Click through the PLOS taxonomy to find articles in your field.
For more information about PLOS Subject Areas, click here .
Loading metrics
Open Access
Peer-reviewed
Research Article

Prevalence and associated factors of malaria in children under the age of five years in Wogera district, northwest Ethiopia: A cross-sectional study
Roles Conceptualization, Data curation, Formal analysis, Methodology, Software, Supervision, Writing – original draft
Affiliation Department of Epidemiology and Biostatics, Institute of Public Health, College of Medicine and Health Sciences, University of Gondar, Gondar, Ethiopia
Roles Conceptualization, Data curation, Formal analysis, Methodology, Software, Writing – original draft
Affiliation Wogera District Health Office, North Gondar Zone, Gondar, Ethiopia
Roles Data curation, Formal analysis, Methodology, Software, Writing – review & editing
* E-mail: [email protected]
Affiliation School of Nursing and Midwifery, Haramaya University, Harar, Ethiopia

- Adino Tesfahun Tsegaye,
- Andualem Ayele,
- Simon Birhanu
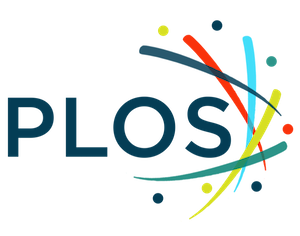
- Published: October 11, 2021
- https://doi.org/10.1371/journal.pone.0257944
- Reader Comments
Malaria is a major public health problem in sub-Saharan Africa, and children are especially vulnerable. In 2019, an estimated 409,000 people died of malaria, most (274,000) were young children and 94% of the cases and deaths were in Africa. Prior studies in Ethiopia focused on the adult population and high transmission areas. Hence, this study aimed to determine the prevalence and associated factors of malaria in children under five years in low transmission areas.
A facility-based cross-sectional study was conducted among 585 under-five children who attended public health facilities in the Wogera district from September to October, 2017. Health facilities were selected by stratified cluster sampling, and systematic random sampling was held to select study participants from the selected facilities. Multivariable logistic regression was used to identify correlates of malaria.
Of 585 children who provided blood samples, 51 (8.7%) had malaria. The predominant Plasmodium species were P . falciparum 33 (65%) and P . vivax 18 (35%). Regularly sleeping under long-lasting insecticide treated nets (LLIN) was associated with decreased odds of malaria (AOR = 0.08, 95% CI: 0.01–0.09), and an increased odds of malaria was observed among children who live in households with stagnant water in the compound (AOR = 6.7, 95% CI: 3.6–12.6) and children who stay outdoors during the night (AOR = 5.5, 95% CI: 2.7–11.1).
The prevalence of malaria in the study population was high. Environmental and behavioral factors related to LLIN use remain potential determinants of malaria. Continued public health interventions targeting proper utilization of bed nets, drainage of stagnant water, and improved public awareness about reducing the risk of insect bites have the potential to minimize the prevalence of malaria and improve the health of children.
Citation: Tsegaye AT, Ayele A, Birhanu S (2021) Prevalence and associated factors of malaria in children under the age of five years in Wogera district, northwest Ethiopia: A cross-sectional study. PLoS ONE 16(10): e0257944. https://doi.org/10.1371/journal.pone.0257944
Editor: Benedikt Ley, Menzies School of Health Research: Charles Darwin University, AUSTRALIA
Received: December 10, 2020; Accepted: September 14, 2021; Published: October 11, 2021
Copyright: © 2021 Tsegaye et al. This is an open access article distributed under the terms of the Creative Commons Attribution License , which permits unrestricted use, distribution, and reproduction in any medium, provided the original author and source are credited.
Data Availability: All data generated or analyzed during this study is included in this published article.
Funding: The author(s) received no specific funding for this work.
Competing interests: The authors have declared that no competing interests exist.
