental well-being of healthcare workers during COVID-19. Postgrad Med J. 2022;98(1157):231–3.
Aloweni F, Bouchoucha SL, Hutchinson A, Ang SY, Toh HX, Suhari NAB, et al. Health care workers’ experience of personal protective equipment use and associated adverse effects during the COVID-19 pandemic response in Singapore. J Adv Nurs. 2022;78(8):2383–96.
Ayton D, Soh S-E, Berkovic D, Parker C, Yu K, Honeyman D, et al. Experiences of personal protective equipment by Australian healthcare workers during the COVID-19 pandemic, 2020: A cross-sectional study. PLoS ONE. 2022;17(6): e0269484.
Galanis P, Vraka I, Fragkou D, Bilali A, Kaitelidou D. Impact of personal protective equipment use on health care workers’ physical health during the COVID-19 pandemic: A systematic review and meta-analysis. Am J Infect Control. 2021;49(10):1305–15.
Download references
Acknowledgements
We thank the experts who participated in the elicitation exercise and also the participants of model review and IPC effectiveness workshops that helped to shape the direction of this project. We also thank the SIREN team at the UKHSA for their data insight throughout the development of this model, and all those involved in running SIREN at participating sites and SIREN participants.
The views expressed are those of the authors and not necessarily those of the UK Department of Health and Social Care (DHSC), FCDO, EU, MRC, NIHR, or UKHSA.
PJW was supported by the MRC Centre for Global Infectious Disease Analysis (grant number MR/X020258/1); this award comes under the Global Health EDCTP3 Joint Undertaking. PJW was also supported by the NIHR Health Protection Research Unit in Modelling and Health Economics, which is a partnership between the UKHSA, Imperial College London, and LSHTM (grant code NIHR200908). PJW has received payment from Pfizer for teaching of mathematical modelling of infectious disease transmission and vaccination, and from the Dutch National Institute for Public Health and the Environment (RIVM) for participation in an audit committee on COVID-19 data analytics and modelling.
Funding sources had no role in the design of this study.
Author information
Authors and affiliations.
Fungal, HCAI, AMU & Sepsis Division, UK Health Security Agency, London, AMR, UK
Stephanie Evans, James Stimson, Diane Pople & Julie V Robotham
Statistics, Modelling and Economics, UK Health Security Agency, London, UK
Stephanie Evans, James Stimson, Diane Pople, Peter J White & Julie V Robotham
MRC Centre for Global Infectious Disease Analysis, Imperial College London, London, UK
Peter J White
NIHR Health Protection Research Unit in Modelling and Health Economics at Imperial College London in partnership with the UK Health Security Agency and London School of Hygiene and Tropical Medicine, London, UK
Peter J White & Julie V Robotham
Healthcare-Associated Infections Research Group, Leeds Institute of Medical Research, University of Leeds, Leeds, LS1 9JT, UK
Mark H Wilcox
Microbiology, Leeds Teaching Hospitals, Leeds, UK
NIHR Health Protection Research Unit in Healthcare Associated Infections and Antimicrobial Resistance at University of Oxford in partnership with the, UK Health Security Agency, Oxford, UK
Julie V Robotham
You can also search for this author in PubMed Google Scholar
Contributions
SE, JR, MW, and PW conceptualised the study, developed the methodology and interpreted the results. SE developed and parameterised the model, ran the analysis, and wrote the manuscript. JS and DP curated the data for parameterisation and reviewed the manuscript.
Corresponding author
Correspondence to Stephanie Evans .
Ethics declarations
Ethics approval and consent to participate.
This is a computational study and no results using human data are reported. Data were used to calibrate the model as described in previous studies. Ethical approval and inclusion of personal data without direct consent to participate was reviewed by the United Kingdom Health Security Agency’s (UKHSA’s) governance processes, and the work was authorised to process identifiable data under Regulation 3 of Sect. 251 of the National Health Service Act 2006. No specific administrative permissions and/or licenses were acquired to access the clinical and personal patient data used in this research.
Consent for publication
Not applicable.
Competing interests
The authors have no competing interests to disclose.
Additional information
Publisher’s note.
Springer Nature remains neutral with regard to jurisdictional claims in published maps and institutional affiliations.
Supplementary Information
Supplementary file 1., supplementary file 2., supplementary file 3., rights and permissions.
Open Access This article is licensed under a Creative Commons Attribution 4.0 International License, which permits use, sharing, adaptation, distribution and reproduction in any medium or format, as long as you give appropriate credit to the original author(s) and the source, provide a link to the Creative Commons licence, and indicate if changes were made. The images or other third party material in this article are included in the article's Creative Commons licence, unless indicated otherwise in a credit line to the material. If material is not included in the article's Creative Commons licence and your intended use is not permitted by statutory regulation or exceeds the permitted use, you will need to obtain permission directly from the copyright holder. To view a copy of this licence, visit http://creativecommons.org/licenses/by/4.0/ . The Creative Commons Public Domain Dedication waiver ( http://creativecommons.org/publicdomain/zero/1.0/ ) applies to the data made available in this article, unless otherwise stated in a credit line to the data.
Reprints and permissions
About this article
Cite this article.
Evans, S., Stimson, J., Pople, D. et al. Impact of interventions to reduce nosocomial transmission of SARS-CoV-2 in English NHS Trusts: a computational modelling study. BMC Infect Dis 24 , 475 (2024). https://doi.org/10.1186/s12879-024-09330-z
Download citation
Received : 10 August 2023
Accepted : 18 April 2024
Published : 07 May 2024
DOI : https://doi.org/10.1186/s12879-024-09330-z
Share this article
Anyone you share the following link with will be able to read this content:
Sorry, a shareable link is not currently available for this article.
Provided by the Springer Nature SharedIt content-sharing initiative
- Infection prevention and control
- Healthcare-associated infection
- Individual-based model
- Agent-based model
BMC Infectious Diseases
ISSN: 1471-2334
- General enquiries: [email protected]
- Open access
- Published: 24 August 2020

Infection prevention and control research priorities: what do we need to combat healthcare-associated infections and antimicrobial resistance? Results of a narrative literature review and survey analysis
- Yohann Lacotte ORCID: orcid.org/0000-0002-8282-9327 1 ,
- Christine Årdal 2 &
- Marie-Cécile Ploy 1
on behalf of the European Union Joint Action on Antimicrobial Resistance and Healthcare-Associated Infections (EU-JAMRAI)
Antimicrobial Resistance & Infection Control volume 9 , Article number: 142 ( 2020 ) Cite this article
22k Accesses
11 Citations
29 Altmetric
Metrics details
Infection prevention and control (IPC) is one of the most cost-effective interventions against antimicrobial resistance (AMR). Yet, IPC knowledge gaps often receive little prominence in AMR research agendas. In this article, we construct IPC research priorities, in order to draw attention to these critical research needs.
We developed a 4-step framework to identify IPC knowledge gaps from literature (narrative review). These gaps were then translated into research priorities and sent to two groups of European IPC experts for validation and critique through an online survey.
Seventy-nine publications were retrieved from the literature review, identifying fifteen IPC research gaps. Forty-four IPC experts, clustered in two groups, vetted them. The experts classified all research gaps as medium or high priority. Overall agreement between both groups was average (Kendall’s τ = 0.43), with strong alignment on the highest priorities: (i) the assessment of organizational, socio-economic, and behavioural barriers/facilitators for the implementation of IPC programmes, (ii) the impact of overcrowding on the spread of infections and (iii) the impact of infrastructural changes, at facility level, on the reduction of infections. Feedback from experts also identified an additional research gap on the interaction between the human and hospital microbiomes.
Conclusions
We formulated a list of sixteen research priorities and identified three urgent needs. Now, we encourage researchers, funding agencies, policymakers and relevant stakeholders to start addressing the identified gaps.
Introduction
Antimicrobial resistance (AMR) is a growing health issue with the potential to undermine modern medicine. In 2015, 670,000 infections with antibiotic-resistant bacteria were reported in Europe accounting for 33,000 deaths [ 1 ]. If AMR rates continue to increase and follow the predicted trend, 2.4 million people could die from resistant bacteria in Europe, North America and Australia between 2015 and 2050 [ 2 ]. Bacteria will always evolve resistance to antibiotics. Yet, this evolution can be hindered through a broad set of interventions combining surveillance, antibiotic stewardship, infection prevention and control (IPC) and ensuring appropriate access to antibiotics while maintaining efforts to bring new innovative antibiotics (or alternative therapeutics), diagnostics, and vaccines to the market [ 3 ]. All these interventions need to be applied in a One Health perspective, considering the interaction between humans, animals and the environment [ 4 ].
Each intervention area comes with knowledge gaps. It is important that these gaps are aggregated and communicated as research priorities so that both national and international actors may concert their efforts on critical needs, avoiding duplication and ensuring that the resulting evidence informs policies [ 5 ]. For the past few years, several initiatives have compiled strategic research agendas covering AMR. Among them, the Joint Programming initiative on Antimicrobial Resistance (JPIAMR, www.jpiamr.eu/ ) Strategic Research and Innovation Agenda (SRIA), launched in 2014 and updated in 2019, covers the full breadth of AMR research in a One Health context [ 6 ]. In 2014, EU’s Innovative Medicines Initiative (IMI, https://www.imi.europa.eu/ ) published a strategic research agenda compiling the innovation priorities for new medicines and other technologies across a range of therapeutic areas, including AMR [ 7 ]. Finally, in 2019, the One Health European Joint Programme (One Health-EJP, www.onehealthejp.eu/ ) published a strategic research agenda in the area of foodborne zoonoses and prevention of transmission of AMR in the food chain [ 8 ].
To assess the uptake of these agendas within Europe, the European Joint Action on Antimicrobial Resistance and Healthcare-Associated Infections (EU-JAMRAI, www.eu-jamrai.eu/ ) previously compared the AMR-related research priorities of seven participating countries with the comprehensive JPIAMR strategic research agenda [ 9 ]. This comparison revealed three potential gaps: (i) clinical trials efficiency, (ii) AMR in the food chain and (iii) IPC. Yet, the first two gaps are respectively covered by the IMI agenda (partially) and the One Health-EJP agenda, meaning that clear research directions are available for policymakers and funders. Regarding IPC, the JPIAMR SRIA outlines six research priorities of which three address IPC on a general level. We therefore believe that there is a gap to fill here and that specific IPC research priorities, validated by IPC experts, are a valuable addition to the existing multi-country strategic agendas.
Indeed, IPC can be one of the most cost-effective interventions to combat AMR. A recent report from the Organisation for Economic Co-operation and Development (OECD) estimated that promoting simple IPC measures such as hand hygiene could reduce by about 40% the AMR health burden [ 2 ]. Improving IPC would also help to reduce the multitude of non-resistant healthcare-associated infections (HCAI) causing millions of extra days in hospital [ 10 ], and representing a financial loss of several billion euros each year [ 11 ]. Despite their tremendous importance, many IPC measures are still based upon insufficient evidence (as assessed through the GRADE methodology), e.g. guidelines for preventing surgical site infections [ 12 ]. Additionally, there is a paucity of studies evaluating the cost-effectiveness of IPC interventions, which are needed to help countries determine how to best improve IPC. Research in the area is therefore needed and specific research priorities would help engaging and coordinating efforts to tackle urgent needs.
This study provides detailed IPC research priorities, validated and supported by IPC experts, which is a valuable tool for researchers, funding agencies and policymakers to fill knowledge and research gaps.
Narrative literature review and gap identification
To identify knowledge gaps, we performed a narrative literature review following a 4-step framework (Fig. 1 .A). First step consisted in a grey literature review to shape our analysis and identify broad “gap areas” for in-depth screening. Within second step, we screened PubMed for articles, published between 2012 and December 2018, highlighting knowledge gaps on each of the identified gap areas. Keywords used for the screening are mentioned on Fig. 1 .A. We selected articles based on their title, abstract and content, favouring meta-analyses or literature reviews. We specifically excluded literature on vaccines since research priorities have already been documented [ 13 , 14 ], and literature on viral/parasitic diseases since our focus here is bacterial resistance. Thirdly, we analysed the literature, determining commonalities between articles to identify important knowledge gaps. When relevant gaps were raised but without enough information in our dataset, additional PubMed searches were performed to gain understanding (enrichment process). Finally, we formulated a draft of research priorities based on all information retrieved from previous steps.
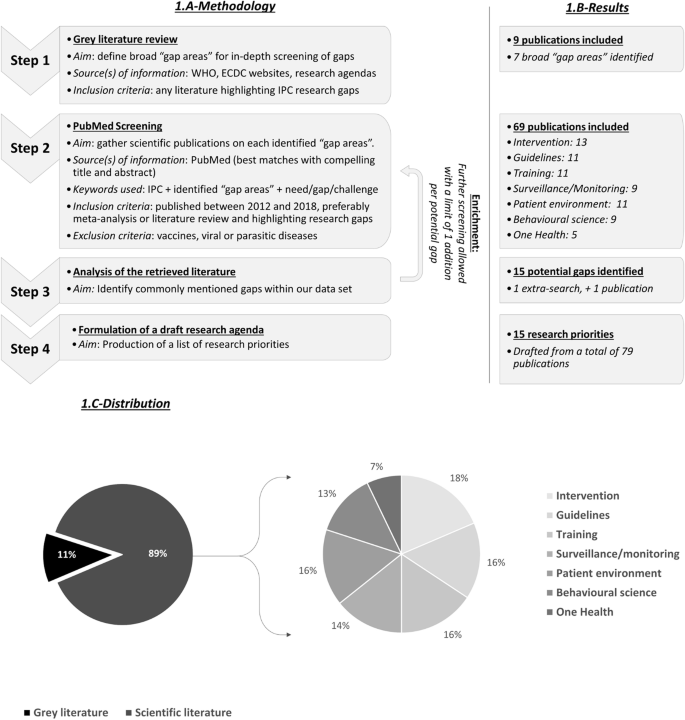
Overview of the 4-step narrative review framework used to build the draft IPC research priorities. a -Methodology. A 4-step framework was used to perform the literature review and build the draft research priorities. b -Results. Seventy-nine publications were retrieved from the literature review. c -Distribution. Overall, publications were fairly distributed between pillars. One Health literature may have been underrepresented
Survey and gap validation
To validate the aggregated research priorities and identify the most pressing needs, our draft research priorities were sent to European IPC experts for review through an online survey.
The survey was composed of three questions (Additional file 1 ). The first question assessed the urgency of each identified research priority. Six answers were available: (i) not a priority, (ii) low priority, (iii) medium priority, (iv) high priority, (v) critical priority and (vi) I don’t know. The second question asked for comments on each research priority, including suggestions for modifications of research priority. The third question was an open comment section where additional research priorities could be added.
The survey was sent to two groups of experts to assess inter-agreement. The first target group was composed of 18 IPC experts from 11 European countries. Experts from this group were selected on their publication record and/or involvement in specific organizations, making sure to have enough diversity (eleven European countries, one European organisation and one One-Health organisation). The second target group was composed of 33 members from the European Committee on Infection Control group (EUCIC, https://www.escmid.org/eucic/ ), a sub-group from the European Society for Clinical Microbiology and Infectious Diseases (ESCMID). The survey was distributed in February/March 2019 to the first group and in September 2019 to the second, upon agreement with the EUCIC Executive Board. There was no overlap between both groups.
Answers from first question were scored a value of 0 to 4 (0 corresponding to “not a priority” and 4 to “critical priority”). Based on this scoring system and answers from the survey, we calculated the average priority of each of the identified research needs and ranked them according to their priority level. This was done for both target groups and for merged groups. To assess inter-agreement between both groups and check whether they agree on the most urgent needs, we calculated (i) the linearly weighted Cohen’s kappa coefficient to measure agreement on classification (either no, low, medium, high or critical priority) and (ii) the Kendall rank correlation coefficient to measure agreement on ranking.
For critical analysis, comments from the second question were labelled as either (i) supportive, (ii) informative (when adding insight on a topic), (iii) critical or (iv) calling for modifications. Comments from the third question were reviewed individually to identify potentially missed research needs. Revision of the draft research priorities was considered when a modification or addition was requested by more than 5% of the total respondent population with requests coming from both groups.
Results of our 4-step review are available in Fig. 1 .B. We identified nine publications from grey literature in step 1: four reports from the European Centre for Disease Prevention and Control (ECDC), two from the World Health Organisation (WHO) and three international research agendas (JPIAMR, IMI and One Health-EJP’s). They allowed to define seven broad “gap areas” requiring in-depth screening: (i) IPC interventions, (ii) guidelines, (iii) training, (iv) surveillance/monitoring, (v) patient environment (facilities and staffing), (vi) behavioural science, and (vii) One Health. In step 2, we screened Pubmed and retrieved 69 relevant articles. Stratification of these 69 articles between gap areas is available in Fig. 1 .B. Enrichment process led to the inclusion of one additional publication on syndromic-based surveillance (surveillance based on available patient clinical information rather than microbiological data for early detection of infection), for a total of 70 publications evenly distributed across gap areas (Fig. 1 .C). Based upon grey and scientific literature, we proposed a draft list of 15 research priorities clustered in 7 categories (Table 1 , column 1).
This draft list was then vetted by two expert groups through an identical online survey. With the first group, composed of 18 selected European IPC experts, we achieved a response rate of 61% (11/18 respondents). For the second group, targeting the EUCIC members, we gathered 33 answers through a two-week open consultation on the EUCIC website.
Overall, there was strong support for the draft research priorities. All priorities were found on average to be of medium priority or higher (Table 1 , merged groups). Within the first target group, five research gaps emerged as high priority topics, nine as medium priority topics and only one as a low priority topic. In the second target group, only one research gap was considered as a high priority topic while all others ranked in the medium priority category. Overall agreement between both groups on their classification of research gaps appeared fair with a Cohen’s κ = 0.21. When looking at priority ranking, concordance between both groups is better with a 42.9% agreement as assessed by the Kendall rank correlation coefficient (z-score = 2.23, p -value = 0.026), indicating an overall average level of agreement. However, a strong alignment on the two most urgent needs was apparent when looking at both group results (Table 1 ). These critical gaps, 14 and 12, concern respectively (i) the assessment of the demographic, organizational, socio-economic and behavioural barriers/facilitators for the implementation IPC programmes, and (ii) the impact of overcrowding on the spread of HCAI and AMR. Additionally, priority 11 (impact of infrastructural changes at facility level on the reduction of HCAI and AMR) was also in the top five of both priority rankings (Table 1 , * mark).
We received 48 comments on our draft priorities. They included 17 comments considered as supportive, 22 as informative, five as critical, and four suggested modifications or rephrasing. All modifications were requested by less than 5% of the experts and thus were not included in the final list of priorities. Regarding critics, some experts (7%) questioned the need for additional IPC evidence. They argued that “high quality” evidence is hard to produce in the field due to methodological concerns and that evidence, while not “high quality”, may already be numerous enough. Other experts (5%) expressed that the highest priority is implementation rather than research.
Fourteen additional research priorities were proposed by experts but only one was mentioned enough to be included in the final list: “research on the interaction between the patient and hospital microbiome” which was mentioned by 9% of the experts and by both groups. According to this feedback, we proposed the final 16 research priorities mentioned in Table 1 .
The aim of this study was to generate IPC research priorities that could be used by policymakers, funders, and researchers to elucidate important IPC knowledge gaps. We constructed this list using an approach combining (i) a 4-step narrative literature review to identify knowledge gaps and (ii) a validation process with the help of two groups of IPC experts responding to a survey.
Survey results clearly demonstrated the need for research on IPC since several research gaps were scored as high priority areas in both target-groups. Results also support our attempt at building a list of important priorities with (i) no research gap bellow the medium priority (merged groups), (ii) mostly supportive and informative comments on research gaps, (iii) only few requests for additions. According to feedbacks, we only made one additional priority on the interaction between the human and hospital microbiomes (Table 1 , ** mark). It was deemed a suitable addition since requested by both expert groups and by 9.1% of the whole expert population. Furthermore, several studies have shown the role of the microbiota in preventing acquisition or expansion of HCAI [ 15 , 16 ], but with studies limited to murine models or clinical studies with small numbers of patients [ 17 ].
Through our analysis, three research gaps emerged as particularly important from both expert groups.
Assessment of demographic, organisational, socio-economic, and behavioural barriers/facilitators to implement effective IPC programmes
Over the past few years, socio-economic and behavioural sciences have greatly contributed to the fight against HCAI by identifying barriers and facilitators for the implementation of IPC measures. Commonly mentioned barriers include a lack of training/knowledge or awareness [ 18 , 19 ], and a lack of institutional resources, especially in low- and middle-income countries (LMIC) [ 19 , 20 ]. On the contrary, close relationships between healthcare workers [ 21 ], positive leadership and role modelling are often seen as facilitating factors [ 22 , 23 ]. While behavioural determinants have been identified, only few interventions have been proposed and tested to address them. An impactful intervention could be, for instance, the appointment of an IPC champion in institutions to help engage and educate colleagues [ 24 ]. Yet, limited quality of evidence failed to generate concrete recommendations. More research is therefore needed to assess innovative interventions and to test organisational frameworks that facilitate the implementation of IPC measures. Interestingly, this research gap is mentioned in the JPIAMR SRIA [ 6 ]. The JPIAMR could therefore help funding research in the area.
The impact of overcrowding (staff workload/availability, bed occupancy, …) on the spread of HCAI
There is growing evidence on the impact of overcrowding on HCAI transmission rate. Low staffing and increased workload have been associated with a higher risk of HCAI acquisition [ 25 , 26 ]. Regarding bed occupancy, the literature is still inconsistent [ 27 , 28 , 29 ]. These discrepancies could be explained by differences in study settings, monitoring outcomes but mostly by differences in methodologies and bed occupancy definition. More research is therefore needed but with appropriate occupancy parametrization [ 30 ]. Overall, there is still insufficient data to generate clear and robust breakpoint thresholds needed by policymakers and hospital managers to implement effective actions (worker/patient and patient/bed ratio for instance). Ideally, breakpoints should be defined for various healthcare settings (intensive care units, short or long stay wards, long-term care facilities) and country settings (high, medium, and low-resource settings). More studies on the impact of visitor frequency and patient movements on HCAI transmission rates would also be beneficial to explore new interventions.
Assessment of the impact of infrastructural changes at facility level on the reduction of infections and resistance
There are little data available on the impact of infrastructural changes on HCAI. In 2016, a meta-analysis concluded that a high density of hand-washing points and single-patient rooms could help reducing HCAI transmission rates in short-term care facilities [ 31 ]. However, these conclusions present some major limitations: (i) the small number of studies included in the meta-analysis, (ii) several studies were uncontrolled before and after intervention and (iii) several studies included in the meta-analysis were biased by bundle effects. More research in the area is therefore needed. However, as highlighted by experts, infrastructural changes are rarely considered as research opportunities. Ideally, IPC outcomes should be studied for any new healthcare facility or any facility remodelling. For instance, purchase of sinks, showers or bathtubs in healthcare institutions should include an analysis of evidence of how easily they can be disinfected. Placement and design of hand sanitisers should be based upon evidence on where healthcare personnel are most likely to use them.
Interestingly, not all IPC experts agreed that there is a need for additional IPC research, despite the dearth of high-quality evidence clearly displayed in many of IPC guidelines [ 12 , 32 ]. The main thrust of this feedback is that these experts would rather have the funds to implement IPC than to research them. We are sympathetic to this argument, as it is a priority to implement effective IPC measures. However, these funding sources are not necessarily competitive, as implementation funds would normally come for the healthcare budget and research funds from the research budget as well as multinational funding sources like JPIAMR. We believe that effective implementation can run in parallel to ongoing research, as it does in other fields.
Other feedback included the difficulty of producing “high quality” IPC research, as determined through the GRADE methodology, which considers randomized control trials (RCTs) as gold standard. While RCTs, especially clustered randomized trials, are appropriate to evaluate some individual elements of IPC programmes (surveillance for instance), they are often limited when assessing IPC programmes containing multiple interventions or relying on qualitative measurements. For example, RCTs are not suited to evaluate organisational or behavioural interventions which rely on measurements such as governance, commitment or compliance. RCTs may also be inappropriate for IPC interventions due to sample size, ethical limitations or even feasibility. However, GRADE does allow for other types of studies to generate high quality of evidence, like cohort, case-control, before-after and time series studies [ 33 ]. These study types would be more suited for the three urgent needs identified and could provide meaningful evidence. For instance, there are new developments in data and analytical technologies, offering the opportunity for observational studies to provide much stronger evidence. Propensity score matching, now, allows the assembly of two or more groups such that they appear to have been randomized to a comparator [ 34 ]. Improvement in data collection and data linkage techniques also make observational studies easier to undertake [ 35 ]. Powerful observational studies could help to provide evidence on barriers/facilitators for IPC measures implementation, thereby tailoring the design of innovative interventions. Implementation studies could then help testing these interventions. Contrary to local and time limited qualitative studies, implementation studies can match qualitative data with measures of success and process indicators over time, generating high quality evidence [ 36 , 37 ]. In the end, all of these study types could strengthen meta-analyses and provide gold standard evidence related to IPC . In some instances, methodological work is also needed to define appropriate parametrisation or standards to undertake research. This is notably the case for research on the link between bed occupancy and HCAI where different parametrisations of bed occupancy have led to conflicting findings.
There are limitations to our study, specifically the small number of survey respondents ( n = 44). However, this small number of respondents also reflects our strategy to target known European IPC experts/expert groups, which drastically reduces sample size. The average level of inter-agreement between both target-groups (Cohen’s κ = 0.21 and Kendall’s τ = 0.43) could also be interpreted as a lack of agreement on the most urgent needs. However, Cohen’s κ have been shown to be naturally lower when computing more than three categories (five in our survey) [ 38 ]. Inter-agreement on sorting could therefore be underestimated by our statistical test. Regarding inter-agreement on ranking, while the computer Kendall’s τ remains average, we clearly have a strong expert alignment on the most urgent needs with top two research needs being the same in both groups. Also, we are aware that differences in the number of respondents (11 versus 33) may impact agreement between both target groups. Another limitation is that the experts we interviewed were only from high-income countries. Although we did include LMICs in our literature analysis and proposed research priorities targeted toward them, LMICs were excluded from the validation process. A further study, focusing on LMICs, should be conducted to validate all our findings in this setting. Lastly, we had only one broad research gap focused on IPC measures for agriculture, livestock and the environment, given our panel of human health experts. More focused and detailed IPC research priorities on animals and environment would be beneficial.
Despite its limitations, we believe that this study will inform policymakers and funding agencies regarding important IPC research priorities.
IPC, as demonstrated through the OECD study, can be one of the most cost-effective interventions to guard against AMR. It is also essential to improve overall health outcomes at healthcare institutions. Therefore, it is remarkable that the research needs of IPC have been to date undervalued. IPC often pertains to tasks like handwashing and instrument disinfection, which may appear dull in comparison to applications for “ground-breaking” or “cutting-edge” innovation. It is imperative that this prejudice is removed so that wide-ranging, impactful IPC interventions can be researched, tested, costed, and optimally bundled.
In this study, we developed a list of sixteen IPC research priorities, supported by experts, including three urgent needs (Table 1 ). We encourage researchers, funding agencies, policymakers and relevant stakeholders to prioritise, fund, and research these identified gaps.
Availability of data and materials
The datasets used and analysed during the current study are available from the corresponding author on reasonable request.
Abbreviations
Antimicrobial Resistance
European Centre for Diseases Prevention and Control
European Joint Action on Antimicrobial Resistance and Healthcare-Associated Infections
European Society for Clinical Microbiology and Infectious Diseases
European Committee on Infection Control
Healthcare Associated Infection
Innovative Medicines Initiative
Infection Prevention and Control
Joint Programming initiative on Antimicrobial Resistance
Low and Middle-Income Countries
Organisation for Economic Co-operation and Development
One Health European Joint Programme
Strategic Research and Innovation Agenda
World Health Organisation
Cassini A, Högberg LD, Plachouras D, Quattrocchi A, Hoxha A, Simonsen GS, et al. Attributable deaths and disability-adjusted life-years caused by infections with antibiotic-resistant bacteria in the EU and the European economic area in 2015: a population-level modelling analysis. Lancet Infect Dis. 2019;19(1):56–66.
Article PubMed PubMed Central Google Scholar
Organisation for Economic Co-operation and Development (OECD). Stemming the Superbug Tide [Internet]. 2018. https://www.oecd-ilibrary.org/content/publication/9789264307599-en . Accessed May 2020.
Årdal C, Outterson K, Hoffman SJ, Ghafur A, Sharland M, Ranganathan N, et al. International cooperation to improve access to and sustain effectiveness of antimicrobials. Lancet. 2016;387(10015):296–307.
Article PubMed Google Scholar
SA ME, Collignon PJ. Antimicrobial Resistance: a One Health Perspective. Microbiology Spectrum. 2018;6:2.
Google Scholar
van Hengel AJ, Marin L. Research, innovation, and policy: an Alliance combating antimicrobial resistance. Trends Microbiol. 2019;27(4):287–9.
Joint Programming Initiative on Antimicrobial Resistance. Strategic Research and Innovation Agenda on Antimicrobial Resistance. 2019. https://www.jpiamr.eu/wp-content/uploads/2019/05/JPIAMR_SRIA_final.pdf . Accessed May 2020.
Innovative Medicines Initiative. The right prevention and treatment for the right patient at the right time. Strategic Research Agenda for Innovative Medicines Initiative. 2014. https://www.imi.europa.eu/sites/default/files/uploads/documents/About-IMI/research-agenda/IMI2_SRA_March2014.pdf . Accessed May 2020.
One Health European Joint Programme. Strategic Research Agenda. 2019. https://onehealthejp.eu/wp-content/uploads/2018/12/One-Health-EJP-Strategic-Research-Agenda.pdf . Accessed May 2020.
European Union Joint Action on Antimicrobial Resistance and Healthcare-Associated Infections (EU-JAMRAI). Gathering of national research priorities from at least five European countries and gap identification. https://eu-jamrai.eu/wp-content/uploads/2019/03/EUjamrai_MS9.1_Report_WP9_2019.02.28.pdf Accessed May 2020.
Cassini A, Plachouras D, Eckmanns T, Abu Sin M, Blank H-P, Ducomble T, et al. Burden of six healthcare-associated infections on European population health: estimating incidence-based disability-adjusted life years through a population prevalence-based Modelling study. PLoS Med. 2016;13(10):e1002150.
World Health Organization. The Burden of Health Care-Associated Infection Worldwide A Summary. 2010. https://www.who.int/gpsc/country_work/summary_20100430_en.pdf . Accessed May 2020.
Berríos-Torres SI, Umscheid CA, Bratzler DW, Leas B, Stone EC, Kelz RR, et al. Centers for Disease Control and Prevention guideline for the prevention of surgical site infection, 2017. JAMA Surgery. 2017;152(8):784–91.
Wellcome Trust and BCG. Vaccines to tackle drug resistant infections: An evaluation of R&D opportunities. 2019. https://vaccinesforamr.org/wp-content/uploads/2018/09/Vaccines_for_AMR.pdf . Accessed May 2020.
Hoelzer K, Bielke L, Blake DP, Cox E, Cutting SM, Devriendt B, et al. Vaccines as alternatives to antibiotics for food producing animals. Part 1: challenges and needs. Veterinary Research. 2018;49(1):64.
Gao Z, Kang Y, Yu J, Ren L. Human pharyngeal microbiome may play a protective role in respiratory tract infections. Genom Proteomics Bioinformatics. 2014;12(3):144–50.
Article Google Scholar
Yoon MY, Lee K, Yoon SS. Protective role of gut commensal microbes against intestinal infections. J Microbiol. 2014;52(12):983–9.
Pettigrew MM, Johnson JK, Harris AD. The human microbiota: novel targets for hospital-acquired infections and antibiotic resistance. Ann Epidemiol. 2016;26(5):342–7.
Marme G. Barriers and facilitators to effective tuberculosis infection control practices in Madang Province, PNG - A qualitative study. Rural Remote Health. 2018;18:4401.
PubMed Google Scholar
Le CD, Lehman EB, Nguyen TH, Craig TJ. Hand hygiene compliance study at a large central Hospital in Vietnam. Int J Environ Res Public Health. 2019;16(4):607.
Article PubMed Central Google Scholar
Mitchell KF, Barker AK, Abad CL, Safdar N. Infection control at an urban hospital in Manila, Philippines: a systems engineering assessment of barriers and facilitators. Antimicrob Resist Infect Control. 2017;6:90.
Troughton R, Mariano V, Campbell A, Hettiaratchy S, Holmes A, Birgand G. Understanding determinants of infection control practices in surgery: the role of shared ownership and team hierarchy. Antimicrobial Resistance Infection Control. 2019;8(1):116.
Saint S, Kowalski CP, Banaszak-Holl J, Forman J, Damschroder L, Krein SL. The Importance of Leadership in Preventing Healthcare-Associated Infection: Results of a Multisite Qualitative Study. Infect Control Hospital Epidemiol. 2015. 2010;31(9):901–7.
Gilbert GL, Kerridge I. The politics and ethics of hospital infection prevention and control: a qualitative case study of senior clinicians’ perceptions of professional and cultural factors that influence doctors’ attitudes and practices in a large Australian hospital. BMC Health Serv Res. 2019;19(1):212.
Zingg W, Holmes A, Dettenkofer M, Goetting T, Secci F, Clack L, et al. Hospital organisation, management, and structure for prevention of health-care-associated infection: a systematic review and expert consensus. Lancet Infect Dis. 2015;15(2):212–24.
Mitchell BG, Gardner A, Stone PW, Hall L, Pogorzelska-Maziarz M. Hospital staffing and health care–associated infections: a systematic review of the literature. Jt Comm J Qual Patient Saf. 2018;44(10):613–22.
Driscoll A, Grant MJ, Carroll D, Dalton S, Deaton C, Jones I, et al. The effect of nurse-to-patient ratios on nurse-sensitive patient outcomes in acute specialist units: a systematic review and meta-analysis. Eur J Cardiovasc Nurs. 2017;17(1):6–22.
Ahyow LC, Lambert PC, Jenkins DR, Neal KR, Tobin M. Bed occupancy rates and hospital-acquired Clostridium difficile infection: a cohort study. Infect Control Hosp Epidemiol. 2013;34(10):1062–9.
Abir M, Goldstick J, Malsberger R, Setodji CM, Dev S, Wenger N. The Association of Inpatient Occupancy with hospital-acquired Clostridium difficile infection. J Hosp Med. 2018;13(10):698–701.
Schwab F, Meyer E, Geffers C, Gastmeier P. Understaffing, overcrowding, inappropriate nurse:ventilated patient ratio and nosocomial infections: which parameter is the best reflection of deficits? J Hosp Infect. 2012;80(2):133–9.
Article CAS PubMed Google Scholar
Goldstein ND, Ingraham BC, Eppes SC, Drees M, Paul DA. Assessing occupancy and its relation to healthcare-associated infections. Infect Control Hosp Epidemiol. 2017;38(1):112–4.
Stiller A, Salm F, Bischoff P, Gastmeier P. Relationship between hospital ward design and healthcare-associated infection rates: a systematic review and meta-analysis. Antimicrob Resist Infect Control. 2016;5:51.
Centers for Disease Control and Prevention. Guidelines for the Prevention of Intravascular Catheter-Related Infections. 2017. https://www.cdc.gov/infectioncontrol/pdf/guidelines/bsi-guidelines-H.pdf . Accessed May 2020.
Schünemann H, Brożek J, Guyatt G, Oxman a, editor(s). Handbook for grading the quality of evidence and the strength of recommendations using the GRADE approach. GRADE Working Group. 2013. https://gdt.gradepro.org/app/handbook/handbook.html . Accessed May 2020.
Austin PC. An introduction to propensity score methods for reducing the effects of confounding in observational studies. Multivariate Behav Res. 2011;46(3):399–424.
Gilmartin-Thomas JF, Liew D, Hopper I. Observational studies and their utility for practice. Aust Prescr. 2018;41(3):82–5.
Szymczak JE. Beyond barriers and facilitators: the central role of practical knowledge and informal networks in implementing infection prevention interventions. BMJ Qual Saf. 2018;27(10):763.
Clack L, Zingg W, Saint S, Casillas A, Touveneau S, da Liberdade JF, et al. Implementing infection prevention practices across European hospitals: an in-depth qualitative assessment. BMJ Qual Saf. 2018;27(10):771.
Sim J, Wright C. The kappa statistic in reliability studies: use, interpretation, and sample size requirements. Phys Ther. 2005;85:257–68.
Download references
Acknowledgments
We thank Patriq Fagerstedt, from the Swedish Research Council, for critical reading. We are also grateful to Evelina Tacconelli and Nico Mutters, from the EUCIC group, and to the whole EUCIC advisory board for distributing our survey on the EUCIC website. Finally, we would like to thank all the IPC experts who kindly took time to complete our survey, including all the 18 experts from the first target group and the 33 experts from the EUCIC group.
This work was supported by the European Commission and the Consumers, Health, Agriculture and Food Executive Agency (Chafea). It was conducted as part of the EU-JAMRAI Consortium. The full membership list can be viewed at https://eu-jamrai.eu/about-us-partners-2/ .
EU-JAMRAI (European Union Joint Action on Antimicrobial Resistance and Healthcare-Associated Infections) has received funding from the health programme of the European Union (2014–2020) under grant agreement N°761296 as well as through own financing by participating organizations. The views expressed in this article are those of the authors and do not represent the European Commission’s or any country’s official position. The funders provided support in the form of salaries for authors but did not have any additional role in the study design, data collection and analysis, decision to publish, or preparation of the manuscript.
Author information
Authors and affiliations.
University of Limoges, INSERM, CHU Limoges, RESINFIT, U1092, F-87000, Limoges, France
Yohann Lacotte & Marie-Cécile Ploy
Antimicrobial Resistance Centre, Norwegian Institute of Public Health, Oslo, Norway
Christine Årdal
You can also search for this author in PubMed Google Scholar
Contributions
All authors participated in the design of the study. YL performed the literature analysis. All authors participated in the survey preparation, YL was in charge of data collection and analysis. YL wrote the first draft of the paper. All authors have critically read and commented on draft versions of the report and approved the final version.
Corresponding author
Correspondence to Yohann Lacotte .
Ethics declarations
Ethics approval and consent to participate.
Not applicable.
Consent for publication
Competing interests.
The authors declare that they have no competing interests.
Additional information
Publisher’s note.
Springer Nature remains neutral with regard to jurisdictional claims in published maps and institutional affiliations.
Supplementary information
Additional file 1., rights and permissions.
Open Access This article is licensed under a Creative Commons Attribution 4.0 International License, which permits use, sharing, adaptation, distribution and reproduction in any medium or format, as long as you give appropriate credit to the original author(s) and the source, provide a link to the Creative Commons licence, and indicate if changes were made. The images or other third party material in this article are included in the article's Creative Commons licence, unless indicated otherwise in a credit line to the material. If material is not included in the article's Creative Commons licence and your intended use is not permitted by statutory regulation or exceeds the permitted use, you will need to obtain permission directly from the copyright holder. To view a copy of this licence, visit http://creativecommons.org/licenses/by/4.0/ . The Creative Commons Public Domain Dedication waiver ( http://creativecommons.org/publicdomain/zero/1.0/ ) applies to the data made available in this article, unless otherwise stated in a credit line to the data.
Reprints and permissions
About this article
Cite this article.
Lacotte, Y., Årdal, C., Ploy, MC. et al. Infection prevention and control research priorities: what do we need to combat healthcare-associated infections and antimicrobial resistance? Results of a narrative literature review and survey analysis. Antimicrob Resist Infect Control 9 , 142 (2020). https://doi.org/10.1186/s13756-020-00801-x
Download citation
Received : 15 June 2020
Accepted : 06 August 2020
Published : 24 August 2020
DOI : https://doi.org/10.1186/s13756-020-00801-x
Share this article
Anyone you share the following link with will be able to read this content:
Sorry, a shareable link is not currently available for this article.
Provided by the Springer Nature SharedIt content-sharing initiative
- Antimicrobial resistance
- Infection prevention and control
- Healthcare-associated infections
- Research priorities
Antimicrobial Resistance & Infection Control
ISSN: 2047-2994
- General enquiries: [email protected]
- Research article
- Open access
- Published: 15 December 2017
Knowledge and practices of nurses regarding nosocomial infection control measures in private hospitals in Sana’a City, Yemen
- Gamil Alrubaiee 1 ,
- Anisah Baharom 1 ,
- Hayati Kadir Shahar 1 ,
- Shaffe Mohd Daud 2 &
- Huda Omar Basaleem 3
Safety in Health volume 3 , Article number: 16 ( 2017 ) Cite this article
43k Accesses
11 Citations
1 Altmetric
Metrics details
In the Yemeni healthcare setting, basic infection control measures are necessary to reduce the rates of hospital-associated infections.
A descriptive cross-sectional study was conducted from April to May 2016. A non-probability sample of 100 nurses working in the private hospitals located in the capital city of Yemen was selected as study participants. A 45-item questionnaire was used to assess knowledge and practices regarding nosocomial infection control measures among the study subjects. The collected data were analyzed by SPSS (version 22.0).
The highest percentage of nurses were males (61.2%) and aging between 25 and above (71.8%) and had 3 years nursing diploma (60%), less than 5 years of employment in the hospitals (56.5%), relatively high training course about nosocomial infections (NIs) (64.7%), and working experience in infection control (78.8%). Most of the nurses (87%) had a fair level of knowledge, while only 4% of them had a good level of knowledge of preventive measures of nosocomial infections. The results also revealed that the majority of the nurses (71%) had fair practices about nosocomial infections whereas 26% of them had good practices and only 3% of them had poor practices.
The gaps in knowledge and practices regarding NI control measures indicate the need to establish a related health care policy regarding NIs and implement a regular training program to upgrade and refresh the nurses’ knowledge and practices regarding NI control measures.