Abbreviations: AIDS, Acquired Immune Deficiency Syndrome; AOR, Adjusted Odds Ratio; API, Annual Parasite Incidence; CI, Confidence interval; DRC, Democratic Republic of Congo; EMIS, Ethiopia Malaria Indicator Survey; HIV, Human Immune Virus; IRS, Indoor Residual Spraying; LLITN, Long Lasting Insecticide Treated Nets; OPD, Outpatient Department; RDT, Rapid Diagnostic Test; SNNPR, Southern Nation Nationalities and People Region
In sub-Saharan Africa, infectious diseases remain the primary public health threat [ 1 ]. Malaria is one of the commonest infections, disproportionately affecting children and pregnant women. In 2019, an estimated 409,000 people died of malaria. Most (274,000) were young children, and 94% of the infections and deaths occurred in Africa [ 2 , 3 ]. Although several Plasmodium species are responsible for malaria, only a few of them cause most infections.
In 2018, Plasmodium falciparum accounted for 99.7% of estimated malaria cases in the World Health Organization (WHO) African Region, 50% in the WHO South-East Asia Region, 71% in the Eastern Mediterranean, and 65% in the Western Pacific. P . vivax is the predominant parasite in the WHO Region of the Americas, representing 75% of malaria cases [ 3 ]. In Ethiopia, peak malaria transmission occurs between September and December in most parts, following the rainy season from June to August, mainly affecting young children, and P . falciparum and P . vivax are the major malaria parasites [ 4 , 5 ].
Children under five years are one of the most vulnerable groups affected by malaria. Severe anemia, hypoglycemia and cerebral malaria are features of severe malaria more commonly seen in children than in adults [ 6 ]. Children’s susceptibility to diarrhea, respiratory infections, and other illnesses increases when they develop repeated malaria infections [ 7 ]. An estimated 2% of children who recover from cerebral malaria develop learning impairments and disabilities, including epilepsy and spasticity, resulting from the brain damage caused by the infection [ 8 ]. In general, malaria could cause severe outcomes in children in three major ways: First, since children do not usually have acquired immunity, they are more likely to develop severe malaria manifested by seizures or coma (cerebral malaria), which can cause emergency death. Second, through complications related to repeated infections such as anemia. Finally, it causes low birth weight when it happens during pregnancy and increases the risk of death in the first month of life [ 4 ].
According to the WHO 2016 report, the global prevalence of malaria among under-five children was 16% [ 9 ]. In the same year, the prevalence in Ethiopia was 0.6% [ 5 ].
The Ethiopian government developed a National Malaria Control Strategy (NMSP) for the years 2017–2020 that was envisioned to be aligned with the country’s four-year health sector transformation plan (HSTP) 2015/16–2019/20. The proposed goals for the 2017–2020 NMSP include: maintaining near-zero malaria deaths (< = 1 death per 100,000) by 2020, reducing malaria cases by 40% by 2020, and eliminating malaria from Ethiopia by 2030 [ 2 , 5 ].
Even though malaria is one of the leading causes of under-five morbidity and mortality in Ethiopia, prior studies focused only on the adult population and were done in malaria-endemic transmission areas. Nevertheless, it is a potential threat in non-endemic regions [ 5 ]. There has been limited information on the epidemiology of malaria among under-five children living in low malaria transmission areas [ 10 ]. This study aimed to close a critical knowledge gap by assessing the prevalence and determinants of malaria among under-five years old children living in low malaria transmission areas. The findings from this study will inform public health and clinical decision-making and will initiate further investigations.
Methods and materials
Study setting and design.
A health facility-based cross-sectional study was conducted from September to October, 2017 in the Wogera district. Wogera is one of the districts in the North Gondar zone. It has an average altitude of greater than 2050 meters above sea level, with an estimated total population of 274,384, of which 37,152 (13.5%) are children under five years old. The district has 42 rural and one city kebeles (the smallest administrative unit ), of which 15 kebeles (35.7%) are malaria-endemic. In the Wogera district, there was 1 hospital, 10 health centers, 42 health posts, and 4 private health institutions. It shares borders with Dabat and Tach-Armacho in the North, Misrak-Belesa and Janamora in the West, Merab Belesa in the South and Lay-Armacho in the East [ 11 ]. According to the new stratification of malaria risk in the country, the district is under the classification of low transmission areas with expected sporadic epidemics every five years [ 5 ]. Despite that, the report of the district health office indicates that malaria is one of the leading causes of morbidity both in adults and under-five children.
Study participants
All children whose age was five years or below visiting the selected health facilities during the study period were the source population.
Sample size estimation
The calculated sample size was 266 using a single population proportion formula as well as a power approach using a double proportion formula based on previous studies [ 12 ]. Adding a 10% non-response rate and a design effect of two, the final sample size was 585.
Sampling procedure
First, we stratified the health facilities as malaria-endemic and non-endemic based on their altitude. Then, we randomly selected five health centers (Ambagiorgis HC, Gedebgie HC, Selarie HC, Tirgosgia HC, and Chichiki HC) and one hospital (Wogera hospital) from the non-endemic clusters by using a lottery method. The calculated 585 sample size was proportionally allocated to the selected health facilities. Finally, a systematic random sampling technique was used to reach under-five clients who attended the selected health facilities.
Data collection tools and procedures
A structured questionnaire was used for data collection. The tool contained socio-demographic, environmental, and malaria prevention related questions. The questionnaire was initially developed in English and translated into Amharic for data collection. A face-to-face interview of the parents/guardians of the under-five children was conducted to collect the data.
After the interview was completed, following the Federal Democratic Republic of Ethiopia Ministry of Health National Malaria Guidelines, blood was taken from a finger prick to prepare thick and thin blood film smears [ 13 ]. Using a sterile lancet, a finger prick was performed, and 5 micro liters of whole blood was drawn from each child included in the sampling regardless of signs and symptoms of malaria using a capillary tube. The blood smears were prepared on microscope slides and stained using 10% Giemsa to be examined under 100x microscopes for the presence of malaria parasites. The thick smear was used to determine whether the malaria parasites were present or absent and the thin smear was used to identify the type of Plasmodium species. A positive result was defined as the presence of one or more asexual stages (trophozoite, ring stage, merozoite, or gametocyte) of plasmodium [ 14 ].
Data quality assurance
Six laboratory technicians (1 from each health facility) and two supervisors from the district health office were trained for two days by the investigators. Each filled questionnaire was checked thoroughly for completeness and consistency, and the necessary feedback was given to data collectors. Recruitment was preceded by obtaining informed written consent from parents or caregivers of the children. To assure the quality of the microscopic examinations, all positive and randomly selected five percent of the negative slides were checked blindly by another experienced medical laboratory technologist.
Operational definitions
Bed net utilization: was self-reported ownership and regular use of bed nets. A 15-day recall period was used to measure whether each child regularly slept under long lasting insecticide treated nets (LLIN) or not.
Malaria : was defined as a positive thin or thick blood film for the Plasmodium parasite.
Data processing and analysis
After data collection, data were entered using Epi info version 7 and then exported to SPSS version 20 for analysis. The correlates of malaria were identified using bivariate and multivariate logistic regression models. Variables which had a P-value of <0.2 in the bivariable regression were included in the multivariable logistic regression analysis. A P-value <0.05 was considered to determine statistical significance. Finally, adjusted odds ratios (AOR) with a 95% confidence interval (CI) were used to determine the strength of association of variables.
Ethical approval and consent to participate
Ethical approval was obtained from the ethical review committee of the Institute of Public Health, College of Medicine and Health Science, University of Gondar, Ethiopia. Permission was gained from the Amhara Regional Health Bureau, North Gondar health department, and Wogera health office. The caregivers were given detailed explanations about the study’s objectives, procedures, and potential risks and benefits, and written consent was obtained following that. The interview of each study participant took place in a separate room after the children gave blood samples. Appropriate treatment was given to children who tested positive.
Socio-demographic characteristics of study participants
In this study, 585 children from five health centers and one district hospital participated: Gedebgie health center (HC) 178 (30.4%), Ambagiorgis HC 114 (19.5%), Tirgosgia HC 111 (19%), Selarie HC 98 (16.8%), Ambagiorgis hospital 37 (6.3%) and Chichiki HC 47 (8%). Three hundred twenty-three (55.2%) were males and 218 (37.3%) were below 12 months. About 370 (63%) of the respondents live in rural areas, and 305 (54%) of the caregivers can not read and write ( Table 1 ).