Nosocomial infections (NIs), also known as a hospital-acquired infection, are defined as infections which are acquired after 48 h of patient admission. Such infections are neither present nor incubating prior to a patient’s admission to a given hospital. NIs represent a universally serious health problem and a major concern for the safety of both patients and the health care providers [ 1 , 2 , 3 , 4 ]. Although the incidence rate for nosocomial infection vary from country to country, at any given time, almost seven patients from developed countries to ten patients from developing countries out of each100 patients admitted to hospitals gain at least one kind of nosocomial infections [ 5 ]. In Yemen, data regarding NIs are few, but the prevalence rate of NIs, specifically surgical site infection, is high from time to time as it accounted for 8% in 2002 [ 6 ] and 34% in 2013 [ 7 ].
NIs have significant consequences on patients, their families, and the community as a whole. The most common consequences of NIs are increased morbidity, mortality, and length of hospitalization [ 4 , 8 ]. Such consequences contribute substantially to raise both the direct and indirect cost of the health care services, which result in additional costs to treat infected cases. Hence, such issue wastes the available resources which are not already enough, especially in developing countries [ 9 ].
Nurses are responsible for providing medications, dressing, sterilization, and disinfection. They are involved in more contact with patients than other health care workers (HCWs). Therefore, they are more exposed to various NIs [ 10 ]. Hence, nurses play a vital role in transmitting NIs, and their compliance with infection control measures seems to be necessary for preventing and controlling NIs [ 11 ]. Accordingly, they should be aware of how to prevent transmission of NIs and be knowledgeable of its potential risk to patients, other staff, and as visitors.
Although there are many previous cross-sectional studies which revealed that the levels of nurses’ knowledge and practices are relatively poor and insufficient [ 12 , 13 , 14 , 15 ], to the researcher’s best of knowledge, so far, no study has been conducted in Yemen which is the context of the current study. Therefore, this study aimed to identify gaps in nurses’ knowledge and practices regarding NI control measures in order to improve the current training courses and enhance future good practice.
This study aims to answer the following research questions:
What is the current level of knowledge of nurses regarding infection control measures?
What is the current level of practices of nurses regarding infection control measures?
Study design
A descriptive, cross-sectional design was used in this study.
Study setting
This study was carried out in two private hospitals in Sana’a, the capital city of Yemen. The study covered a period from April 2016 to May 2016. All nurses who were working in the selected private hospitals and involved in a direct contact with patients were invited to participate in this study.
A non-probability (purposive) sampling technique was used to select the study participants. A total of 100 nurses participated in this study and responded to the study questionnaire. However, only 85 questionnaires were filled completely and included in the final analysis.
Study instrument
A structured questionnaire was developed based on the World Health Organization (WHO) infection control guidelines [ 9 ]. The final questionnaire comprised 45 items related to knowledge and practice regarding NI control measures. It is divided into the following three sections:
Section 1 is related to the demographic information of the participants: age, gender, level of education, current position and duration of working, course training on NI control, and working experience in NI control measures.
Section 2 is related to knowledge and is divided into these two parts:
Knowledge of preventive measures of person-to-person transmission, which includes hand hygiene (5 items), personal protective equipment (5 items), and safe injection practices (4 items)
Knowledge of preventive measures of transmission from hospital environment, which involves routine hospital cleaning (4 items), safe waste handling and disposal (4 items), reprocessing of patient care equipment (4 items), and safe linen handling (4 items)
Section 3 is related to practices on NI control measures, and it consists of 15 items. This section is also subdivided into two parts: precautions to prevent NIs (9 items) and actual actions to prevent NIs (6 items).
Scoring system
The 30 items related to knowledge were assessed with “Correct,” “Incorrect,” and “I don’t know” options, while the practices was assessed using 15 scenario-based items with “Yes,” “No,” and “I don’t know” options. The reverse statement was corrected first and then a 0 score was given for each “incorrect” or “I don’t know” response; 1 score was given for each correct response. The maximum and minimum scores for each section vary based on the number of items in each section. Correct answers were calculated to obtain total scores for different infection control practices. A score of less than 50% was considered poor, 50–79% fair, and 80% and above was considered as good [ 16 ].
Validity and reliability
Content validity was obtained by three experts in infection control and prevention at hospitals and academic institutions. Their comments regarding the tool layout and format, relevance, accuracy, consistency, and scoring system were taken in consideration. Reliability of the tool items was tested using alpha Cronbach ( α ) test: section 2 of the tool = 0.81 and section 3 = 0.79 which considered acceptable.
The responses were recorded and analyzed using the statistical software (IBMSPSS), version 22.0. Descriptive statistics was applied (percent and number). A p value of ≤ 0.05 was taken as statistically significant.
Data collection
The questionnaire was distributed during the study period of April to May 2016. In-ward nurses from both hospitals were invited during regularly scheduled educational meetings to fill the questionnaire and had to return it back at the same time to avoid any non-response bias. A total of 100 questionnaire were distributed, 50 in each hospital. Only 85 out of the 100 distributed questionnaires were completed, and therefore, they were used in the final analysis.
Permission for this study was obtained from the Ethics Committee of both selected private hospitals (University of Science and Technology and Saudi German Hospital Sana’a). A written consent was also obtained from all eligible nurses before data collection.
Response rate
Out of 100 distributed questionnaires, 85 questionnaires were filled completely and included in the final analysis, thus making up a response rate of 85%.
Demographic details of the respondents
The results of the study showed that the majority of the nurses (61.2%) were males and they were the age group of 25 years and above (71.8%). Sixty percent of them had a 3-year diploma and 95.3% were staff nurse. Regarding duration of work, 56.5% of the participating nurses had less than 5 years of working experience. Around two thirds of the nurses (64.7%) had attended training courses, whereas 78.8% of them had working experience in infection control. Further details of the respondents’ demographic characteristics are presented in Table 1 .
Nurses’ knowledge on person-to-person infection control measures
The results of the study showed that the majority (72.9%) of the Yemeni nurses had a fair level of knowledge on hand hygiene, while almost above of the third (35.3%) had a good level of knowledge on PPE. Likewise, more than half of the nurses (67.1%) had a poor level of knowledge regarding safe injection practices. Further details are given in Table 2 .
Nurses’ knowledge on preventive measures of transmission from hospital environment
Regarding the preventive measures used in preventing transmission of infections from the hospital environment, the results revealed that more than half (52.9%) of the participants had a good level of knowledge on routine hospital cleaning and the majority (81.1%) of them had a fair level of knowledge on safe waste handling and disposal. It was also found that more than two thirds (82.8%) of the nurses had a fair level of knowledge on patient care equipment reprocessing, while above half (60%) of them had a poor level of knowledge of safe linen handling. More details are given in Table 3 .
Overall level of knowledge on different NI control measures
The results of the current study showed that the majority (87%) of the participants had a fair level of knowledge on the different NI preventive measures. However, 9% of them had a poor level of knowledge, and only 4% of them had a good level of knowledge on the different NI preventive measures. The results are illustrated in Fig. 1 .
The overall knowledge on different infection prevention and control measures
Level of nurses’ practices of different NI control measures
Based on the findings of the study in Table 4 , most of the nurses (74.1%) had good practices on actual actions utilized to prevent NIs, while 71.8% of them reported fair practices regarding precautions to prevent NIs. Further details are presented in Table 4 .
Overall level of practices on different NI control measures
Figure 2 indicates that the majority (71%) of nurses had fair overall practices, whereas 26% of them demonstrated good practices on different infection prevention and control measures.
The overall nurses’ practices on different infection prevention and control measures
NIs increase patients’ morbidity, mortality, the length of hospital stay, and treatment cost (Ginny [ 17 ]). Therefore, infection prevention and control are important to prevent the occurrence of NIs in health and hospital settings. During patients’ nursing care, it is thus of utmost importance for nurses to have the knowledge and appropriate practice of infection prevention and control measures. This study aimed to determine the level of nurses’ knowledge and practices regarding NIs.
Based on the results of the present study, the majority of the Yemeni participating nurses were 25 years old and above (71.8%). This result is similar to the result reported in previous studies conducted by Ginny Kaushal et al. [ 17 ] in India, Johnson et al. [ 18 ] in Nigeria, and Reda et al. [ 19 ] in Ethiopia. Furthermore, the results revealed that almost above half (61.2%) of the participants were male holding nursing diploma (60%), which corroborates what was reported by El-Sayed et al. [ 20 ] in regard to nursing diploma. However, our result is inconsistent with this previous study concerning the gender as El-Sayed et al. [ 20 ] reported that most of the study participants were females. Thus, why the males outnumbered the females in the current study could be explained from a cultural and religious perspective. In other words, in many Middle East countries, cultural and Islamic perspectives still prevent female nurses from working in the night shift or working in the male’s wards. Regarding years of employment in the hospital, about half of nurses (56.5%) were found to have less than 5 years of experience. This result is compatible with the result obtained by Fashafsheh et al. [ 16 ] who found that about (43.9%) of the participants had working experience of 5 years or less. Such result indicates that new employees seem to be more cooperative than senior ones to participate in research. The current study also revealed that most of the participants (64.7%) attended annual continuing education courses about infection control. This result is compatible with Ebied [ 21 ] who found that more than half of nurses attended infection control course and continuing education programs. In contrast, such result is inconsistent with the result reported by Fashafsheh et al. [ 16 ] who indicated that most (63.8%) of the nurses had no training course about NIs. This contrast in results could be due to the difference in setting and targeted group from study to another study. The difference in in-service training-related policy could also be another factor for this difference.
Regarding the level of nurses’ knowledge on different preventive measures of person-to-person infection transmission, our study showed that the majority (72.9%) of the participants had a fair level of knowledge of Hand hygiene. Almost two thirds of the participants were found to have fair (27.1%) and good (35.3%) levels of knowledge on personal protective equipment. The results also demonstrated that there were above half (67.1%) of the participants which had a poor level of knowledge and 32.9% of them had a fair level of knowledge; none of the participants had good knowledge on safe injection practices. For the nurses’ knowledge on different preventive measures of transmission from hospital environment, it was found that above half (52.9%) of the participants had a good level of knowledge of routine hospital cleaning and the majority (81.1 and 82.8%) had a fair level of knowledge on safe waste handling and disposal and patient care equipment reprocessing, respectively. However, above half of the Yemeni nurses (60%) had a poor level of knowledge of safe linen handling. In general, regarding the overall nurses’ knowledge on the different infection prevention and control measures, it was found to be fair (87%). It was even higher than what was found by Abdulraheem et al. [ 12 ] in Northern Nigeria and Shamaa and Talaat [ 15 ] in Egypt and Isara and Ofili [ 22 ] in the Federal Medical center. The knowledge level in these studies was (12.9, 10, and 37.7%), respectively. However, the level of knowledge in the current study is lower than what was found by Vaz et al. [ 23 ] and Labrague et al. [ 24 ], as it accounted for 90 and 89.7%, respectively. Such different levels of knowledge among nurses in these different studies might be due to the inadequacy of infection prevention and control training’ education, as it was found that those nurses who attended in-service training courses achieved a high knowledge scores [ 25 ]. In particular, the results highlighted the necessity to implement an in-service training course on infection control measures with more focusing on safe injection practices and safe linen handling because nurses had had poor knowledge in these two aspects.
Concerning the level of nurses’ practices regarding infection prevention and control measures, the present study indicates that the majority (71.8%) of the Yemeni nurses had a fair level of practices in regard with precautions to prevent NIs, while nearly a third of them had a good level of practices on these precautions. However, the study demonstrates that almost two thirds (74.1) of the nurses had a good level of practices on actual actions to prevent NIs. In general, the study revealed that the majority (71%) of the participants had a fair overall level of practices, whereas 26% of them had good overall practices regarding different NI prevention and control. This result is close to the result by Eskander et al. [ 25 ] which showed that the level of practice was more than 75%. However, it is less than what was obtained by Fashafsheh et al. [ 16 ] as it was 91.14%, but it is higher than what was found in many other studies [ 18 , 20 , 26 , 27 ] as this level of practice accounted for 20, 48.7, 55.3, and 57.5%, respectively. This discrepancy in results could be due to the difference in participants’ attitudes towards utilizing infection control measure methods. It could also be either due to the difference in the operational definition of the good practice from one study to another study or due to the difference in knowledge of the nurses regarding infection prevention and control. Furthermore, although the nurses showed a fair level of practices about the precautions that should be used to prevent NIs, they demonstrated a good level of practices in the actual actions as a way to prevent NIs during the daily activities. This could be an indication of the existing contrast between theory and practice, which reflects the need for linking theoretical to practical aspects in curriculum addressing infection control measures.
Limitations
Our study has several limitations, which should be addressed for future research. The study was exclusive to private hospitals and nurses, and it used purposive sampling method. Therefore, generalizability of the results needs to be taken with caution. The selection bias also might exist in this study. Furthermore, our study determined the self-reported practices, but how nurses are translating these practices in the real clinical practices needs further investigation.
Based on the findings of this study, it could be concluded that the majority of the Yemeni nurses had fair knowledge and practices regarding NI control measures. Therefore, future research should focus on improving knowledge and practices among nurses through educational intervention, during either their training courses or in-service refresher courses by assessing their knowledge and practices before and after intervention. Further studies involving both public and private hospitals are also recommended.
Abbreviations
Statistical Package for the Social Sciences
- Nosocomial infections
World Health Organization
Iliyasu G, Dayyab FM, Habib ZG, Tiamiyu AB, Abubakar S, Mijinyawa MS, et al. Knowledge and practices of infection control among healthcare workers in a tertiary referral center in North-Western Nigeria. Ann Afr Med. 2016;15(1):34.
Article PubMed PubMed Central Google Scholar
Kamunge EW. Exploring knowledge, attitudes and practices of registered nurses regarding the spread of nosocomial infections, in health and medical sciences. 2013, Seton Hall University Dissertations and Theses (ETDs). New Jersey; 1865.
WHO. WHO guidelines on hand hygiene in health care. Geneva: WHO; 2010. ISBN, 978(92), 4
Google Scholar
Allegranzi B, et al. Report on the burden of endemic health care-associated infection worldwide. Geneva: World Health Organization; 2011.
WHO. Health care-associated infections fact sheet. World Health Organization; 2014.
Raja’a YA, Salam AR, Salih YA, Salman MS, Al-Baseer LS, Al-Kirshi NA, et al. Rate and risk factors of surgical site infections with antibiotic prophylaxis. Saudi Med J. 2002;23(6):672–4.
PubMed Google Scholar
Nasser AM, Zhang X, Yang L, Sawafta FJ, Salah B. Assessment of surgical site infections from signs & symptoms of the wound and associated factors in public hospitals of Hodeidah City, Yemen. Int J Appl. 2013;3(3):101–10.
Samuel S, Kayode O, Musa O, Nwigwe G, Aboderin A, Salami T, et al. Nosocomial infections and the challenges of control in developing countries. Afr J Clin Exp Microbiol. 2010;11(2)
Ducel, G., Fabry, J., & Nicolle, L. (2002). Prevention of hospital acquired infections: a practical guide. Prevention of hospital acquired infections: a practical guide(Ed. 2).
Shinde MB, Mohite VR. A study to assess knowledge, attitude and practices of five moments of hand hygiene among nursing staff and students at a tertiary care hospital at Karad. Int J Sci Res. 2014;3(2):311–21.
Sarani H, Balouchi A, Masinaeinezhad N, Ebrahimitabs E. Knowledge, attitude and practice of nurses about standard precautions for hospital-acquired infection in teaching hospitals affiliated to Zabol University of Medical Sciences (2014). Glob J Health Sci. 2016;8(3):193.
Article Google Scholar
Abdulraheem I, Amodu M, Saka M, Bolarinwa O, Uthman M. Knowledge, awareness and compliance with standard precautions among health workers in north eastearn Nigeria. J Community Med Health Edu. 2012;2(3):1–5.
Alwutaib A, Abdulghafour Y, Alfadhli A, Makboul G, El-Shazly M. Knowledge and attitude of the physicians and nurses regarding blood borne infections in primary health care, Kuwait. Greener J Med Sci. 2012;2(4):107–14.
Amin T, Al Wehedy A. Healthcare providers’ knowledge of standard precautions at the primary healthcare level in Saudi Arabia. Healthcare Infect. 2009;14(2):65–72.
Shamaa E, Talaat E. Developing a control action plan for infection prevention at the endoscopy unit. Int J Acad Res. 2010;2(4):218–27.
Fashafsheh I, Ayed A, Eqtait F, Harazneh L. Knowledge and practice of nursing staff towards infection control measures in the Palestinian hospitals. J Educ Pract. 2015;6(4):79–90.
Kaushal G, Doke P, Shah A, Verma V. An analysis of knowledge, attitude and practices regarding standard precautions of infection control and impact of knowledge and attitude of ICU nurses on self-reported practices of infection control. Int J Res Found Hosp Healthcare Admin. 2015;2:79–85.
Johnson O, Asuzu M, Adebiyi A. Knowledge and practice of universal precautions among professionals in public and private health facilities in Uyo, Southern Nigeria—a comparative study. Ibom Med. 2013;5(1):9–19.
Reda AA, Fisseha S, Mengistie B, Vandeweerd J-M. Standard precautions: occupational exposure and behavior of health care workers in Ethiopia. PLoS One. 2010;5(12):e14420.
Article CAS PubMed PubMed Central Google Scholar
El-Sayed M, Gomaa M, Abdel-Aziz M. Nurses’ knowledge and practice for prevention of infection in burn unit at a university hospital: suggested nursing guidelines. J Nurs Health Sci. 2015;4(4):62–9.
Ebied, E. M. a. E. Impact of a blood-borne diseases prevention program on compliance with infection control standard precautions among nurses in family health centers. Egypt: Elfayoum Governorate; 2011.
Isara A, Ofili A. Knowledge and practice of standard precautions among health care workers in the Federal Medical Centre, Asaba, Delta State, Nigeria. Niger Postgrad Med J. 2010;17(3):204–9.
CAS PubMed Google Scholar
Vaz K, McGrowder D, Alexander-Lindo R, Gordon L, Brown P, Irving R. Knowledge, awareness and compliance with universal precautions among health care workers at the University Hospital of the West Indies, Jamaica. Int J Occup Environ Med. 2010;1(4):171–81.
Labrague LJ, Rosales RA, Tizon MM. Knowledge and compliance of standard precautions among student nurses. Int J Adv Nurs Stud. 2012;1(2):84–97.
Eskander HG, Morsy WY, Elfeky HA. Intensive care nurses’ knowledge & practices regarding infection control standard precautions at a selected Egyptian cancer hospital. Int J Adv Nurs Stud. 2013;1(2):84–97.
Taneja J, BibhaBati M, Aradhana B, Poonam L, Vinita D, Archana T. Evaluation of knowledge and practice amongst nursing staff toward infection control measures in a tertiary care hospital in India. Can J Infect Control. 2008;24(2):104–7.
Teshager, F. A., Engeda, E. H., & Worku, W. Z. . Knowledge, practice, and associated factors towards prevention of surgical site infection among nurses working in Amhara regional state referral hospitals, Northwest Ethiopia. Surg Res Pract. 2015;2015.
Download references
Acknowledgements
We would like to thank all the nurses who willingly agreed to participate in this study as well as to those who helped us in the data collection, particularly the head of nursing departments in the selected hospitals. Also, we wish to thank the managers of the private hospitals for their agreement, cooperation, and assistance.
This study has not received any types of grants to support.
Availability of data and materials
As stated in the respondents’ consent, the data used in the current study is confidential and cannot be publicly shared but is available from the corresponding author on a reasonable request.
Author information
Authors and affiliations.
Department of Community Health, Faculty of Medicine and Health Sciences, Universiti Putra Malaysia, Serdang, Malaysia
Gamil Alrubaiee, Anisah Baharom & Hayati Kadir Shahar
Department of Foundations of Education, Faculty of Educational Studies, Universiti Putra Malaysia, Serdang, Malaysia
Shaffe Mohd Daud
Department of Community Medicine and Public Health, Faculty of Medicine and Health Sciences, University of Aden, Aden, Yemen
Huda Omar Basaleem
You can also search for this author in PubMed Google Scholar
Contributions
GGA, AB, HKS, SMD, and HOB were involved in the notion and the study design. GGA was responsible for the data collection and analysis. All the authors contributed in the data interpretation, reviewing and drafting the manuscript, and approved the final manuscript.
Corresponding author
Correspondence to Gamil Alrubaiee .
Ethics declarations
Ethics approval and consent to participate.
Ethical approval was obtained from the Ethics Committee of University of Science and Technology hospital and Saudi German Hospital Sana’a, where data were collected. Written consent using respondents’ sheet and consent was also obtained from all eligible participants prior to conducting this study.
Consent for publication
Not applicable
Competing interests
The authors declare that they have no competing interests.
Publisher’s Note
Springer Nature remains neutral with regard to jurisdictional claims in published maps and institutional affiliations.
Rights and permissions
Open Access This article is distributed under the terms of the Creative Commons Attribution 4.0 International License ( http://creativecommons.org/licenses/by/4.0/ ), which permits unrestricted use, distribution, and reproduction in any medium, provided you give appropriate credit to the original author(s) and the source, provide a link to the Creative Commons license, and indicate if changes were made. The Creative Commons Public Domain Dedication waiver ( http://creativecommons.org/publicdomain/zero/1.0/ ) applies to the data made available in this article, unless otherwise stated.