- PPT PowerPoint slide
- PNG larger image
- TIFF original image
https://doi.org/10.1371/journal.pone.0257944.t001
Indoor Residual Spraying (IRS), Long Lasting Insecticide Treated Nets (LLIN), and environmental characteristics of study participants
Only 131 (22.4%) of the respondents had LLIN. Of the respondents who possessed LLIN, 90% of respondents reported that their children had regularly slept under LLIN in the last 15 days ( Table 2 ).
https://doi.org/10.1371/journal.pone.0257944.t002
Magnitude of Malaria
In this study, the prevalence of malaria by microscopy among under-five children was 8.7% (51). There was a considerable variation in the prevalence rate between the health facilities, ranging from 0% at Wogera hospital to 21% at Selarie health center ( Table 3 ).
https://doi.org/10.1371/journal.pone.0257944.t003
Factors associated with malaria infection
Both bivariable and multivariable binary logistic regression analyses were done to identify the determinants of malaria infection. In bivariate analysis, factors with a P-value of <0.2 were: place of residence, stagnant water around the home, staying outside during the night, possession of an LLIN and regularly sleeping under an LLIN for the last 2 weeks. However, place of residence, sex of the child, age of the child, age of the mother/guardian, educational status of the mother/guardian, presence of radio/television, child having a regular sleeping area, construction material of the house and incidence of IRS within six months had a P-value of >0.2 in the bivariate analysis and were not included in the final model.
In the final adjusted model, children who stayed outside at night had 5.5 times higher odds of malaria infection than children who did not stay outside at night (AOR = 5.5, 95% CI: 2.7–11.1). Children who regularly slept under a LLIN had 92% lower odds of infection than those who did not sleep regularly (AOR = 0.08, 95% CI: 0.08, 0.09). Children who lived in households with close to stagnant water had—4 times higher odds of malaria infection than children who did not live in those homes with nearby stagnant water (AOR = 4, 95% CI: 1.9, 8.1) ( Table 4 ).
https://doi.org/10.1371/journal.pone.0257944.t004
In this study, we estimated the prevalence of malaria among under-five children in the low-risk area and its determinant factors, and the results showed that the malaria prevalence in under-five children was 8.7%, which is in line with the study conducted in Dilla, Southern Ethiopia, where the prevalence of malaria in under-five children was identified to be 7.1% [ 15 ] and a study of analysis of the five-year trend of malaria at Bichena primary hospital, Amhara Region, Ethiopia, where the overall prevalence of malaria was 9.28% [ 16 ].
This finding is much higher when compared to the national malaria indicator survey in 2015 that identified a prevalence of 0.6% among under-five children [ 5 ] and another study conducted in four regional states in Ethiopia, where the prevalence was 4.6% [ 17 ]. This could be due to the difference in methodology used, and also, it might be due to the season when the studies were conducted. Malaria increases from September to December (major transmission season). However, this finding is lower when compared to the global magnitude of malaria among under-five children, which is about 16% [ 9 ] and studies conducted in East Shewa 18.9% [ 18 ], Tanzania 26.3% [ 19 ], Sudan 22% [ 20 ], Uganda 19.5% [ 21 ], and Mozambique 33% [ 22 ]. Those studies were conducted in low land areas, and the difference could be due to a study population difference in the case of a study conducted in Mozambique in which the study population was people with comorbidity.