Reprints and permissions
About this article
Cite this article.
Alrubaiee, G., Baharom, A., Shahar, H.K. et al. Knowledge and practices of nurses regarding nosocomial infection control measures in private hospitals in Sana’a City, Yemen. Saf Health 3 , 16 (2017). https://doi.org/10.1186/s40886-017-0067-4
Download citation
Received : 18 October 2017
Accepted : 28 November 2017
Published : 15 December 2017
DOI : https://doi.org/10.1186/s40886-017-0067-4
Share this article
Anyone you share the following link with will be able to read this content:
Sorry, a shareable link is not currently available for this article.
Provided by the Springer Nature SharedIt content-sharing initiative
- Hospital-acquired infections
- Hospital-associated infections
- Cross infection
Safety in Health
ISSN: 2056-5917
- Submission enquiries: [email protected]
- General enquiries: [email protected]
Academia.edu no longer supports Internet Explorer.
To browse Academia.edu and the wider internet faster and more securely, please take a few seconds to upgrade your browser .
Enter the email address you signed up with and we'll email you a reset link.
- We're Hiring!
- Help Center
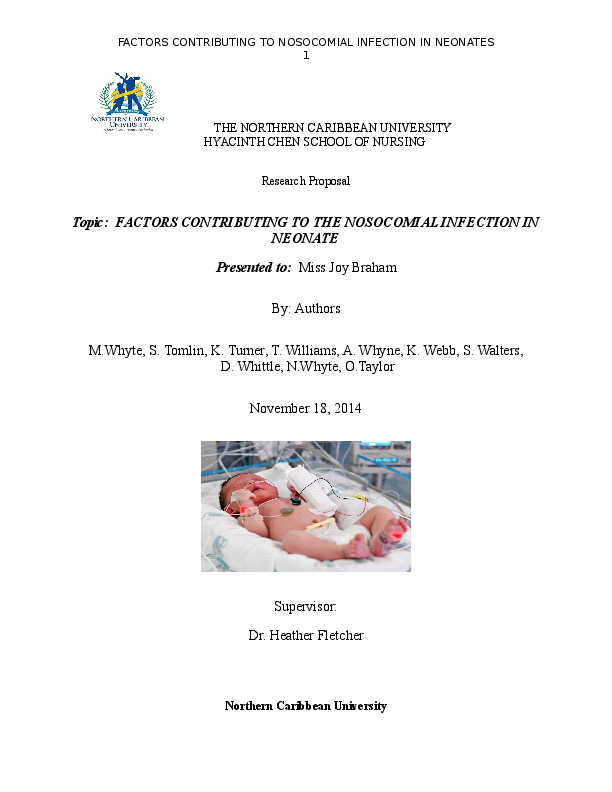
Topic: FACTORS CONTRIBUTING TO THE NOSOCOMIAL INFECTION IN NEONATE Presented to: Miss Joy Braham

Related Papers
International Journal of Infectious Diseases
Genevieve Zipp
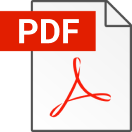
Zohair Abbas
Aladeen Alloubani , Tina Joyce
Marzouk elkhawaga
Journal of Perinatology
Angela Hoyos
Anjali Joseph
Seminars in Perinatology
Katheleen Hawes
Asmaa Elsemin
Stunning Spirit
RELATED PAPERS
Inonge Mubita
Journal of perinatology : official journal of the California Perinatal Association
Gary Darmstadt
The Lancet Infectious Diseases
Fatma Sefaan
Journal of Nursing Care Quality
Annemieke Brouwer , Agnes van Den Hoogen
Journal of Hospital Infection
Agnes van Den Hoogen
Mustafa Esmaio , buyiswa lizzie Sithole , Adebola Olayinka
Thea van de Mortel
NUR HIDAAYAH
Journal of Clinical Microbiology
Krishna Singh
Australian critical care : official journal of the Confederation of Australian Critical Care Nurses
Jacqueline Taylor , Kenneth Tan
fakruddin dafa
Nziki Nana Tadfor
Journal of Critical Care Nursing
Mansoureh Ashghali Farahani
Riccardo Pfister
Frontiers in Pediatrics
Noraida Ramli , Maziah Mahmood
Hadi Sedigh
Stephania Mercado
W. van der Zwet
Texila International Journal
Hyun-Bo Seo
Texila International Journal of Public Health
Acta Paediatrica
Bert Smit , J. Wielenga
W. van der Zwet , Ruurd Van Elburg , Christina Vandenbroucke-Grauls , Anneke Cranendonk
Ruurd Van Elburg
American Journal of Infection Control
Abdulaziz Binsaeed
Jeroen Stoof
American journal of infection control
Holly Ruch-Ross
Texila International Journal of Nursing
Infection Control and Hospital Epidemiology
Guilherme Santoro-lopes
Amanda Ullman
Christopher McCabe
ESTIMA, Braz. J. Enterostomal Ther.
ESTIMA Brazilian Journal of Enterostomal Therapy
Australian Critical Care
Lucy Maurice
Esther Daniel , Ruhee Mir
BMC Infectious Diseases
Elizabeth McInnes
- We're Hiring!
- Help Center
- Find new research papers in:
- Health Sciences
- Earth Sciences
- Cognitive Science
- Mathematics
- Computer Science
- Academia ©2024

An official website of the United States government
The .gov means it’s official. Federal government websites often end in .gov or .mil. Before sharing sensitive information, make sure you’re on a federal government site.
The site is secure. The https:// ensures that you are connecting to the official website and that any information you provide is encrypted and transmitted securely.
- Publications
- Account settings
Preview improvements coming to the PMC website in October 2024. Learn More or Try it out now .
- Advanced Search
- Journal List
- Front Public Health
Economic Analysis of the Prevention and Control of Nosocomial Infections: Research Protocol
Eric nguemeleu tchouaket.
1 Department of Nursing, Université du Québec en Outaouais, Gatineau, QC, Canada
Sylvain Brousseau
Kelley kilpatrick.
2 Ingram School of Nursing, McGill University, Montreal, QC, Canada
Sandra Boivin
3 CISSS des Laurentides, St.Jerome, QC, Canada
Bruno Dubreuil
4 Institut de Cardiologie-Montreal Heart Institute, Université de Montréal, Montreal, QC, Canada
Catherine Larouche
5 Department of Nursing, Centre Intégré Universitaire de Santé et de Services Sociaux du Saguenay–Lac-Saint-Jean, Saguenay, QC, Canada
Natasha Parisien
6 Department of Biomedical Risks and Occupational Health, Institut National de Santé Publique du Québec, Quebec, QC, Canada
Carl-Ardy Dubois
7 School of Public Health, University of Montréal, Montreal, QC, Canada
Astrid Brousselle
8 School of Public Administration, University of Victoria, Victoria, BC, Canada
Roxane Borgès Da Silva
Associated data.
Background: Nosocomial infections (NIs) are among the main preventable healthcare adverse events. Like all countries, Canada and its provinces are affected by NIs. In 2004, Ministry of Health and Social Services (MSSS) of Quebec instituted a mandatory surveillance NI program for the prevention and control (NIPC) in the hospitals of the province. One target of the MSSS 2015–2020 action plan is to assess the implementation, costs, effects, and return on investment of NIPC measures. This project goes in the same way and is one of the first major studies in Canada to evaluate the efficiency of the NIPC measures. Three objectives will be pursued: evaluate the cost of implementing clinical best practices (CBPs) for infection control; evaluate the economic burden attributable to NIs; and examine the cost-effectiveness of the NIPC by comparing the costs of CBPs against those of NIs.
Methods: This project is based on an infection control intervention framework that includes four CBPs: hand hygiene; hygiene and sanitation; screening; and additional precautions. Four medical and surgical units in two hospitals (nonUniversity, University) in the province of Quebec will be studied. The project has four components. Component 1 will construct and content validate an observation grid for measuring the costs of CBPs. Component 2 will estimate CBP costs via 2-week prospective observations of health workers, conducted every 2 months over a 1-year period. Component 3 will evaluate, through a matched case-control study, the economic burden of the four most monitored NIs in Quebec ( C-difficile , MRSA, VRE, and CPGNB). Archival patient data will be collected retrospectively. Component 4 will determine the optimal breakeven point for CBPs associated with NIPC.
Discussion: This project will produce evidence of the economic analysis of NIPC and give health stakeholders an overview of NIPC cost-effectiveness. It will meet the objectives of the Canadian Patient Safety Institute and the MSSS action plan to analyze the efficiency of NIPC preventive measures. To our knowledge, this is the first such exercise in Quebec and Canada. It will provide governments with a decision support tool through a major empirical study that could be replicated nationally to capture the financial benefits of NIPC.
In recent years, healthcare institutions in Organisation for Economic Co-operation and Development (OECD) countries have been under cultural, regulatory, and, especially, economic pressures. They are confronted with the acceleration of health expenditures ( 1 , 2 ), problems related to population aging and to medical and technological advances ( 3 , 4 ), and rising expectations of patients regarding care quality and safety ( 3 ). Over this time, standards for governance, leadership, drug management, and infection prevention, and control have been imposed on health facilities ( 5 ). The quality and safety of inpatient care is a major issue in health systems. As a result, interest in matters of safety has been growing in recent years ( 6 – 11 ). Health professionals, decision-makers, the public, and scientists are all deeply preoccupied with analyzing patient safety and infection control in healthcare facilities ( 12 ).
Nosocomial infections (NIs) are infections acquired during an episode of care in a healthcare facility ( 13 ). They are a significant burden on patients, healthcare organizations, and the public health system ( 14 , 15 ). They lead to increases in care complications, mortality, morbidity, and lengths of hospital stays ( 16 – 19 ). They also generate higher human, material, and financial costs for health facilities ( 16 – 18 ). NIs are one of the main preventable health care adverse events ( 20 ). Like every country in the world, Canada is affected by NIs. In 2013, the Public Health Agency of Canada reported that more than 200,000 patients contract nosocomial infections each year, resulting in more than 8,000 deaths. Since 1997, mortality rates due to Clostridium difficile-associated diarrhea (CDAD) have tripled in Canada. From 1995 to 2009, the rate of NIs caused by methicillin-resistant Staphylococcus aureus (MRSA) bacteremia increased by more than 1,000% ( 21 ). In addition, the incidence of vancomycin-resistant enterococcus (VRE) in Canada increased significantly from approximately.02 cases per 10,000 patient days in 1999 to approximately.68 cases per 10,000 patient days in 2011 ( 22 ).
The province of Quebec is no exception to this reality. To address this persistent problem, the Ministry of Health and Social Services (MSSS) implemented, in response to the 2004 CDAD crisis, standardized mandatory surveillance of NIs in all hospitals in the province. This surveillance is based on a reference program for nosocomial infection prevention and control (NIPC program), which has become the mainstay for healthcare institutions in managing risks, quality, and patient safety. The program is based on seven key actions: (1) surveillance of NIs and monitoring for emergent infectious problems; (2) development of policies, procedures, and support measures; (3) education and training; (4) evaluation; (5) communication and information; (6) outbreak management; and (7) risk management. Its effective implementation should lead to a reduction in NIs ( 23 ).
It is with this in mind that decision-makers in the health network wish to evaluate the implementation, costs, effects, and return on investment of the NIPC program. To this end, in target 22 of its 2015–2020 action plan ( 24 ) on the prevention and control of NIs for safe delivery of healthcare in the province of Quebec, the MSSS sets out the need to: “evaluate NIPC measures, taking into account the organizational model, the burden of disease, as well as their clinical and epidemiological impacts.” Action 1 of that same target stipulates the need to “assess the clinical and economic impacts of NIs, as well as the outcomes of measures to prevent and control these infections in both general and specialized hospitals” ( 25 ). This action by the MSSS is in line with the approach that has been observed since the last economic recession in 2008 in terms of controlling public spending.
The issue of infection prevention investment cost is undeniably very relevant and timely. Moreover, since 2010, Canada has shown great interest in assessing costs associated with adverse events and nosocomial infections ( 26 ). In fact, the Canadian Patient Safety Institute funded a project entitled The Economic Burden of Patient Safety to undertake research to assess the economic impact of adverse events and the potential benefits for patients and the healthcare system of reducing them ( 12 ). Meanwhile, the province of Quebec did not stand idly by. In 2011, the public health directors of the MSSS initiated a study on the economic benefits of public health interventions. This project, led by the Charles LeMoyne Hospital Research Centre under the direction of Professor Astrid Brousselle and Eric Tchouaket, was aimed at developing robust, economically sound arguments to demonstrate the efficiency (economic value) of certain public health programs in Quebec. The programs studied were chosen by public health directors of Quebec at their provincial round table. This is how the project on the economic analysis (EA) of the NIPC program of Quebec came into being. The present research project is part of the same strategy. This is the first major study in Quebec to evaluate the efficiency of an NIPC program. Several benefits are envisaged. On the clinical front, this project will inform healthcare providers and facility managers about the benefits of rigorous application of NIPC program measures, as well as about the costs of managing a patient who is either a carrier of, or infected with, an NI. On the political front, it will provide a strong evidence-based rationale for NIPC investment in Quebec and Canada. It will, thereby, help the MSSS achieve target 22 of its 2015–2020 action plan. On the research front, this project is a precursor to the development of EA studies on both public health and nursing interventions.
The main objective of the project is to evaluate the efficiency of an NIPC program by conducting an economic analysis of clinical best practices (CBPs) implemented. Three specific objectives will be pursued:
- Evaluate the cost of implementing CBPs associated with NIPC;
- Assess the economic burden attributable to NIs;
- Examine the cost-effectiveness of NIPC by comparing the cost of implementing CBPs against the costs of NIs.
Main Hypothesis
This research project is based on the hypothesis that proper implementation of measures related to clinical best practices for prevention and control of NIs is efficient. In other words, investing in these measures is both beneficial in terms of health and economically profitable.
Theoretical Framework
This project builds on the adverse-events prevention framework developed by Resar et al. ( 27 ) at the Institute for Healthcare Improvement in the United States, which advocates implementing “bundles” of three to five evidence-based clinical best practices (CBPs) in professional teams. These practices ensure healthcare providers can, together, provide safe care for their patients. This intervention framework supported the implementation, in Canadian and Quebec healthcare facilities, of infection prevention and control strategies and the deployment of Canadian ( 28 ) and Quebec ( 29 , 30 ) safe care campaigns. According to the Public Health Agency of Canada, the use of best practices for prevention would reduce the risk of contracting some NIs to near zero ( 21 ). The CBPs for NIs are subdivided into four essential measures: (1) hand hygiene; (2) hygiene and sanitation of surfaces and equipment; (3) screening on the admission of patients who are carriers or infected, in accordance with the protocols of the healthcare facility; and (4) application of additional precautions. Appendix 1 presents a diagram of the project framework based on the CBPs associated with NIPC.
i) Hand hygiene . Hand hygiene refers to the washing and disinfecting (hygienic and surgical) of hands, wrists, and forearms using water, soap, and hydroalcoholic solutions (HAS) at four specific times (see Appendix 2 ). This action extends from the initial wetting of the hands to the point where they are completely dry ( 31 ). The World Health Organization (WHO) estimates that hand hygiene could help reduce healthcare-associated infections by 50%.
ii) Hygiene and sanitation of surfaces and equipment . In Québec, a 2005 report from the MSSS committee studying NIPC, entitled D'abord, ne pas nuire [ First, Do No Harm ], stressed the importance of cleanliness and sanitation as one of the fundamental measures for infection prevention and control ( 32 ). Neglect of regular preventive cleaning and disinfection of surfaces and equipment creates a reservoir for the spread of microorganisms. Hygiene and sanitation activities must be carried out with appropriate frequency (daily, or several times daily), depending on the risk area ( 33 , 34 ).
iii) Screening on admission of patients who are carriers or infected . Screening is the systematic search, in persons with or at risk for NI, for any hitherto undetected condition or anomaly, based on screening algorithms ( Appendix 3 ). Screening techniques differ according to the type of NI. In general, screening involves performing clinical diagnosis and laboratory analysis. Any patient currently or previously hospitalized is considered at risk if he or she presents with signs and symptoms of infection. Analyses of stools or clinical specimens (for CDADs), blood, nasal smears, blood cultures, and laboratory tests can be used to detect NIs, in accordance with predefined surveillance protocols for each NI, in symptomatic or even asymptomatic patients ( 35 – 39 ).
iv) Additional precautions . Besides the three above-mentioned basic practices, additional precautions must be taken when an NI is declared. While they depend on the infection detected, they involve, among other things, implementing isolation measures and contact precautions for patients who are carriers or infected ( 40 ). In the event that a major outbreak of an NI is declared, CBPs must be applied intensively and additional care team meetings and resources added for as long as it continues ( 41 ).
An Operational Framework and Research Questions
Prior to embarking on an EA, it is important to understand that any interpretation of economic studies must consider three elements: the analytical perspective, the time horizon, and the allocation of healthcare resources and interventions, taking into account the prior condition of the patient ( 12 ). The analytical perspective determines what costs are included in the calculations. The study can be done from the patient, hospital, or societal perspective. For example, from a hospital perspective, medical costs will not include costs related to the patient post-discharge nor those related to lost productivity due to hospitalization. The time horizon defines the time frame for measuring medical costs. As well, the stage and severity of illness , comorbidities, risk factors, admitting diagnosis, and length of stay all influence the costs of care ( 12 , 42 , 43 ). Conducting an EA of an NIPC program thus involves examining questions about the quality of management and prevention, as well as about the safety of care. Thus, as Finkler (1993, 1996) states, the cost of managing quality takes into account the cost of investing in preventive measures and the costs associated with poor quality or with problems experienced ( 44 , 45 ). According to Finkler, a certain level of quality could be achieved by investing in prevention. Thus, there is a breakeven point, referred to as “the optimum,” above which prevention could increase with quality. Thus, based on Finkler's model (1993, 1996), an EA of the NIPC program, using CBPs, involves answering the following questions:
- What is the cost of investing in prevention through best clinical practices in NIPC?
- What are the costs of NIs?
- What is the optimal breakeven point for measuring return on investment when comparing prevention intervention costs against potential benefits?
Settings and Project Scope
Because NI incidence varies according to types of facilities and of patients present in hospitals ( 23 ), and in keeping with the standards for reporting statistics by region in the documents of Provincial NI Surveillance Group (SPIN) of Quebec ( 35 – 38 ), this project will be conducted in two Quebec hospitals, one nonUniversity and the other University affiliated. As such, one of the selected sites will be a nonUniversity hospital included in the CISSS des Laurentides (integrated health and social services centre for the Laurentians region), and the other, a University-affiliated hospital included in the CIUSSS (integrated University centre for health and social services) of the Saguenay–Lac-Saint-Jean region.
Four reasons prompted the choice of these two regions. First, these regions are de facto part of this project, given the involvement of researchers and professionals with NIPC expertise affiliated with universities and health centers in these regions. Second, in the Laurentians region, the incidence rate of Clostridium difficile-associated diarrhea (CDAD) has been comparable to the provincial average over the past 5 years ( 35 ). The Saguenay–Lac-Saint-Jean region has an incidence rate of CDAD slightly below the provincial average for the same period. Third, the Laurentians region has an incidence rate of nosocomial VRE colonization higher than the provincial average over the past 5 years (with the exception of 2016–2017), while, over the same period, this rate has been significantly lower than the provincial average in the Saguenay–Lac-Saint-Jean region ( 36 ). Lastly, the Laurentians region is a CISSS while the Saguenay–Lac-Saint-Jean region is a CIUSSS. These are two different case types that will help cover a fairly broad spectrum of costs in Quebec hospitals. A nonUniversity hospital will be selected from the CISSS, and a University-affiliated hospital will be selected from the CIUSSS. It is important to note that two co-investigators (Sandra Boivin and Catherine Larouche) will facilitate obtaining the consent and participation of the two hospitals, each in their respective regions. Moreover, a similar project has been submitted to the multicenter ethics committee of the Laurentians CISSS with the involvement of the Saguenay–Lac-Saint-Jean CIUSSS.
Project Components
The proposed project has four components that each provides deliverables:
Component 1: Construction and Content Validation of an Observation Grid for Measuring the Costs of CBPs Associated With NIPC
The best clinical practices that will be studied are those described in guidelines for the prevention of CDAD, MRSA, VRE, and carbapenemase-producing Enterobacteriaceae (CPE, or CPGNB).
Methods : A “time-motion” observation grid ( 46 , 47 ) was constructed to measure the time taken (in seconds) by health workers to perform each CBP (hand hygiene, hygiene and sanitation, screening, and additional precautions). This grid was developed in accordance with the strategies and methods for measuring compliance in each of the CBPs recommended by the Public Health Institute (INSPQ) of Quebec ( 29 , 30 ). The grid was constructed according to the algorithm developed by the project team, which is presented in Appendix 2 . Using a Delphi approach, the grid will be validated by 18 experts (six field experts from CISSS des Laurentides, six field experts from CIUSSS du Saguenay–Lac-St-Jean, and four content experts). The field experts are NIPC professionals, microbiology and infectious disease specialists, as well as professionals in hygiene and sanitation. The content experts have been selected for their expertise in the field of NIPC. The degree of content validity of each section of the grid will be measured by means of the content validity index (CVI) by retrieving the percentage of statements rated 3 and 4 (number of statements rated 3 and 4/total number of statements in the grid) ( 40 – 50 ). A CVI ≥0.80 will show that the grid is acceptable and its content valid with minor corrections ( 49 ). If it is <0.80 in any section of the grid, that section will undergo major revision and then be resubmitted to the same experts for assessment. Referring to their previous comments and suggestions, they will be asked to reassess it, based on the previous ordinal scale, until a CVI ≥0.80 is obtained for each section of the observation grid. At least two rounds will be required.
Deliverable 1 : Production and validation of a standardized grid , which can be used in all general and specialized (medical and surgical) physical health services units in Quebec to measure the costs of the four practices: hand hygiene, hygiene and sanitation, NI screening, and additional precautions.
Component 2: Use of the Constructed and Validated Grid to Assess the Costs of the Four CBPs in a CISSS Hospital in the Laurentians Region and a University-Affiliated CIUSSS Hospital in the Saguenay–Lac-Saint-Jean Region
In each of the two hospitals , two units from the general and specialized (medical and surgical) physical health services will be randomly selected for the study. A prospective investigation ( 48 ) will be conducted in the four study units to collect information on CBPs through direct observation.
Methods: In line with CBP compliance assessment methods ( 29 , 30 ), a sample of practitioners, a sample of patient records, and a sample of patients will be selected for each type of CBP studied over the course of the year (April 1, 2019, to March 31, 2020).
For hand hygiene , in each of the four units of the two selected hospitals, three physicians, three nurses, three nursing assistants, and three patient attendants will be randomly selected from the day and evening shifts. During this period, for each hand hygiene opportunity, time taken (in seconds) and products used will be systematically recorded. For hygiene and sanitation , in addition to the above-mentioned practitioners, three hygiene and sanitation workers will also be randomly selected and observed during disinfection (daily and terminal) of the ordinary rooms and/or for additional precautions.
Each of the 15 workers will be shadowed by a research nurse observer duly trained for this purpose, for 3 h in their shift. Given the heavy volume and activity during the day, two out of three workers will be randomly selected during the day shift (10 during the day and five in the evening).
As recommended by INSPQ ( 29 , 30 ) for the assessment of CBP compliance, observations will be made during six 2-week observation periods conducted every 2 months over the course of 1 year.
A total of 15 × 6 = 90 workers (3 × 6 = 18 physicians, 18 nurses, 18 nursing assistants, 18 patient attendants, and 18 hygiene and sanitation workers) will be observed per unit during 1 year. Thus, for two units in one hospital, 180 workers will be observed during the year, i.e., 360 workers for the two hospitals. It is possible, due to random selection, that a worker may be observed more than one time during the year.
Practices related to additional precautions will be systematically observed when applied by the workers.
With regard to screening , as suggested by the INSPQ ( 29 , 30 ), in each 2-week observation period, to be conducted six times over the year, 15 clinical records of patients screened (at admission or during hospitalization) for MRSA, VRE, and CPGNB (i.e., five records for each of the three screened NIs) will be randomly selected from the total number of patient records screened in the two units of each hospital. In addition, five records with diagnostic tests for CDAD detection will also be randomly selected from the records of patients with diarrhea in the two units of each hospital. Thus, 20 files × 6 observation periods, or 120 patient files studied per hospital, will be reviewed during the year, i.e., 240 patient files for both hospitals in total. All screening tests and diagnostic tests (related to CDAD) performed on a selected patient will be noted. The screening and diagnostic testing algorithms for each NI ( 51 – 54 ) in Appendix 3 will be used as models. By associating a cost with each test, the total cost of patient screening will be calculated. An average annual cost will be estimated.
With regard to training and information sessions and any meetings that may be organized by the NIPC service of the hospital in the units studied, a dashboard will be maintained throughout the observation year to systematically record participants, their professional profiles, trainers, instructors, duration of meetings, and equipment used. Finally, one representative (e.g., from the central supplies service) from each hospital (two in total) will provide information on unit prices and depreciation for each of the products and materials used. Thus, in addition to the activities of NIPC nurses (the grid), awareness-raising and information campaigns, and training activities for health personnel in the unit, information will be collected at weekly, monthly, and annual meetings; management and coordination activities related to NIPC in the care units; evaluation activities; and investments made (as needed).
Deliverable 2 : An estimate of the average annual cost of each CBP (hand hygiene, hygiene and sanitation, screening, additional precautions) and the average annual overall cost of actions implemented for NICP (including training and information activities), whether during an outbreak period or not, in a teaching hospital and a non-teaching hospital. To this end, costs will be estimated on a 1-year horizon in Canadian dollars (CAD) and from a healthcare facility perspective (costs related only to hospital activities). The following cost indicators will be estimated (in days) per unit: the cost of an observation; the cost of a CBP; the cost per worker; and the overall cost of the four CBPs considered together in one unit. The cost of an observation will be calculated as follows: (Time spent [in minutes] on an observation × the hourly wage of the worker [in minutes) × 24 h + (Depreciated cost of the product or material used (in days) during the observation). The cost of a CBP (in days) will be the sum of the costs of the observations made. The overall cost will be the sum of CBP costs and costs related to meetings, training sessions, and awareness-raising campaigns. Sensitivity analyses will be carried out by varying the hourly wages of workers from minimum to maximum, according to their salary scales (lowest and highest salary scales). For physicians, the rate for a regular consultation for a patient at risk of infection will be applied.
Component 3: Assessment of the Economic Burden Attributable to the Occurrence of Nosocomial Infections in Two Quebec Hospitals
While the costs of NIs in healthcare facilities have been sufficiently analyzed in Western scientific literature ( 12 , 55 ), to our knowledge, there are currently no empirical studies in Quebec on the financial costs associated with the four NIs most closely monitored in the province (CDAD, MRSA, VRE, and CPGNB). Complementing the epidemiological surveillance carried out by SPIN, the results of Component 3 will provide decision-makers in the Quebec healthcare network with information on the health-related and economic benefits of strengthening the NIPC program.
Methods: Assessing the economic burden of NIs involves estimating, from the healthcare facilities perspective, the additional financial cost of each of the four Nis during a care episode. As such, it includes the additional financial costs attributable to the increase in overall care consumption, the extension of the length of stay, the consumption of drugs, and the increase in laboratory tests caused by the occurrence of an NI ( 56 ). This component is based on a matched case-control design of patients with an NI (colonized or infected patients: cases) with similar patients who have not contracted an NI (controls) for a given period of time. The advantage of using such a design is that it is suitable for infrequent diseases such as NIs, and it also allows comparisons to be made between similar groups that are differentiated only by the presence of an illness ( 57 ).
Population : Included: Patients 18 years of age and over hospitalized for at least 72 h in the four units between April 1, 2019, and March 31, 2020. A patient readmitted either for a new hospital stay or for a complication related to the initial admission will be considered a new patient. Patients will be followed for a maximum of 30 days on the unit. A case is a patient who has contracted one of the four NIs studied. A control is a similar patient who did not develop an NI during the same care episode. Matching : Propensity score matching will be used, followed by 1:1 nearest neighbor matching ( 58 – 60 ). As in D'Amour et al. ( 9 ) and Tchouaket et al. ( 56 ) the cases and controls will be matched according to age, sex, risk factors, admitting diagnoses, comorbidities, date of admission to the unit, and severity of illness on admission as measured by the Charlson index ( 61 ). Sample size : All cases of each of the NIs occurring between April 1, 2019, and March 31, 2020, in the care units of the two hospitals will be included in the study. For MRBs (MRSA, VRE, and CPGNB), a distinction will be made between colonized patients (diagnosed on admission) and patients infected in the hospital. Data source : Data from hospital archives containing information on the units studied will be used. A grid (see a model in Appendix 4 ) will be used to extract the following information: health status of a patient on admission; date of NI occurrence; date of discharge of the patient from the unit; care and services consumed during the period in the unit; medical procedures performed by physicians; interventions carried out on the patient (professional involved, date, time spent); drugs consumed; laboratory tests performed; and imaging services used.
Deliverable 3 : An estimate of the care and services consumption cost for each patient group (cases and controls) over a 1-year horizon and from a health facility perspective , and cost comparison between cases and controls to determine the financial burden (additional cost) generated by the occurrence of an NI . First, the time spent by nonphysician professionals to perform each intervention on a patient will be multiplied by the hourly wage of the professional in question to estimate the cost per nonphysician professional intervention per patient . Data on medical procedures performed for the benefit of a patient (according to the profile of the physician) will be collected, and, based on RAMQ and AP-DRG data, the medical (physician) costs per patient associated with each procedure will be estimated. Laboratory and medical imaging fees will be determined from the MSSS price list to estimate the cost of diagnostic tests per patient. Lastly, the prices of each drug consumed (based on actual drug costs at the pharmacy, without insurance deduction) will be used to estimate the cost of drugs consumed per patient . The cost of care and services consumed per patient will be the sum of the cost of nonphysician professional interventions received, the cost of medical procedures, the cost of diagnostic tests, and the cost of drugs consumed per patient . Second, the additional cost generated by the occurrence of an NI will be determined by estimating the average additional cost generated by the presence of an NI (additional cost) after matching propensity scores via the average treatment effects on the treated (ATT) parameter. STATA 14 statistical software will be used for this purpose, and the 95% confidence interval for the ATT will be determined, using the bootstrap method by determining the 2.5th percentile and 97.5th percentile of the ATT series obtained after resampling and matching in 1,000 iterations ( 56 , 62 , 63 ). Sensitivity analyses will be conducted in both steps by varying the annual hourly wage of nonphysician professionals from minimum to maximum according to their salary scales.
Component 4: Determination of the Optimal Breakeven Point for NI Prevention Practices
Associated with objective three of the project, this more analytical component is aimed at measuring the return on investment in NIPC by comparing the costs of implementing CBPs against the potential benefits. This will involve estimating the potential benefits associated with the reduction of NIs via CBPs and determining the optimal breakeven point at which implementing CBP activities associated with NICP becomes profitable.
Calculation of potential benefits: If the use of CBPs associated with NIPC leads to an X% reduction in NIs and, consequently, a reduction in NI costs related to management of carrier or infected patients, economic benefits will be observed ( 64 ). Taking into account an X% reduction in NI prevalence due to an NIPC program, the benefit (B) is calculated as follows: B = (1 – X%) × total cost of NIs. Because the results in the literature are divergent, with the proven effectiveness of NIPC programs ranging from 0 to 50% ( 31 ), we will conduct a sensitivity analysis to estimate benefits by varying X%. Determination of the breakeven point: The cost-effectiveness of the NIPC program in relation to CBPs will be assessed by comparing the benefits of the NIPC intervention with the costs of implementing CBPs. The benefit–cost ratio (B/C) will be calculated. This will provide information on the savings achieved, in 2019 dollars, for each dollar invested in the NIPC program related to implementing CBPs. The program will be considered cost-effective if the B/C ratio is >1. This ratio will be calculated by varying the percentage X of NI reduction from 0 to 50%. Sensitivity analyses will also be conducted to determine the breakeven point for the NIPC program in relation to the four CBPs.
Knowledge Transfer and Results Use
Before presenting the knowledge transfer strategies, a brief overview of the different stakeholders involved in this proposal is in order. The Université du Québec en Outaouais is represented by the principal investigator (Prof. Eric Tchouaket), a researcher in health economics and holder of an FRQS junior 1 career award (2017–2021); one co-investigator, a physician and public health specialist (Dr. Drissa Sia); and one co-investigator, a nursing research fellow and former NIPC consultant (Dr. Sylvain Brousseau). The Université de Montréal is represented by one co-investigator, a nurse specialized in time–motion grid development and validation (Dr. Kelley Kilpatrick). The CISSS des Laurentides is represented by one co-investigator, the overall coordinator of the project, a clinical nurse specialized in NIPC (Ms. Sandra Boivin). The CIUSSS du Saguenay–Lac-Saint-Jean is represented by one co-investigator, an NIPC senior consultant (Ms. Catherine Larouche). The Montreal Heart Institute is represented by one co-investigator, a coordinator in hygiene and sanitation (Mr. Bruno Dubreuil). The INSPQ is represented by one co-investigator, a consultant in nursing care, immunization, and nosocomial infections from the Department of Biological Risk and Occupational Health (DRBST) and NIPC expert (Ms. Natasha Parisien). The project has been endorsed by the NICP leaders in both regions of the study. Added to this team are the Réseau de recherche en interventions en sciences infirmières du Québec (RRISIQ) , which partially funded component 1; and the Fonds de Recherche Québec en Santé (FRQS) , which provided a career award to the principal investigator from 2017 to 2021 to conduct components 1, 2, and 3 of this proposal. The MSSS , which is the organization that will implement and conduct the work related to target 22 of its action plan, is theoretically an ex officio stakeholder in this proposal. The team also includes research assistants (one statistician and one computer scientist), research nurses (responsible for data collection), and students (master's or doctoral).
Knowledge transfer is central to this project proposal. The aim is to present clearly how the results will be used and transferred to the various stakeholders and key actors in the Quebec health network (policy-makers, including the MSSS; the INSPQ via the SPIN and the CINQ (Comité des infections nosocomiales du Québec); the CEOs of the CISSS and CIUSSS and their boards of directors; nursing directors; health professionals; and researchers).
First, the partnership built through this proposal will foster ongoing interactions among decision-makers, researchers, managers, and practitioners. It is based on a deliberative approach that incorporates knowledge translation as an integral part of the research process. The knowledge generated will be discussed, adapted, or negotiated within the teams to be applied in specific contexts. Regular meetings will be held with all stakeholders to organize data collection, mobilize the staff of the institutions involved, finalize the methodology, and discuss the results. Collaborations with the public health and nursing departments of the CISSS des Laurentides and the CIUSSS du Saguenay–Lac-Saint-Jean, as well as with the experts participating in the study (component 1, the Delphi process) will provide opportunities to design and implement knowledge transfer activities.
Second, the time–motion observation grid developed here, whose content is validated in component 1, can be used by the MSSS for the systematic collection of information related to CBP costs. To this end, a web and mobile application, the AnéPCI , will be developed by the computer technician that will allow the grid to be viewed on computers, tablets, and telephones since the collection of observation data (component 2) will be done via tablets. The platform will be free of charge, but only the principal investigator will grant access to those wishing to consult it. At the same time, the stakeholders in this project (MSSS, INSPQ, researchers, CISSS des Laurentides, and CIUSSS du Saguenay–Lac-Saint-Jean) will have direct access to the platform (secure access). The platform could be used for technological transfer of results and flexible use of the grid in the case of an eventual Quebec-wide scale up to the other CISSSs and CIUSSSs of the network.
Third, the results of component 2 will quantify the costs of implementing CBPs related to NIPC. Since component 2 will be carried out during the same period as component 3 (April 1, 2018, to March 31, 2020) in the same units of the two hospitals studied, the costs of implementing NIPC (component 2 deliverable) will be compared with the costs of managing patients who are carriers or infected by an NI (deliverable 3). This comparison will provide an opportunity to discuss the cost-effectiveness of the NIPC program through the implementation of CBPs.
Once completed, this project will also provide information on the true cost of CBPs in NIPC, i.e., the costs of both basic practices and additional precautions (component 2). In addition, it will help to determine the cost of a patient colonized or infected with CDAD, MRSA, VRE, or CPGNB. For decision-makers , knowledge of infection control costs will allow them to make decisions based on data that represent what is currently being done in facilities within the Quebec health and social services network. This project will allow the MSSS to estimate the cost of its orientations more accurately and to be able to invest human, material, and financial resources in NIPC in the right places and at the right time, using financial resources made cost-effective by the NIPC program. It will, thus, be able to invest in a timely manner in the nurse/patient ratio in additional precautions; nurse ratios for infection prevention; the training of NIPC nurse consultants or specialists; equipment purchases for patient care or for hygiene and sanitation; information and awareness-raising campaigns, etc. CEOs and boards of directors in CISSSs and CIUSSSs will be able to make decisions on investments to avoid repeated outbreaks (e.g., adding dedicated equipment in rooms; adding dedicated staff to a cohort; maintaining an empty bed in a cohort of patients who are carriers or infected with MRBs for 24–48 h to avoid bed transfers and admit them promptly, etc.). They will know the financial implications of not having NIPC measures in their budgets.
Knowledge of NIPC cost-effectiveness data should encourage nursing directors (DSIs) to maximize NI prevention measures to reduce cases of infection. Professional practitioners will be informed about the financial consequences of not applying CBPs in hand hygiene, hygiene and sanitation, and additional precautions. This will provide them with a better understanding of the situation so they can work more closely with managers to ensure the quality and safety of patient care.
Fourth, throughout the study, results of each component will be made available to targeted audiences through a variety of channels: research reports on each component; scientific and review articles; and oral and poster presentations. Appropriate forms of dissemination will be defined in collaboration with managers and decision-makers. All of the stakeholders of the study will be encouraged to make presentations in their communities and to share their opinions on issues related to applying the results of each component of the study.
Fifth, this project will foster the development of more economic analyses in public health and nursing related to infection prevention and control. It will contribute to the training of master's or doctoral students who will be recruited to further develop components 2 and 3. Two research nurses will also be recruited and trained to administer the observation grid, using the platform constructed.
Caution in Interpreting Results
Economic analysis is an approach that provides information on the financial benefits of investing in an intervention. It complements the epidemiological surveillance already underway. Decisions about implementing an intervention cannot depend solely on the results of this type of analysis. Other sociosanitary, ethical, political, and even environmental dimensions also need to be taken into account.
This proposal covers the period from January 1, 2018, to December 31, 2020.
Consent for Publication
Only sociodemographic data will be collected from the participants. Any image and clinical data will be collected. The observation will be done by eye contact, and the time spent for each activity will be counted, using a stopwatch developed in a web/mobile application.
Ethics Statement
This research protocol has been approved (see supplementary file ) by the Multicentre Research Ethics Committee of Centre intégré de santé et de services sociaux de l'Outaouais (CISSSO) and accepted by Research Ethics Committee of the Université du Québec en Outaouais. All the participants will provide informed written consent. They will sign the consent form before the beginning of the observation.
Author Contributions
ET, SBo, CL, NP, BD, SBr, KK, DS, C-AD, AB, and RB made substantial contributions to study conception and design for this research protocol. All the authors were involved in drafting and making revisions for critical intellectual content in the manuscript. All the authors gave final approval of the version to be published.
Conflict of Interest
The authors declare that the research was conducted in the absence of any commercial or financial relationships that could be construed as a potential conflict of interest.
Acknowledgments
We would like to thank RRISIQ, the MSSS, and FRQS for their financial support. They thank all the staff and managers at the public health department of the Laurentians region and the nursing department of the Saguenay–Lac-Saint-Jean region.
Abbreviations
Funding. This research project has been funded by the Partnership from the Quebec Ministry of Health and Social Services (MSSS) from January 1, 2018, to December 31, 2020. The Canadian Institutes of Health Research (CIHR) Grant no. 409071 from April 1, 2019, to December 31, 2021; Fonds de Recherche du Québec-Santé (FRQS) 35124 Grant no from April 1, 2017, to March 31, 2021. Eric Tchouaket received a Junior 1 researcher award from the FRQS. The funding bodies played no role in study development nor in the writing of the manuscript.
Supplementary Material
The Supplementary Material for this article can be found online at: https://www.frontiersin.org/articles/10.3389/fpubh.2021.531624/full#supplementary-material
STUDY PROTOCOL article
Economic analysis of the prevention and control of nosocomial infections: research protocol.
- 1 Department of Nursing, Université du Québec en Outaouais, Gatineau, QC, Canada
- 2 Ingram School of Nursing, McGill University, Montreal, QC, Canada
- 3 CISSS des Laurentides, St.Jerome, QC, Canada
- 4 Institut de Cardiologie-Montreal Heart Institute, Université de Montréal, Montreal, QC, Canada
- 5 Department of Nursing, Centre Intégré Universitaire de Santé et de Services Sociaux du Saguenay–Lac-Saint-Jean, Saguenay, QC, Canada
- 6 Department of Biomedical Risks and Occupational Health, Institut National de Santé Publique du Québec, Quebec, QC, Canada
- 7 School of Public Health, University of Montréal, Montreal, QC, Canada
- 8 School of Public Administration, University of Victoria, Victoria, BC, Canada
Background: Nosocomial infections (NIs) are among the main preventable healthcare adverse events. Like all countries, Canada and its provinces are affected by NIs. In 2004, Ministry of Health and Social Services (MSSS) of Quebec instituted a mandatory surveillance NI program for the prevention and control (NIPC) in the hospitals of the province. One target of the MSSS 2015–2020 action plan is to assess the implementation, costs, effects, and return on investment of NIPC measures. This project goes in the same way and is one of the first major studies in Canada to evaluate the efficiency of the NIPC measures. Three objectives will be pursued: evaluate the cost of implementing clinical best practices (CBPs) for infection control; evaluate the economic burden attributable to NIs; and examine the cost-effectiveness of the NIPC by comparing the costs of CBPs against those of NIs.