In Ethiopia, there is spatial and temporal variability in the occurrence of malaria. The current findings also demonstrated similar spatial variations in the proportion of Plasmodium species, with the predominant occurrence of P . falciparum infections at 65% over P . vivax at 35%. This estimate is approximately similar to the study conducted by the Carter Center in Amhara, Oromia, and Southern Ethiopia, where P . falciparum accounted for 56.5% and P . vivax for 43.5% [ 17 ], and a 7-year trend of malaria study done at primary health facilities in Northwest Ethiopia P . falciparum accounted for 15.6% of the participants, which was threefold higher than P . vivax in the seven-year trend [ 23 ]. However, other studies reported a different proportion, such as those conducted in East Shewa ( P . falciparum = 41.2%, P . vivax = 57.1 and Mixed = 1.8%) [ 19 ]; Hadiya ( P . falciparum = 25.5%, P . vivax = 71.7% and Mixed = 2.8%) [ 24 ] and Dilla town ( P . falciparum = 26.8%, P . vivax = 62.5%, and Mixed = 10.7%) [ 15 ]. The variability could be related to the wide climatic diversity between the areas.
Sleeping under LLIN for the last two weeks was found to be protective against malaria. This evidence is supported by other similar studies conducted in East Shewa [ 18 ], Amhara, Oromia, and SNNRP [ 17 ], Dilla [ 15 ], Ethiopia [ 25 ], Ghana [ 26 ], and Uganda [ 21 ]. It was evident that using ITN properly decreased mosquito bites, and thereby decreased malaria infection.
In this study, malaria was highly prevalent among children living in households with stagnant water in the compound compared to their counterparts. This is consistent with a facility-based cross-sectional study conducted in a low transmission area of the Hadiya zone, south Ethiopia [ 24 ]. This is because water collection is one of the favorable conditions for mosquito breeding, which in turn increases malaria transmission. Staying outside during the night showed a statistically significant association with malaria. Staying outside during the night increases the probability of mosquito bites due to the nocturnal nature of the mosquito.
Limitations of the study
As a limitation of this study, since it is a cross-sectional study, it only captures the point prevalence and can not account for seasonal trends in transmission. All surveys are self-report with no confirmation of bed net ownership or use. RDTs with PCR confirmed were not conducted, nor are there details on the life stages of detected parasites observed–gametocytemia, parasitemia.
The prevalence of malaria in under-five children attending health care facilities in Wogera district was high. Regularly sleeping under a bed net, staying outside during the night, and stagnant water around the household were the main correlates of malaria. Focusing on LLIN distribution, environmental management, and changing attitudes towards malaria prevention and control through health education would help minimize the burden of malaria.
Acknowledgments
We would like to thank the Wogera health bureau, the study participants, data collectors, and supervisors who participated in this study for their commitment and cooperation.
- View Article
- PubMed/NCBI
- Google Scholar
- 2. Communicable disease control (CDC). Malaria Impact of Malaria. 2019. https://www.cdc.gov/malaria/malaria_worldwide/impact.html .
- 3. World health organization (WHO). Fact sheet malaria. 30 November 2020. https://www.who.int/news-room/fact-sheets/detail/malaria .
- 4. President’s Malaria Initiative, Ethiopia. Malaria Operational Plan FY. 2019. https://www.pmi.gov/docs/default-source/default-documentlibrary/malariaoperational-plans/fy19/fy-2019-ethiopia-malaria-operational-plan.pdf?sfvrsn=3 .
- 5. Ethiopia National Malaria Indicator Survey. 2015. https://www.ephi.gov.et/images/pictures/download2009/MIS-2015-FinalReport-December-_2016.pdf .
- 6. World health organization (WHO). Malaria in children under five. 2019. https://www.who.int/malaria/areas/high_risk_groups/children/en/ .
- 9. World health organization (WHO). World malaria report. 2016. https://www.who.int/malaria/publications/world-malaria-report-2016/report/en/ .
- 11. Wogera Woreda health bureau Annual Report. 2016.
- 13. Federal Democratic Republic of Ethiopia Ministry of Health. National Malaria Guidelines fourth edition. November 2017 Addis Ababa. https://www.humanitarianresponse.info/sites/www.humanitarianresponse.info/files/documents/files/eth_national_malaria_guidline_4th_edition.pdf .
- 14. Centres for disease control. Malaria Diagnostic Tests.2017. https://www.cdc.gov/malaria/diagnosis_treatment/diagnostic_tools .