Methods: This project is based on an infection control intervention framework that includes four CBPs: hand hygiene; hygiene and sanitation; screening; and additional precautions. Four medical and surgical units in two hospitals (nonUniversity, University) in the province of Quebec will be studied. The project has four components. Component 1 will construct and content validate an observation grid for measuring the costs of CBPs. Component 2 will estimate CBP costs via 2-week prospective observations of health workers, conducted every 2 months over a 1-year period. Component 3 will evaluate, through a matched case-control study, the economic burden of the four most monitored NIs in Quebec ( C-difficile , MRSA, VRE, and CPGNB). Archival patient data will be collected retrospectively. Component 4 will determine the optimal breakeven point for CBPs associated with NIPC.
Discussion: This project will produce evidence of the economic analysis of NIPC and give health stakeholders an overview of NIPC cost-effectiveness. It will meet the objectives of the Canadian Patient Safety Institute and the MSSS action plan to analyze the efficiency of NIPC preventive measures. To our knowledge, this is the first such exercise in Quebec and Canada. It will provide governments with a decision support tool through a major empirical study that could be replicated nationally to capture the financial benefits of NIPC.
In recent years, healthcare institutions in Organisation for Economic Co-operation and Development (OECD) countries have been under cultural, regulatory, and, especially, economic pressures. They are confronted with the acceleration of health expenditures ( 1 , 2 ), problems related to population aging and to medical and technological advances ( 3 , 4 ), and rising expectations of patients regarding care quality and safety ( 3 ). Over this time, standards for governance, leadership, drug management, and infection prevention, and control have been imposed on health facilities ( 5 ). The quality and safety of inpatient care is a major issue in health systems. As a result, interest in matters of safety has been growing in recent years ( 6 – 11 ). Health professionals, decision-makers, the public, and scientists are all deeply preoccupied with analyzing patient safety and infection control in healthcare facilities ( 12 ).
Nosocomial infections (NIs) are infections acquired during an episode of care in a healthcare facility ( 13 ). They are a significant burden on patients, healthcare organizations, and the public health system ( 14 , 15 ). They lead to increases in care complications, mortality, morbidity, and lengths of hospital stays ( 16 – 19 ). They also generate higher human, material, and financial costs for health facilities ( 16 – 18 ). NIs are one of the main preventable health care adverse events ( 20 ). Like every country in the world, Canada is affected by NIs. In 2013, the Public Health Agency of Canada reported that more than 200,000 patients contract nosocomial infections each year, resulting in more than 8,000 deaths. Since 1997, mortality rates due to Clostridium difficile-associated diarrhea (CDAD) have tripled in Canada. From 1995 to 2009, the rate of NIs caused by methicillin-resistant Staphylococcus aureus (MRSA) bacteremia increased by more than 1,000% ( 21 ). In addition, the incidence of vancomycin-resistant enterococcus (VRE) in Canada increased significantly from approximately.02 cases per 10,000 patient days in 1999 to approximately.68 cases per 10,000 patient days in 2011 ( 22 ).
The province of Quebec is no exception to this reality. To address this persistent problem, the Ministry of Health and Social Services (MSSS) implemented, in response to the 2004 CDAD crisis, standardized mandatory surveillance of NIs in all hospitals in the province. This surveillance is based on a reference program for nosocomial infection prevention and control (NIPC program), which has become the mainstay for healthcare institutions in managing risks, quality, and patient safety. The program is based on seven key actions: (1) surveillance of NIs and monitoring for emergent infectious problems; (2) development of policies, procedures, and support measures; (3) education and training; (4) evaluation; (5) communication and information; (6) outbreak management; and (7) risk management. Its effective implementation should lead to a reduction in NIs ( 23 ).
It is with this in mind that decision-makers in the health network wish to evaluate the implementation, costs, effects, and return on investment of the NIPC program. To this end, in target 22 of its 2015–2020 action plan ( 24 ) on the prevention and control of NIs for safe delivery of healthcare in the province of Quebec, the MSSS sets out the need to: “evaluate NIPC measures, taking into account the organizational model, the burden of disease, as well as their clinical and epidemiological impacts.” Action 1 of that same target stipulates the need to “assess the clinical and economic impacts of NIs, as well as the outcomes of measures to prevent and control these infections in both general and specialized hospitals” ( 25 ). This action by the MSSS is in line with the approach that has been observed since the last economic recession in 2008 in terms of controlling public spending.
The issue of infection prevention investment cost is undeniably very relevant and timely. Moreover, since 2010, Canada has shown great interest in assessing costs associated with adverse events and nosocomial infections ( 26 ). In fact, the Canadian Patient Safety Institute funded a project entitled The Economic Burden of Patient Safety to undertake research to assess the economic impact of adverse events and the potential benefits for patients and the healthcare system of reducing them ( 12 ). Meanwhile, the province of Quebec did not stand idly by. In 2011, the public health directors of the MSSS initiated a study on the economic benefits of public health interventions. This project, led by the Charles LeMoyne Hospital Research Centre under the direction of Professor Astrid Brousselle and Eric Tchouaket, was aimed at developing robust, economically sound arguments to demonstrate the efficiency (economic value) of certain public health programs in Quebec. The programs studied were chosen by public health directors of Quebec at their provincial round table. This is how the project on the economic analysis (EA) of the NIPC program of Quebec came into being. The present research project is part of the same strategy. This is the first major study in Quebec to evaluate the efficiency of an NIPC program. Several benefits are envisaged. On the clinical front, this project will inform healthcare providers and facility managers about the benefits of rigorous application of NIPC program measures, as well as about the costs of managing a patient who is either a carrier of, or infected with, an NI. On the political front, it will provide a strong evidence-based rationale for NIPC investment in Quebec and Canada. It will, thereby, help the MSSS achieve target 22 of its 2015–2020 action plan. On the research front, this project is a precursor to the development of EA studies on both public health and nursing interventions.
The main objective of the project is to evaluate the efficiency of an NIPC program by conducting an economic analysis of clinical best practices (CBPs) implemented. Three specific objectives will be pursued:
1. Evaluate the cost of implementing CBPs associated with NIPC;
2. Assess the economic burden attributable to NIs;
3. Examine the cost-effectiveness of NIPC by comparing the cost of implementing CBPs against the costs of NIs.
Main Hypothesis
This research project is based on the hypothesis that proper implementation of measures related to clinical best practices for prevention and control of NIs is efficient. In other words, investing in these measures is both beneficial in terms of health and economically profitable.
Theoretical Framework
This project builds on the adverse-events prevention framework developed by Resar et al. ( 27 ) at the Institute for Healthcare Improvement in the United States, which advocates implementing “bundles” of three to five evidence-based clinical best practices (CBPs) in professional teams. These practices ensure healthcare providers can, together, provide safe care for their patients. This intervention framework supported the implementation, in Canadian and Quebec healthcare facilities, of infection prevention and control strategies and the deployment of Canadian ( 28 ) and Quebec ( 29 , 30 ) safe care campaigns. According to the Public Health Agency of Canada, the use of best practices for prevention would reduce the risk of contracting some NIs to near zero ( 21 ). The CBPs for NIs are subdivided into four essential measures: (1) hand hygiene; (2) hygiene and sanitation of surfaces and equipment; (3) screening on the admission of patients who are carriers or infected, in accordance with the protocols of the healthcare facility; and (4) application of additional precautions. Appendix 1 presents a diagram of the project framework based on the CBPs associated with NIPC.
i) Hand hygiene . Hand hygiene refers to the washing and disinfecting (hygienic and surgical) of hands, wrists, and forearms using water, soap, and hydroalcoholic solutions (HAS) at four specific times (see Appendix 2 ). This action extends from the initial wetting of the hands to the point where they are completely dry ( 31 ). The World Health Organization (WHO) estimates that hand hygiene could help reduce healthcare-associated infections by 50%.
ii) Hygiene and sanitation of surfaces and equipment . In Québec, a 2005 report from the MSSS committee studying NIPC, entitled D'abord, ne pas nuire [ First, Do No Harm ], stressed the importance of cleanliness and sanitation as one of the fundamental measures for infection prevention and control ( 32 ). Neglect of regular preventive cleaning and disinfection of surfaces and equipment creates a reservoir for the spread of microorganisms. Hygiene and sanitation activities must be carried out with appropriate frequency (daily, or several times daily), depending on the risk area ( 33 , 34 ).
iii) Screening on admission of patients who are carriers or infected . Screening is the systematic search, in persons with or at risk for NI, for any hitherto undetected condition or anomaly, based on screening algorithms ( Appendix 3 ). Screening techniques differ according to the type of NI. In general, screening involves performing clinical diagnosis and laboratory analysis. Any patient currently or previously hospitalized is considered at risk if he or she presents with signs and symptoms of infection. Analyses of stools or clinical specimens (for CDADs), blood, nasal smears, blood cultures, and laboratory tests can be used to detect NIs, in accordance with predefined surveillance protocols for each NI, in symptomatic or even asymptomatic patients ( 35 – 39 ).
iv) Additional precautions . Besides the three above-mentioned basic practices, additional precautions must be taken when an NI is declared. While they depend on the infection detected, they involve, among other things, implementing isolation measures and contact precautions for patients who are carriers or infected ( 40 ). In the event that a major outbreak of an NI is declared, CBPs must be applied intensively and additional care team meetings and resources added for as long as it continues ( 41 ).
An Operational Framework and Research Questions
Prior to embarking on an EA, it is important to understand that any interpretation of economic studies must consider three elements: the analytical perspective, the time horizon, and the allocation of healthcare resources and interventions, taking into account the prior condition of the patient ( 12 ). The analytical perspective determines what costs are included in the calculations. The study can be done from the patient, hospital, or societal perspective. For example, from a hospital perspective, medical costs will not include costs related to the patient post-discharge nor those related to lost productivity due to hospitalization. The time horizon defines the time frame for measuring medical costs. As well, the stage and severity of illness , comorbidities, risk factors, admitting diagnosis, and length of stay all influence the costs of care ( 12 , 42 , 43 ). Conducting an EA of an NIPC program thus involves examining questions about the quality of management and prevention, as well as about the safety of care. Thus, as Finkler (1993, 1996) states, the cost of managing quality takes into account the cost of investing in preventive measures and the costs associated with poor quality or with problems experienced ( 44 , 45 ). According to Finkler, a certain level of quality could be achieved by investing in prevention. Thus, there is a breakeven point, referred to as “the optimum,” above which prevention could increase with quality. Thus, based on Finkler's model (1993, 1996), an EA of the NIPC program, using CBPs, involves answering the following questions:
(i) What is the cost of investing in prevention through best clinical practices in NIPC?
(ii) What are the costs of NIs?
(iii) What is the optimal breakeven point for measuring return on investment when comparing prevention intervention costs against potential benefits?
Settings and Project Scope
Because NI incidence varies according to types of facilities and of patients present in hospitals ( 23 ), and in keeping with the standards for reporting statistics by region in the documents of Provincial NI Surveillance Group (SPIN) of Quebec ( 35 – 38 ), this project will be conducted in two Quebec hospitals, one nonUniversity and the other University affiliated. As such, one of the selected sites will be a nonUniversity hospital included in the CISSS des Laurentides (integrated health and social services centre for the Laurentians region), and the other, a University-affiliated hospital included in the CIUSSS (integrated University centre for health and social services) of the Saguenay–Lac-Saint-Jean region.
Four reasons prompted the choice of these two regions. First, these regions are de facto part of this project, given the involvement of researchers and professionals with NIPC expertise affiliated with universities and health centers in these regions. Second, in the Laurentians region, the incidence rate of Clostridium difficile-associated diarrhea (CDAD) has been comparable to the provincial average over the past 5 years ( 35 ). The Saguenay–Lac-Saint-Jean region has an incidence rate of CDAD slightly below the provincial average for the same period. Third, the Laurentians region has an incidence rate of nosocomial VRE colonization higher than the provincial average over the past 5 years (with the exception of 2016–2017), while, over the same period, this rate has been significantly lower than the provincial average in the Saguenay–Lac-Saint-Jean region ( 36 ). Lastly, the Laurentians region is a CISSS while the Saguenay–Lac-Saint-Jean region is a CIUSSS. These are two different case types that will help cover a fairly broad spectrum of costs in Quebec hospitals. A nonUniversity hospital will be selected from the CISSS, and a University-affiliated hospital will be selected from the CIUSSS. It is important to note that two co-investigators (Sandra Boivin and Catherine Larouche) will facilitate obtaining the consent and participation of the two hospitals, each in their respective regions. Moreover, a similar project has been submitted to the multicenter ethics committee of the Laurentians CISSS with the involvement of the Saguenay–Lac-Saint-Jean CIUSSS.
Project Components
The proposed project has four components that each provides deliverables:
Component 1: Construction and Content Validation of an Observation Grid for Measuring the Costs of CBPs Associated With NIPC
The best clinical practices that will be studied are those described in guidelines for the prevention of CDAD, MRSA, VRE, and carbapenemase-producing Enterobacteriaceae (CPE, or CPGNB).
Methods : A “time-motion” observation grid ( 46 , 47 ) was constructed to measure the time taken (in seconds) by health workers to perform each CBP (hand hygiene, hygiene and sanitation, screening, and additional precautions). This grid was developed in accordance with the strategies and methods for measuring compliance in each of the CBPs recommended by the Public Health Institute (INSPQ) of Quebec ( 29 , 30 ). The grid was constructed according to the algorithm developed by the project team, which is presented in Appendix 2 . Using a Delphi approach, the grid will be validated by 18 experts (six field experts from CISSS des Laurentides, six field experts from CIUSSS du Saguenay–Lac-St-Jean, and four content experts). The field experts are NIPC professionals, microbiology and infectious disease specialists, as well as professionals in hygiene and sanitation. The content experts have been selected for their expertise in the field of NIPC. The degree of content validity of each section of the grid will be measured by means of the content validity index (CVI) by retrieving the percentage of statements rated 3 and 4 (number of statements rated 3 and 4/total number of statements in the grid) ( 40 – 50 ). A CVI ≥0.80 will show that the grid is acceptable and its content valid with minor corrections ( 49 ). If it is <0.80 in any section of the grid, that section will undergo major revision and then be resubmitted to the same experts for assessment. Referring to their previous comments and suggestions, they will be asked to reassess it, based on the previous ordinal scale, until a CVI ≥0.80 is obtained for each section of the observation grid. At least two rounds will be required.
Deliverable 1 : Production and validation of a standardized grid , which can be used in all general and specialized (medical and surgical) physical health services units in Quebec to measure the costs of the four practices: hand hygiene, hygiene and sanitation, NI screening, and additional precautions.
Component 2: Use of the Constructed and Validated Grid to Assess the Costs of the Four CBPs in a CISSS Hospital in the Laurentians Region and a University-Affiliated CIUSSS Hospital in the Saguenay–Lac-Saint-Jean Region
In each of the two hospitals , two units from the general and specialized (medical and surgical) physical health services will be randomly selected for the study. A prospective investigation ( 48 ) will be conducted in the four study units to collect information on CBPs through direct observation.
Methods: In line with CBP compliance assessment methods ( 29 , 30 ), a sample of practitioners, a sample of patient records, and a sample of patients will be selected for each type of CBP studied over the course of the year (April 1, 2019, to March 31, 2020).
For hand hygiene , in each of the four units of the two selected hospitals, three physicians, three nurses, three nursing assistants, and three patient attendants will be randomly selected from the day and evening shifts. During this period, for each hand hygiene opportunity, time taken (in seconds) and products used will be systematically recorded. For hygiene and sanitation , in addition to the above-mentioned practitioners, three hygiene and sanitation workers will also be randomly selected and observed during disinfection (daily and terminal) of the ordinary rooms and/or for additional precautions.
Each of the 15 workers will be shadowed by a research nurse observer duly trained for this purpose, for 3 h in their shift. Given the heavy volume and activity during the day, two out of three workers will be randomly selected during the day shift (10 during the day and five in the evening).
As recommended by INSPQ ( 29 , 30 ) for the assessment of CBP compliance, observations will be made during six 2-week observation periods conducted every 2 months over the course of 1 year.
A total of 15 × 6 = 90 workers (3 × 6 = 18 physicians, 18 nurses, 18 nursing assistants, 18 patient attendants, and 18 hygiene and sanitation workers) will be observed per unit during 1 year. Thus, for two units in one hospital, 180 workers will be observed during the year, i.e., 360 workers for the two hospitals. It is possible, due to random selection, that a worker may be observed more than one time during the year.
Practices related to additional precautions will be systematically observed when applied by the workers.
With regard to screening , as suggested by the INSPQ ( 29 , 30 ), in each 2-week observation period, to be conducted six times over the year, 15 clinical records of patients screened (at admission or during hospitalization) for MRSA, VRE, and CPGNB (i.e., five records for each of the three screened NIs) will be randomly selected from the total number of patient records screened in the two units of each hospital. In addition, five records with diagnostic tests for CDAD detection will also be randomly selected from the records of patients with diarrhea in the two units of each hospital. Thus, 20 files × 6 observation periods, or 120 patient files studied per hospital, will be reviewed during the year, i.e., 240 patient files for both hospitals in total. All screening tests and diagnostic tests (related to CDAD) performed on a selected patient will be noted. The screening and diagnostic testing algorithms for each NI ( 51 – 54 ) in Appendix 3 will be used as models. By associating a cost with each test, the total cost of patient screening will be calculated. An average annual cost will be estimated.
With regard to training and information sessions and any meetings that may be organized by the NIPC service of the hospital in the units studied, a dashboard will be maintained throughout the observation year to systematically record participants, their professional profiles, trainers, instructors, duration of meetings, and equipment used. Finally, one representative (e.g., from the central supplies service) from each hospital (two in total) will provide information on unit prices and depreciation for each of the products and materials used. Thus, in addition to the activities of NIPC nurses (the grid), awareness-raising and information campaigns, and training activities for health personnel in the unit, information will be collected at weekly, monthly, and annual meetings; management and coordination activities related to NIPC in the care units; evaluation activities; and investments made (as needed).
Deliverable 2 : An estimate of the average annual cost of each CBP (hand hygiene, hygiene and sanitation, screening, additional precautions) and the average annual overall cost of actions implemented for NICP (including training and information activities), whether during an outbreak period or not, in a teaching hospital and a non-teaching hospital. To this end, costs will be estimated on a 1-year horizon in Canadian dollars (CAD) and from a healthcare facility perspective (costs related only to hospital activities). The following cost indicators will be estimated (in days) per unit: the cost of an observation; the cost of a CBP; the cost per worker; and the overall cost of the four CBPs considered together in one unit. The cost of an observation will be calculated as follows: (Time spent [in minutes] on an observation × the hourly wage of the worker [in minutes) × 24 h + (Depreciated cost of the product or material used (in days) during the observation). The cost of a CBP (in days) will be the sum of the costs of the observations made. The overall cost will be the sum of CBP costs and costs related to meetings, training sessions, and awareness-raising campaigns. Sensitivity analyses will be carried out by varying the hourly wages of workers from minimum to maximum, according to their salary scales (lowest and highest salary scales). For physicians, the rate for a regular consultation for a patient at risk of infection will be applied.
Component 3: Assessment of the Economic Burden Attributable to the Occurrence of Nosocomial Infections in Two Quebec Hospitals
While the costs of NIs in healthcare facilities have been sufficiently analyzed in Western scientific literature ( 12 , 55 ), to our knowledge, there are currently no empirical studies in Quebec on the financial costs associated with the four NIs most closely monitored in the province (CDAD, MRSA, VRE, and CPGNB). Complementing the epidemiological surveillance carried out by SPIN, the results of Component 3 will provide decision-makers in the Quebec healthcare network with information on the health-related and economic benefits of strengthening the NIPC program.
Methods: Assessing the economic burden of NIs involves estimating, from the healthcare facilities perspective, the additional financial cost of each of the four Nis during a care episode. As such, it includes the additional financial costs attributable to the increase in overall care consumption, the extension of the length of stay, the consumption of drugs, and the increase in laboratory tests caused by the occurrence of an NI ( 56 ). This component is based on a matched case-control design of patients with an NI (colonized or infected patients: cases) with similar patients who have not contracted an NI (controls) for a given period of time. The advantage of using such a design is that it is suitable for infrequent diseases such as NIs, and it also allows comparisons to be made between similar groups that are differentiated only by the presence of an illness ( 57 ).
Population : Included: Patients 18 years of age and over hospitalized for at least 72 h in the four units between April 1, 2019, and March 31, 2020. A patient readmitted either for a new hospital stay or for a complication related to the initial admission will be considered a new patient. Patients will be followed for a maximum of 30 days on the unit. A case is a patient who has contracted one of the four NIs studied. A control is a similar patient who did not develop an NI during the same care episode. Matching : Propensity score matching will be used, followed by 1:1 nearest neighbor matching ( 58 – 60 ). As in D'Amour et al. ( 9 ) and Tchouaket et al. ( 56 ) the cases and controls will be matched according to age, sex, risk factors, admitting diagnoses, comorbidities, date of admission to the unit, and severity of illness on admission as measured by the Charlson index ( 61 ). Sample size : All cases of each of the NIs occurring between April 1, 2019, and March 31, 2020, in the care units of the two hospitals will be included in the study. For MRBs (MRSA, VRE, and CPGNB), a distinction will be made between colonized patients (diagnosed on admission) and patients infected in the hospital. Data source : Data from hospital archives containing information on the units studied will be used. A grid (see a model in Appendix 4 ) will be used to extract the following information: health status of a patient on admission; date of NI occurrence; date of discharge of the patient from the unit; care and services consumed during the period in the unit; medical procedures performed by physicians; interventions carried out on the patient (professional involved, date, time spent); drugs consumed; laboratory tests performed; and imaging services used.
Deliverable 3 : An estimate of the care and services consumption cost for each patient group (cases and controls) over a 1-year horizon and from a health facility perspective , and cost comparison between cases and controls to determine the financial burden (additional cost) generated by the occurrence of an NI . First, the time spent by nonphysician professionals to perform each intervention on a patient will be multiplied by the hourly wage of the professional in question to estimate the cost per nonphysician professional intervention per patient . Data on medical procedures performed for the benefit of a patient (according to the profile of the physician) will be collected, and, based on RAMQ and AP-DRG data, the medical (physician) costs per patient associated with each procedure will be estimated. Laboratory and medical imaging fees will be determined from the MSSS price list to estimate the cost of diagnostic tests per patient. Lastly, the prices of each drug consumed (based on actual drug costs at the pharmacy, without insurance deduction) will be used to estimate the cost of drugs consumed per patient . The cost of care and services consumed per patient will be the sum of the cost of nonphysician professional interventions received, the cost of medical procedures, the cost of diagnostic tests, and the cost of drugs consumed per patient . Second, the additional cost generated by the occurrence of an NI will be determined by estimating the average additional cost generated by the presence of an NI (additional cost) after matching propensity scores via the average treatment effects on the treated (ATT) parameter. STATA 14 statistical software will be used for this purpose, and the 95% confidence interval for the ATT will be determined, using the bootstrap method by determining the 2.5th percentile and 97.5th percentile of the ATT series obtained after resampling and matching in 1,000 iterations ( 56 , 62 , 63 ). Sensitivity analyses will be conducted in both steps by varying the annual hourly wage of nonphysician professionals from minimum to maximum according to their salary scales.
Component 4: Determination of the Optimal Breakeven Point for NI Prevention Practices
Associated with objective three of the project, this more analytical component is aimed at measuring the return on investment in NIPC by comparing the costs of implementing CBPs against the potential benefits. This will involve estimating the potential benefits associated with the reduction of NIs via CBPs and determining the optimal breakeven point at which implementing CBP activities associated with NICP becomes profitable.
Calculation of potential benefits: If the use of CBPs associated with NIPC leads to an X% reduction in NIs and, consequently, a reduction in NI costs related to management of carrier or infected patients, economic benefits will be observed ( 64 ). Taking into account an X% reduction in NI prevalence due to an NIPC program, the benefit (B) is calculated as follows: B = (1 – X%) × total cost of NIs. Because the results in the literature are divergent, with the proven effectiveness of NIPC programs ranging from 0 to 50% ( 31 ), we will conduct a sensitivity analysis to estimate benefits by varying X%. Determination of the breakeven point: The cost-effectiveness of the NIPC program in relation to CBPs will be assessed by comparing the benefits of the NIPC intervention with the costs of implementing CBPs. The benefit–cost ratio (B/C) will be calculated. This will provide information on the savings achieved, in 2019 dollars, for each dollar invested in the NIPC program related to implementing CBPs. The program will be considered cost-effective if the B/C ratio is >1. This ratio will be calculated by varying the percentage X of NI reduction from 0 to 50%. Sensitivity analyses will also be conducted to determine the breakeven point for the NIPC program in relation to the four CBPs.
Knowledge Transfer and Results Use
Before presenting the knowledge transfer strategies, a brief overview of the different stakeholders involved in this proposal is in order. The Université du Québec en Outaouais is represented by the principal investigator (Prof. Eric Tchouaket), a researcher in health economics and holder of an FRQS junior 1 career award (2017–2021); one co-investigator, a physician and public health specialist (Dr. Drissa Sia); and one co-investigator, a nursing research fellow and former NIPC consultant (Dr. Sylvain Brousseau). The Université de Montréal is represented by one co-investigator, a nurse specialized in time–motion grid development and validation (Dr. Kelley Kilpatrick). The CISSS des Laurentides is represented by one co-investigator, the overall coordinator of the project, a clinical nurse specialized in NIPC (Ms. Sandra Boivin). The CIUSSS du Saguenay–Lac-Saint-Jean is represented by one co-investigator, an NIPC senior consultant (Ms. Catherine Larouche). The Montreal Heart Institute is represented by one co-investigator, a coordinator in hygiene and sanitation (Mr. Bruno Dubreuil). The INSPQ is represented by one co-investigator, a consultant in nursing care, immunization, and nosocomial infections from the Department of Biological Risk and Occupational Health (DRBST) and NIPC expert (Ms. Natasha Parisien). The project has been endorsed by the NICP leaders in both regions of the study. Added to this team are the Réseau de recherche en interventions en sciences infirmières du Québec (RRISIQ) , which partially funded component 1; and the Fonds de Recherche Québec en Santé (FRQS) , which provided a career award to the principal investigator from 2017 to 2021 to conduct components 1, 2, and 3 of this proposal. The MSSS , which is the organization that will implement and conduct the work related to target 22 of its action plan, is theoretically an ex officio stakeholder in this proposal. The team also includes research assistants (one statistician and one computer scientist), research nurses (responsible for data collection), and students (master's or doctoral).
Knowledge transfer is central to this project proposal. The aim is to present clearly how the results will be used and transferred to the various stakeholders and key actors in the Quebec health network (policy-makers, including the MSSS; the INSPQ via the SPIN and the CINQ (Comité des infections nosocomiales du Québec); the CEOs of the CISSS and CIUSSS and their boards of directors; nursing directors; health professionals; and researchers).
First, the partnership built through this proposal will foster ongoing interactions among decision-makers, researchers, managers, and practitioners. It is based on a deliberative approach that incorporates knowledge translation as an integral part of the research process. The knowledge generated will be discussed, adapted, or negotiated within the teams to be applied in specific contexts. Regular meetings will be held with all stakeholders to organize data collection, mobilize the staff of the institutions involved, finalize the methodology, and discuss the results. Collaborations with the public health and nursing departments of the CISSS des Laurentides and the CIUSSS du Saguenay–Lac-Saint-Jean, as well as with the experts participating in the study (component 1, the Delphi process) will provide opportunities to design and implement knowledge transfer activities.
Second, the time–motion observation grid developed here, whose content is validated in component 1, can be used by the MSSS for the systematic collection of information related to CBP costs. To this end, a web and mobile application, the AnéPCI , will be developed by the computer technician that will allow the grid to be viewed on computers, tablets, and telephones since the collection of observation data (component 2) will be done via tablets. The platform will be free of charge, but only the principal investigator will grant access to those wishing to consult it. At the same time, the stakeholders in this project (MSSS, INSPQ, researchers, CISSS des Laurentides, and CIUSSS du Saguenay–Lac-Saint-Jean) will have direct access to the platform (secure access). The platform could be used for technological transfer of results and flexible use of the grid in the case of an eventual Quebec-wide scale up to the other CISSSs and CIUSSSs of the network.
Third, the results of component 2 will quantify the costs of implementing CBPs related to NIPC. Since component 2 will be carried out during the same period as component 3 (April 1, 2018, to March 31, 2020) in the same units of the two hospitals studied, the costs of implementing NIPC (component 2 deliverable) will be compared with the costs of managing patients who are carriers or infected by an NI (deliverable 3). This comparison will provide an opportunity to discuss the cost-effectiveness of the NIPC program through the implementation of CBPs.
Once completed, this project will also provide information on the true cost of CBPs in NIPC, i.e., the costs of both basic practices and additional precautions (component 2). In addition, it will help to determine the cost of a patient colonized or infected with CDAD, MRSA, VRE, or CPGNB. For decision-makers , knowledge of infection control costs will allow them to make decisions based on data that represent what is currently being done in facilities within the Quebec health and social services network. This project will allow the MSSS to estimate the cost of its orientations more accurately and to be able to invest human, material, and financial resources in NIPC in the right places and at the right time, using financial resources made cost-effective by the NIPC program. It will, thus, be able to invest in a timely manner in the nurse/patient ratio in additional precautions; nurse ratios for infection prevention; the training of NIPC nurse consultants or specialists; equipment purchases for patient care or for hygiene and sanitation; information and awareness-raising campaigns, etc. CEOs and boards of directors in CISSSs and CIUSSSs will be able to make decisions on investments to avoid repeated outbreaks (e.g., adding dedicated equipment in rooms; adding dedicated staff to a cohort; maintaining an empty bed in a cohort of patients who are carriers or infected with MRBs for 24–48 h to avoid bed transfers and admit them promptly, etc.). They will know the financial implications of not having NIPC measures in their budgets.
Knowledge of NIPC cost-effectiveness data should encourage nursing directors (DSIs) to maximize NI prevention measures to reduce cases of infection. Professional practitioners will be informed about the financial consequences of not applying CBPs in hand hygiene, hygiene and sanitation, and additional precautions. This will provide them with a better understanding of the situation so they can work more closely with managers to ensure the quality and safety of patient care.
Fourth, throughout the study, results of each component will be made available to targeted audiences through a variety of channels: research reports on each component; scientific and review articles; and oral and poster presentations. Appropriate forms of dissemination will be defined in collaboration with managers and decision-makers. All of the stakeholders of the study will be encouraged to make presentations in their communities and to share their opinions on issues related to applying the results of each component of the study.
Fifth, this project will foster the development of more economic analyses in public health and nursing related to infection prevention and control. It will contribute to the training of master's or doctoral students who will be recruited to further develop components 2 and 3. Two research nurses will also be recruited and trained to administer the observation grid, using the platform constructed.
Caution in Interpreting Results
Economic analysis is an approach that provides information on the financial benefits of investing in an intervention. It complements the epidemiological surveillance already underway. Decisions about implementing an intervention cannot depend solely on the results of this type of analysis. Other sociosanitary, ethical, political, and even environmental dimensions also need to be taken into account.
This proposal covers the period from January 1, 2018, to December 31, 2020.
Consent for Publication
Only sociodemographic data will be collected from the participants. Any image and clinical data will be collected. The observation will be done by eye contact, and the time spent for each activity will be counted, using a stopwatch developed in a web/mobile application.
Ethics Statement
This research protocol has been approved (see supplementary file ) by the Multicentre Research Ethics Committee of Centre intégré de santé et de services sociaux de l'Outaouais (CISSSO) and accepted by Research Ethics Committee of the Université du Québec en Outaouais. All the participants will provide informed written consent. They will sign the consent form before the beginning of the observation.
Author Contributions
ET, SBo, CL, NP, BD, SBr, KK, DS, C-AD, AB, and RB made substantial contributions to study conception and design for this research protocol. All the authors were involved in drafting and making revisions for critical intellectual content in the manuscript. All the authors gave final approval of the version to be published.
This research project has been funded by the Partnership from the Quebec Ministry of Health and Social Services (MSSS) from January 1, 2018, to December 31, 2020. The Canadian Institutes of Health Research (CIHR) Grant no. 409071 from April 1, 2019, to December 31, 2021; Fonds de Recherche du Québec-Santé (FRQS) 35124 Grant no from April 1, 2017, to March 31, 2021. Eric Tchouaket received a Junior 1 researcher award from the FRQS. The funding bodies played no role in study development nor in the writing of the manuscript.
Conflict of Interest
The authors declare that the research was conducted in the absence of any commercial or financial relationships that could be construed as a potential conflict of interest.
Acknowledgments
We would like to thank RRISIQ, the MSSS, and FRQS for their financial support. They thank all the staff and managers at the public health department of the Laurentians region and the nursing department of the Saguenay–Lac-Saint-Jean region.
Supplementary Material
The Supplementary Material for this article can be found online at: https://www.frontiersin.org/articles/10.3389/fpubh.2021.531624/full#supplementary-material
Abbreviations
AB, Astrid Brousselle; ATT, Average treatment effects on the treated; BD, Bruno Dubreuil; CAD, Carl-Ardy Dubois; CBP, Clinical best practice; CDAD, Clostridium difficile-associated diarrhea; CISSS, Integrated health and social services center for the Laurentians region; CIUSSS, Integrated University center for health and social services; CL, Catherine Larouche; CPE, or CPGNB, Carbapenemase-producing Enterobacteriaceae; CVI, Content validity index; DS, Drissa Sia; ET, Eric Tchouaket; HAS, Hydroalcoholic solutions; INSPQ, Quebec's Public Health Institute; KK, Kelley Kilpatrick; MRSA, Methicillin-resistant staphylococcus aureus; MSSS, Quebec's Ministry of Health and Social Services; NI, Nosocomial infection; NIPC, NI program for the prevention and control; NP, Natasha Parisien; OECD, Organisation for Economic Co-operation and Development; ROX, Roxanne Borgès Da Silva; SB, Sandra Boivin; SBR, Sylvain Brousseau; SPIN, Quebec's Provincial NI Surveillance Group; VRE, Vancomycin-resistant enterococcus; WHO, World Health Organization.
1. Clavet NJ, Duclos JY, Fortin B, Marchand S, Michaud PC. Les Dépenses en santé du Gouvernement du Québec, 2013–2030: Projections et déterminants . Montreal, QC: CIRANO–Centre interuniversitaire de recherche en analyse des organisations;. (2013) Available online at: https://www.cirano.qc.ca/fr/sommaires/2013s-45 (Accessed Feb 9, 2019).
Google Scholar
2. Contandriopoulos AP, Champagne F, Denis JL, Pineault R. L'évaluation dans le domaine de la santé: concepts et méthodes. Rev Epidemiol Sante Publique. (2000) 48:517–39.
3. Anaes. Les coûts de la qualité et de la non-qualité des soins dans les établissements de santé: état des lieux et propositions . Saint-Denis La Plaine, FR: Anaes–Agence nationale d'accréditation et d'évaluation en santé (2004). Available online at : https://www.has-sante.fr/portail/plugins/ModuleXitiKLEE/types/FileDocument/doXiti.jsp?id=c_268021 . (Accessed Feb 9, 2019).
4. Contandriopoulos AP, Denis JL, Dubois CA. La place et le Rôle du Secteur Privé Dans la Transformation du Système de Soins . Sainte-Foy, QC: Conseil de la santé et du bien-être (2000). Available online at : https://www.csbe.gouv.qc.ca/fileadmin/www/Archives/ConseilSanteBienEtre/Rapports/20001103_rapp_cfr.pdf (Accessed Feb 9, 2019).
5. Accreditation Canada. Health and Social Services Standards . (2019). Available online at: https://accreditation.ca/standards/ (Accessed Feb 9, 2019).
6. Agency for Healthcare Research and Quality. Advancing Patient Safety: Adecade of Evidence, Design and Implementation . AHRQ PublicationNo.09(10)-0084. Rockville, MD: AHRQ; (2009). Available online at: https://www.ahrq.gov/sites/default/files/publications/files/advancing/patient/safety.pdf (Accessed Feb 9, 2019).
7. Blais R, Tamblyn R, Bartlett G, Tré G, St-Germain D. Incidence d'événements Indésirables Dans Les Hôpitaux Québécois . Montreal, QC: Université de Montréal, Groupe de recherche interdisciplinaire en santé (GRIS) (2004) Available online at : http://www.irspum.umontreal.ca/rapportpdf/R04-06.pdf (Accessed Feb 9, 2019).
8. Champagne F, Contandriopoulos AP, Pomey MP, Tanon AA. Élaboration d'un Modèle Conceptuel d'analyse de la Sécurité Dans Les Organisations de Santé . Montreal, QC: Université de Montréal, Groupe de recherche interdisciplinaire en santé (GRIS) (2006). Available online at : http://www.irspum.umontreal.ca/rapportpdf/N06-02.pdf (Accessed Feb 9, 2019).
9. D'Amour D, Dubois CA, Tchouaket É, Clarke S, Blais R. The occurrence of adverse events potentially attributable to nursing care in medical units: cross sectional record review. Int J Nurs Stud. (2014) 51:882–91. doi: 10.1016/j.ijnurstu.2013.10.017
PubMed Abstract | CrossRef Full Text | Google Scholar
10. Institute of Medicine. Crossing the Quality Chasm: A New Health System for the 21st Century . Washington, DC: National Academies Press (2001).
11. National Quality Forum. Safe Practices for Better Healthcare−2009 Update: Aconsensus Report . Washington, DC: NQF (2009).
12. Etchells E, Mittmann N, Koo M, Baker M, Krahn M, Shojania K, et al. The Economics of Patient Safety in Acute Care: Technical Report . Ottawa, ON: Canadian Patient Safety Institute (2012).
13. Baker GR, Norton PG, Flintoft V, Blais R, Brown A, Cox J, et al. The Canadian Adverse Events Study: the incidence of adverse events among hospital patients in Canada. CMAJ. (2004) 170:1678–86. doi: 10.1503/cmaj.1040498
14. Kohn LT, Corrigan JM, Donaldson MS editors. Institute of Medicine Committee on Quality of Health Care in America. To Err is Human: Building a Safer Health System . Washington, DC: National Academies Press (2000).
15. Ducel G, Fabry J, Nicolle L editors. Prevention of Hospital-Acquired Infections: A Practical Guide. 2nd ed. Geneva: World Health Organization, Department of Communicable Disease, Surveillance and Response (2002).
PubMed Abstract | Google Scholar
16. Public Health Agency of Canada. Essential Resources for Effective Infection Prevention and Control Programs: A Matter of Patient Safety–A Discussion Paper . Ottawa, ON: PHAC (2008). Available online at: http://www.phac-aspc.gc.ca/nois-sinp/guide/ps-sp/pdf/ps-sp-eng.pdf (Accessed Feb 9, 2019).
17. Centers for Disease Control Prevention. Hospital Infections Cost U.S. Billions of Dollars Annually. Press release . Atlanta, GA: CDC (2000) Available online at: https://www.cdc.gov/media/pressrel/r2k0306b.htm (Accessed Feb 9, 2019).
18. Jarvis WR. Selected aspects of the socioeconomic impact of nosocomial infections: mordibity, mortality, cost and prevention. Infect Control Hosp Epidemiol. (1996) 17:552–7. doi: 10.1017/S019594170000480X
19. McGarry SA, Engemann JJ, Schmader K, Sexton DJ, Kaye KS. Surgical-site infection due to Staphylococcus aureus among elderly patients: mortality, duration of hospitalization, and cost. Infect Control Hosp Epidemiol. (2004) 25:461–7. doi: 10.1086/502422
20. Leape LL, Brennan TA, Laird N, Lawthers AG, Localio AR, Barnes BA, et al. The nature of adverse events in hospitalized patients. Results of the Harvard Medical Practice Study II. N Engl J Med. (1991) 324:377–84. doi: 10.1056/NEJM199102073240605
21. Public Health Agency of Canada. The Chief Public Health Officer's Report on the State of Public Health in Canada 2013: Infectious Disease–The Never-Ending Threat . Ottawa, ON: PHAC (2013). Available online at: http://publichealth.gc.ca/CPHOReport (Accessed Feb 9, 2019).
22. Public Health Agency of Canada. Vancomycin-Resistant Enterococci Infections in Canadian Acute-Care Hospitals: Surveillance Report January 1, 1999 to December 31, (2011) . Ottawa, ON: PHAC, Infectious Disease Prevention and Control (2013). Available online at: http://publications.gc.ca/collections/collection_2013/aspc-phac/HP40-85-2013-eng.pdf (Accessed Feb, 9 2019).
23. Ministère de la Santé et des Services sociaux. La Prévention et le Contrôle des Infections Nosocomiales: Cadre de Référence à l'intention des Établissements de Santé du Québec . Québec, QC: MSSS, Direction générale de la santé publique (2017). Available online at; http://publications.msss.gouv.qc.ca/msss/fichiers/2017/17-209-01W.pdf .
24. Ministère de la Santé et des Services sociaux. Plan Stratégique du Ministère de la Santé et des Services Sociaux du Québec 2015–2020 (mise à jour 2017) . Québec, QC: MSSS, Direction des communications (2017). Available online at: https://cdn-contenu.quebec.ca/cdn-contenu/adm/min/sante-services-sociaux/publications-adm/plan-strategique/PL_17-717-01W_MSSS.pdf (Accessed Feb, 9 2019)
25. Ministère de la Santé et des Services sociaux. Pour Une Prestation Sécuritaire des soins de santé au Québec: Plan d'action Ministériel 2015–2010 sur la prévention et le contrôle des Infections Nosocomiales . Québec, QC: Gouvernement du Québec (2015). http://publications.msss.gouv.qc.ca/msss/fichiers/2015/15-209-01W.pdf (Accessed Feb, 9 2019)
26. Husereau D, Jacobs P, Manns B, Hoomans T, Marshall D, Tamblyn R, on behalf of the IHE-IHSPR Complex Interventions Working Group. Economic Evaluation of Complex Health System Interventions: A Discussion Paper . Edmonton, AB: Institute of Health Economics (2014). Available online at: http://canimpact.utoronto.ca/wp-content/uploads/2015/07/Economic-Evaluation-of-Complex-Health-System-Interventions.pdf (Accessed Feb, 9 2019).