- 17. The Carter Center. Prevalence and Risk Factors for Malaria And Trachoma In Ethiopia.Report of Malaria and Trachoma Survey in Ethiopia.2007.
- 19. Mushashu u. Prevalence of malaria infection among under-fives and the associated factors in muleba district-kagera region tanzania. 2012.
- Research article
- Open access
- Published: 29 April 2020
A systematic review of changing malaria disease burden in sub-Saharan Africa since 2000: comparing model predictions and empirical observations
- Alice Kamau 1 , 2 ,
- Polycarp Mogeni 1 ,
- Emelda A. Okiro 1 ,
- Robert W. Snow 1 , 2 &
- Philip Bejon 1 , 2
BMC Medicine volume 18 , Article number: 94 ( 2020 ) Cite this article
4920 Accesses
11 Citations
46 Altmetric
Metrics details
The most widely used measures of declining burden of malaria across sub-Saharan Africa are predictions from geospatial models. These models apply spatiotemporal autocorrelations and covariates to parasite prevalence data and then use a function of parasite prevalence to predict clinical malaria incidence. We attempted to assess whether trends in malaria cases, based on local surveillance, were similar to those captured by Malaria Atlas Project (MAP) incidence surfaces.
We undertook a systematic review (PROSPERO International Prospective Register of Systematic Reviews; ID = CRD42019116834) to identify empirical data on clinical malaria in Africa since 2000, where reports covered at least 5 continuous years. The trends in empirical data were then compared with the trends of time-space matched clinical malaria incidence from MAP using the Spearman rank correlation. The correlations ( rho ) between changes in empirically observed and modelled estimates of clinical malaria were displayed by forest plots and examined by meta-regression.
Sixty-seven articles met our inclusion criteria representing 124 sites from 24 African countries. The single most important factor explaining the correlation between empirical observations and modelled predictions was the slope of empirically observed data over time ( rho = − 0.989; 95% CI − 0.998, − 0.939; p < 0.001), i.e. steeper declines were associated with a stronger correlation between empirical observations and modelled predictions. Factors such as quality of study, reported measure of malaria and endemicity were only slightly predictive of such correlations.
Conclusions
In many locations, both local surveillance data and modelled estimates showed declines in malaria burden and hence similar trends. However, there was a weak association between individual surveillance datasets and the modelled predictions where stalling in progress or resurgence of malaria burden was empirically observed. Surveillance data were patchy, indicating a need for improved surveillance to strengthen both empiric reporting and modelled predictions.
Peer Review reports
The burden of malaria has been reported to have declined across sub-Saharan Africa (SSA) [ 1 , 2 , 3 ]. However, uncertainty on the magnitude of this transition remains, and it is unclear to what extent the decline is spatially uniform across SSA [ 4 , 5 , 6 ]. Our current understanding of the declining burden of malaria across much of SSA has relied on modelled estimations based on historical epidemiological data, predictions in time and space based on sparse parasite prevalence data, and presumed impacts of interventions [ 1 , 7 , 8 ].
The traditional metric for classifying the quantity of malaria in a given location, or endemicity, has been parasite prevalence (referred to in literature as the “parasite rate (PR)”), which is derived from community-based surveys of infection [ 2 , 9 ]. In current models of malaria disease burden in African, empirical data on 27,573 spatially and temporally unique PR observations are first modelled in time and space using a range of environmental, population and intervention covariates to provide approximately nine hundred thousand 5 × 5 km gridded surfaces of estimated malaria prevalence for every year between 2000 and 2015 [ 1 ]. These surfaces are then used in conjunction with limited historical epidemiological data on disease incidence, malaria-specific mortality and case-fatality rates [ 1 , 8 ] to predict both malaria clinical incidence and mortality at a 5 × 5 km grid surface across SSA based on an estimated function of PR [ 1 , 8 ]. The uncertainty in the predictions of parasite prevalence and disease burden was measured through out-of-bag sampling and reported as Bayesian credible intervals [ 1 , 8 ]. The World Health Organization (WHO) has adopted these methods to estimate malaria burden in SSA countries where routine data is thought to be unreliable. During the estimations for 2017, the WHO [ 10 ] included adjusted and unadjusted empirical routine data for Botswana, Cape Verde, Comoros, Ethiopia, Namibia, Rwanda, Sao Tome and Principe, South Africa and Swaziland (Eswatini). In 2018, the list of countries where routine data was used expanded to include Eritrea, the Gambia, Madagascar, Mauritania, Mayotte, Senegal and Zimbabwe [ 11 ]. In 2019, the list was extended to include Djibouti [ 3 ].