27. Resar R, Griffin FA, Haraden C, Nolan TW. Using Care Bundles to Improve Health Care Quality. IHI Innovation Series White Paper . Cambridge, MA: Institute for Healthcare Improvement (2012) Available online at: https://emergencylaparotomy.org.uk/wp-content/uploads/2015/11/IHIUsingCareBundlesWhitePaper2012-1.pdf (Accessed Feb, 9 2019).
28. Canadian Patient Safety Institute. New approach to controlling superbugs. Safer Healthcare Now! Campaign . Ottawa, ON: CPSI (2010). Available online at: https://www.patientsafetyinstitute.ca/en/toolsResources/Documents/Interventions/Infection%20Prevention%20and%20Control/NACS%20one%20pager.pdf
29. Laberge A, Carignan A, Galarneau LA, Gourdeau M. L'hygiène et Autres Mesures de Prévention des Infections Associées aux Bactéries Multirésistantes: Document Synthèse . Montreal, QC: Institut national de santé publique du Québec (2014) Available online at: https://www.inspq.qc.ca/pdf/publications/1826_Mesures_Prevention_Bacteries.pdf (Accessed 9 Feb, 2019).
30. Institut national de santé publique du Québec. Campagne Québécoise des soins Sécuritaires–Volet Prévention et Contrôle des Infections . Montreal, QC: INSPQ; (2014). Available online at: https://www.inspq.qc.ca/sites/default/files/documents/soins-securitaires/Campagne_%20soins_securitaires_breve.pdf (Accessed Feb 9, 2019).
31. Pittet D, Widmer A. Hygiène des mains : nouvelles recommandations. Swiss NOSO. (2001) 8:25–32.
32. Comité d'examen sur la prévention et le contrôle des infections nosocomiales. D'abord, ne pas nuire. Les Infections Nosocomiales au Québec, un Problème Majeur de Santé, une priorité. Québec, QC: Gouvernement du Québec, Ministère de la Santé et des Services sociaux (2005). http://publications.msss.gouv.qc.ca/msss/fichiers/2005/05-209-01W.pdf (Accessed 9 Feb, 2019).
33. Groupe de travail en hygiène et salubrité. Guide de Gestion intégrée de la Qualité en Hygiène et Salubrité . Québec, QC: Gouvernement du Québec, Ministère de la Santé et des Services sociaux (2013). http://publications.msss.gouv.qc.ca/msss/fichiers/2013/13-602-02W.pdf (Accessed 9 Feb, 2019).
34. Groupe Hygiène et salubrité au regard de la lutte aux infections nosocomiales. Lignes Directrices en Hygiène et Salubrité: Analyse et Concertation . Québec, QC: Gouvernement du Québec, Ministère de la Santé et des Services sociaux, Direction générale de la coordination, du financement et de l'équipement; (2006). http://publications.msss.gouv.qc.ca/msss/fichiers/2006/06-602-01.pdf (Accessed 9 Feb, 2019).
35. Institut national de la santé publique du Québec. Surveillance Provinciale des Diarrhées à Clostridium Difficile au Québec: Protocole . Montreal, QC: INSPQ (2018). Available online at: https://www.inspq.qc.ca/sites/default/files/documents/infectionsnosocomiales/protocole_dacd_2018.pdf (Accessed 9 Feb, 2019).
36. Institut national de la santé publique du Québec. Surveillance Provinciale des Bactériémies à Staphylococcus au Québec: Protocole . Montreal, QC: INSPQ (2018). Available online at: https://www.inspq.qc.ca/sites/default/files/documents/infectionsnosocomiales/protocole_sarm_2018.pdf
37. Institut national de la santé publique du Québec. Surveillance Provinciale des Infections à Entérocoque Résistant à la Vancomycine au Québec: Protocole. Montreal, QC: INSPQ (2018). Available online at: https://www.inspq.qc.ca/sites/default/files/documents/infectionsnosocomiales/protocole_erv_2017.pdf (Accessed 9 Feb, 2019).
38. Institut national de la santé publique du Québec. Surveillance des Infections à Bacilles Gram Négatif Producteurs de Carbapénémases au Québec: Protocole . Montreal, QC: INSPQ; (2017). Available online at: https://www.inspq.qc.ca/sites/default/files/documents/infectionsnosocomiales/protocole_bgnpc_2017.pdf (Accessed 9 Feb, 2019).
39. Institut national de la santé publique du Québec. Surveillance Provinciale des Bactériémies Nosocomiales dans les Centres Hospitaliers de Soins Aigus du Québec: Protocole . Montreal, QC: INSPQ (2018). Available online at: https://www.inspq.qc.ca/sites/default/files/documents/infectionsnosocomiales/protocole_bactot_2018.pdf .
40. Public Health Agency of Canada. Routine Practices and Additional Precautions for Preventing the Transmission Of infection in Healthcare Settings . Ottawa, ON: PHAC, Centre for Communicable Diseases and Infection Control (2013). Available online at: https://www.canada.ca/content/dam/phac-aspc/documents/services/publications/diseases-conditions/routine-practices-precautions-healthcare-associated-infections/routine-practices-precautions-healthcare-associated-infections-2016-FINAL-eng.pdf (Accessed 9 Feb, 2019).
41. Comité sur les infections nosocomiales du Québec. Précisions sur la Gestion d'une Éclosion Majeure de Grippe Saisonnière Nosocomiale en Milieux de Soins: Lignes Directrices. Montreal, QC: Institut national de santé publique du Québec (2013). Available online at: https://www.inspq.qc.ca/pdf/publications/1727_PrecGestionEcloMajGrippeSaisonNosoMilieuxSoins.pdf (Accessed 9 Feb, 2019).
42. Drummond MF, Sculpher MJ, Torrance GW, O'Brien BJ, Stoddart GL. Methods for the Economic Evaluation of Health Care Programmes. 3rd ed. Oxford, UK: Oxford University Press (2005).
43. Drummond MF, Sculpher MJ, Claxton K, Stoddart GL, Torrance GW. Methods for the Economic Evaluation of Health Care Programmes . 4th ed. Oxford, UK: Oxford University Press (2015).
44. Finkler SA. Total quality management: measuring costs of quality. Hosp Cost Manag Account. (1993) 5:1–6.
PubMed Abstract
45. Finkler SA. Measuring the costs of quality. Hosp Cost Manag Account. (1996) 7:1–6.
46. Ogunfiditimi F, Takis L, Paige VJ, Wyman JF, Marlow E. Assessing the productivity of advanced practice providers using a time and motion study. J Healthc Manag. (2013) 58:173–86. doi: 10.1097/00115514-201305000-00005
47. Zheng K, Guo MH, Hanauer DA. Using the time and motion method to study clinical work processes and workflow: methodological inconsistencies and a call for standardized research. J Am Med Inform Assoc. (2011) 18:704–10. doi: 10.1136/amiajnl-2011-000083
48. Fortin MF, Gagnon J. Fondements et Étapes du Processus de Recherche : Méthodes Quantitatives et Qualitatives . 3rd ed. Montreal, QC: Chenelière Éducation (2016).
49. Waltz CF, Strickland OL, Lenz ER. Measurement in Nursing and Health Research . 4th ed. New York, NY: Springer Publishing Company (2010).
50. Waltz C, Bausell RB. Nursing Research: Design, Statistics and Computer Analysis . Philadelphia, PA: F. A. Davis. (1981).
51. Comité sur les infections nosocomiales du Québec. Prévention et Contrôle de la Diarrhée Nosocomiale Associée au Clostridium difficile au Québec: Lignes Directrices Pour les Établissements de Soins . 3rd ed. Montreal, QC: Institut national de santé publique du Québec (2005). Available online at: https://www.inspq.qc.ca/pdf/publications/362-CDifficile-LignesDirectrices-3eEdition.pdf (Accessed 9 Feb, 2019).
52. Comité sur les infections nosocomiales du Québec. Mesures de Prévention et de Contrôle des Infections à Staphylococcus aureus Résistant à la Méthicilline (SARM) au Québec . 2nd ed. interim report. Montreal, QC: Institut national de santé publique du Québec (2006). Available online at: https://www.inspq.qc.ca/pdf/publications/489-MesuresPreventionControleSARM.pdf (Accessed 9 Feb, 2019).
53. Comité sur les infections nosocomiales du Québec. Mesures de Prévention et de Contrôle de l'entérocoque Résistant à la Vancomycine dans les Milieux de Soins Aigus: Avis et Recommandations . Montreal: Institut national de santé publique du Québec (2012). Available online at: https://www.inspq.qc.ca/pdf/publications/1555_MesuresPrevContEnteroResisVancomMilieuxSoinsQc.pdf (Accessed Feb 9 Feb, 2019).
54. Institut national de santé publique du Québec. Entérobactéries Productrices de Carbapénémases (EPC): Mesures de Prévention et de Contrôle Pour les soins Aigus. Knowledge transfer webinar led by Dr. Josée Massicotte, November 8, (2017). Available online at: https://www.inspq.qc.ca/formation/evenements/mer-11082017-1330-enterobacteries-productrices-de-carbapenemases-epc-mesures-de-prevention-et-de-controle-pour-les-soins-aigus (Accessed 9 Feb, 2019).
55. Arefian H, Vogel M, Kwetkat A, Hartmann M. Economic evaluation of interventions for prevention of hospital acquired infections: a systematic review. PLoS ONE. (2016) 11:e0146381. doi: 10.1371/journal.pone.0146381
56. Tchouaket E, Dubois CA, D'Amour D. The economic burden of nurse-sensitive adverse events in 22 medical-surgical units: retrospective and matching analysis. J Adv Nurs. (2016) 73:1696–711. doi: 10.1111/jan.13260
57. Loiselle CG, Profetto-McGrath J. Méthodes de Recherche en Sciences Infirmières: Approches Quantitatives et Qualitatives . Montreal, QC: Pearson ERPI (2007).
58. Brodaty T, Crépon B, Fougère D. Les méthodes microéconométriques d'évaluation et leurs applications aux politiques actives de l'emploi. Econ Previs. (2007) 177:93–118. doi: 10.3917/ecop.177.0093
CrossRef Full Text | Google Scholar
59. Rosenbaum PR, Rubin DB. The central role of the propensity score in observational studies for causal effects. Biometrika. (1983) 70:41–55. doi: 10.1093/biomet/70.1.41
60. Rubin DB, Stuart EA, Zanutto EL. A potential outcomes view of value-added assessment in education. J Educ Behav Stat. (2004) 29:103–16. doi: 10.3102/10769986029001103
61. Charlson ME, Pompei P, Ales KL, MacKenzie CR. A new method of classifying prognostic comorbidity in longitudinal studies: development and validation. J Chronic Dis. (1987) 40:373–83. doi: 10.1016/0021-9681(87)90171-8
62. Pan W, Bai H. Propensity score interval matching: using bootstrap confidence intervalsfor accommodating estimation errors of propensity scores. BMC Med Res Methodol. (2015) 15:53. doi: 10.1186/s12874-015-0049-3
63. Nichols A. Erratum and discussion of propensity-score reweighting. Stata J. (2008) 4:532–9. doi: 10.1177/1536867X0800800405
64. Tchouaket E, Brousselle A, Fansi A, Dionne PA, Bertrand E, Fortin C. The economic value of Quebec's water fluoridation program. J Public Health. (2013) 21:523–33. doi: 10.1007/s10389-013-0578-3
Keywords: economic analyses, costs, case control design, prevention and control, research protocol, prospective observational study, nosocomiai infection
Citation: Tchouaket EN, Sia D, Brousseau S, Kilpatrick K, Boivin S, Dubreuil B, Larouche C, Parisien N, Dubois C-A, Brousselle A and Borgès Da Silva R (2021) Economic Analysis of the Prevention and Control of Nosocomial Infections: Research Protocol. Front. Public Health 9:531624. doi: 10.3389/fpubh.2021.531624
Received: 23 April 2021; Accepted: 07 June 2021; Published: 07 July 2021.
Reviewed by:
Copyright © 2021 Tchouaket, Sia, Brousseau, Kilpatrick, Boivin, Dubreuil, Larouche, Parisien, Dubois, Brousselle and Borgès Da Silva. This is an open-access article distributed under the terms of the Creative Commons Attribution License (CC BY) . The use, distribution or reproduction in other forums is permitted, provided the original author(s) and the copyright owner(s) are credited and that the original publication in this journal is cited, in accordance with accepted academic practice. No use, distribution or reproduction is permitted which does not comply with these terms.
*Correspondence: Eric Nguemeleu Tchouaket, eric.tchouaket@uqo.ca
Disclaimer: All claims expressed in this article are solely those of the authors and do not necessarily represent those of their affiliated organizations, or those of the publisher, the editors and the reviewers. Any product that may be evaluated in this article or claim that may be made by its manufacturer is not guaranteed or endorsed by the publisher.
[Proposal for the nosocomial infection control of methicillin-resistant Staphylococcus aureus (MRSA)]
Affiliation.
- 1 Department of Microbiology, Kitasato University School of Hygienic Sciences, Japan.
- PMID: 1484403
- DOI: 10.3412/jsb.47.767
To determine the prevalence of methicillin-resistant Staphylococcus aureus (MRSA) in hospital environments, quantitative isolation of MRSA from environmental and human specimens was performed. It was found that as many as 6 x 10(4) colony forming units (CFU)/100cm2 of MRSA and also methicillin-resistant coagulase-negative Staphylococcus (MRCNS) were isolated from 1) 25-100% of the bed-mattresses tested in 10 out of 11 (90%) hospitals, and also 2) bed sheets for patients, floors of patient wards, laundry, bath-room, toilet and laundry storage-room. And seven and eight palms of 20 patients were contaminated with MRCNS and MRSA, respectively, and MRCNS contamination was revealed in six of medical staffs. These results indicate that hospital environments, especially the mattresses and hospital floors are highly contaminated with MRCNS and MRSA, and sanitation and cleanliness of mattresses and floors are necessary to prevent the dissemination of both MRCNS and MRSA in hospital.
Publication types
- English Abstract
- Research Support, Non-U.S. Gov't
- Cross Infection / microbiology
- Cross Infection / prevention & control*
- Disinfection*
- Environmental Microbiology*
- Medical Staff, Hospital
- Methicillin Resistance
- Staphylococcal Infections / microbiology
- Staphylococcal Infections / prevention & control*
- Staphylococcus aureus / isolation & purification*

IMAGES
VIDEO
COMMENTS
Nosocomial infections or healthcare associated infections occur in patients under medical. care. These infections occur worldwide both in developed and developing countries. Nosocomial infections ...
Strategies to Prevent Healthcare-Associated Infections. HCAIs (their prevention and control) are a significant global public health burden about which concerns have been raised from all healthcare stakeholders, including health professionals, patients, and the public. 45 - 48 Their impact has dramatically increased because of the advent of multidrug-resistant pathogenic microorganisms. 49 ...
Background An increasing number of patients requires extracorporeal membrane oxygenation (ECMO) for life support. This supportive modality is associated with nosocomial infections (NIs). This systematic review and meta-analysis aim to assess the incidence and risk factors of NIs in adult. Methods We searched PubMed, Scopus, Web of Science, and ProQuest databases up to 2022. The primary ...
Around 20-40% of nosocomial infections can be mainly attributed to cross-infection via the hands of healthcare personnel. Less frequently, patients can become colonized with nosocomial pathogens by direct contact with contaminated patient care equipment or contaminated surfaces in the healthcare environment [ 51 ].
We suggest to decision makers that by focusing on different aspects of nosocomial infections such as age, gender, causes, etc. that we mentioned in this study, comprehensive and practical programs can be used to prevent these infections. Reviews 2 This is a large meta-analysis evaluating the global prevalence of Hospital-acquired infection (HAI).
Abstract. Nosocomial infections, also known as hospital-acquired infections, pose a serious challenge to healthcare professionals globally during the Coronavirus disease 2019 (COVID-19) pandemic. Nosocomial infection of COVID-19 directly impacts the quality of life of patients, as well as results in extra expenditure to hospitals.
Demographics of study participants and patients' knowledge of nosocomial infections. A total of 102 patients gave consent to participate in this study of which 46.1% (47) were male, 27.5% (28 ...
Nosocomial infections (NCIs) have been associated with several adverse outcomes including extended hospitalization, persistent disability, heightened antimicrobial resistance, amplified socio-economic disruption, and elevated mortality rates. The adoption of infection prevention strategies has the greatest tendency to significantly reduce the risk and occurrence of NCIs among the population ...
Over the course of the COVID-19 pandemic in England, there has been evidence of nosocomial SARS-CoV-2 transmission to both patients and healthcare workers (HCWs) [1,2,3,4], with 0.5-1.25% of susceptible inpatients (55,000-90,000 patients) identified as having developed a nosocomial infection between 01-March-2020 and 01-Sept-2021 [5, 6].Several interventions have been introduced in ...
Infection prevention and control (IPC) is one of the most cost-effective interventions against antimicrobial resistance (AMR). Yet, IPC knowledge gaps often receive little prominence in AMR research agendas. In this article, we construct IPC research priorities, in order to draw attention to these critical research needs. We developed a 4-step framework to identify IPC knowledge gaps from ...
Intensive care units (ICUs) have contributed greatly to the survival of patients with trauma, shock states, and other life-threatening conditions1, 2, 3 but are associated with a greatly increased risk of nosocomial (hospital-acquired) infection. Rates of nosocomial infection in patients requiring more than 1 week of advanced life support within an ICU are three to five times higher than in ...
Citation 2 The importance of hospital-acquired infections goes beyond its impact on morbidity and mortality figures in any country, and has profound economic implications. According to Robert, acquisition of a nosocomial infection can prolong duration of hospitalization, increase the costs of health care, and place a serious economic burden on ...
Introduction. Nosocomial infections (NIs) or healthcare associated infections are infections developed during the process of getting health care which occures 48 h after hospital admission without any evidence that the infection was existing at the time of hospital admission (1-3).The types of health care associated infections are catagorised as Catheter-associated urinary tract infections ...
Overall, 15.7% showed good knowledge while most patients showed fair knowledge (71.6%) and a negative perception (51%) of nosocomial infections. There was a significant diference in patients ...
about 1.7 million cases of hospital -acquired infections per year in the U.S ... to infection control research with nursing and midwifery students. Journal of Clinical Nursing, 22, ... source of nosocomial infections. American Journal of Infection Control, 39(7), 555-559. Research Proposal. Literature Review of Nosocomial Infections Passed ...
Background In the Yemeni healthcare setting, basic infection control measures are necessary to reduce the rates of hospital-associated infections. Methods A descriptive cross-sectional study was conducted from April to May 2016. A non-probability sample of 100 nurses working in the private hospitals located in the capital city of Yemen was selected as study participants. A 45-item ...
REVIEW ON NOSOCOMIAL INFECTIONS. A. Nautiyal, R. Sharma, +2 authors. Samir Bhargava. Published 2015. Medicine. Nosocomial infections (NIs) are most contributing factors affecting the patients during the hospital visit and has become challenging for the healthcare professionals, also increasing the health…. Expand.
The research proposal will be submitted to the Northern Caribbean University Institutional Review Board for approval. FACTORS CONTRIBUTING TO NOSOCOMIAL INFECTION IN NEONATES 19 Data Collection Procedures Phase 1 Quantitative The first, quantitative phase of the study will focus on identifying internal and external factors contributing to ...
Nosocomial infections also referred to as healthcare-associated infections (HAI), are infection(s) acquired during the process of receiving health care that was not present during the time of admission. They may occur in different areas of healthcare delivery, such as in hospitals, long-term care facilities, and ambulatory settings, and may also appear after discharge. HAIs also include ...
Conclusion: The HCIA infection rate was 15%, and urinary tract infections (UTI) was the commonest hospital acquired infection in our ICU. Klebsiella oxytoca and Staphylococcus aureus were the ...
Background: Nosocomial infections (NIs) are among the main preventable healthcare adverse events. Like all countries, Canada and its provinces are affected by NIs. In 2004, Ministry of Health and Social Services (MSSS) of Quebec instituted a mandatory surveillance NI program for the prevention and control (NIPC) in the hospitals of the province.
Before presenting the knowledge transfer strategies, a brief overview of the different stakeholders involved in this proposal is in order. ... Economic Analysis of the Prevention and Control of Nosocomial Infections: Research Protocol. Front. Public Health 9:531624. doi: 10.3389/fpubh.2021.531624. Received: 23 April 2021; Accepted: 07 June 2021
To determine the prevalence of methicillin-resistant Staphylococcus aureus (MRSA) in hospital environments, quantitative isolation of MRSA from environmental and human specimens was performed. It was found that as many as 6 x 10(4) colony forming units (CFU)/100cm2 of MRSA and also methicillin-resis …