Geostatistical and epidemiological models are rarely compared with empirical surveillance data. Here, the objective was to conduct a systematic review of published observations of temporal changes in malaria disease burden in SSA since 2000, updated from previous reviews [ 12 , 13 , 14 ], and compare this with the modelled predictions of changing clinical incidence of malaria over the same time period. In the Malaria Atlas Project (MAP) models, empirical data on PR observations are first modelled in time and space to provide estimated malaria prevalence. These surfaces of PR are then used to predict malaria clinical incidence at 5 × 5 km resolutions across Africa for each year between 2000 and 2015. We used these modelled predictions of changing clinical incidence of malaria to compare spatially matched clinical cases identified in our literature review over the same time period. While there are factors that might impact on malaria cases recorded by health facilities, we attempted to assess whether trends in malaria cases are in agreement with MAP incidence surfaces.
Search strategy and selection criteria
A systematic review and meta-analysis was conducted using PRISMA guidelines [ 15 ]. The protocol was registered in the PROSPERO International Prospective Register of Systematic Reviews (ID = CRD42019116834).
A literature search was performed in PubMed, MEDLINE, EMBASE, Web of Science and reference lists of publications between January 2000 and August 2018 on the test positivity rate/incidence of clinical malaria in SSA. A search strategy combining relevant terms and the names of the African countries was applied (Table 1 ). Studies considered included published papers in peer-reviewed journals, reports, book chapters and theses. We also manually screened citations of relevant articles to identify additional studies. In addition, we contacted authors of published hospital data to provide help with annual data not possible to extract directly from the published source.
For studies to be included in the review, they had to fulfill the following criteria: (i) articles reporting data from SSA; (ii) articles that included data on the following outcomes of interest: clinical malaria test positivity rate, malaria case period prevalence, incidence from facility-based surveillance, or community-based disease surveillance; and (iii) articles reporting continuous data for five or more years since 2000. We excluded studies that (i) reported data from countries where in 2017 the WHO used empirical routine data for morbidity estimation, and where most countries are near malaria elimination: Cape Verde, Comoros, Sao Tome and Principe, South Africa and Swaziland (Eswatini) [ 2 , 10 ]; (ii) reported only repeat cross-sectional survey data on parasite prevalence or vector sporozoite rates in the community; (iii) reported modelled disease projections without empirical data; and (iv) reported only on verbal autopsy defined malaria mortality. For studies published in more than one report, the most comprehensive (years covered or availability of data for extraction) report was included.
The study selection process began by screening titles and abstracts retrieved from different electronic databases. We then reviewed the full text of eligible articles and compared all the retrieved articles with those included in the previous three reviews [ 12 , 13 , 14 ] to ensure no studies were missed.
Data abstracted
Two authors (AK and PM) independently screened the articles for inclusion and extracted data on general information, i.e. first author name, year of publication, study location, country, source of data (hospital surveillance, cohort studies or clinic registers), sample size, age range, number of cases, test positive rate (defined as the number of positive malaria tests per 100 tests conducted), or incidence rate of malaria from tables, figures, text or summary data in the articles. Disagreements between reviewers were resolved through consensus. We used Gwet’s AC1 statistic to assess the inter-rater agreement for study inclusion [ 16 ].
Critical appraisal
The quality of all included studies was assessed using the Joanna Briggs Institute Prevalence Critical Appraisal Tool [ 17 ]. Each study was assessed on 10 items; a score of 10% (yes) or 0% (no/unclear) was assigned and was summed across all items to generate an overall quality score that ranged from 0 to 100% (Additional file 1 ). Based on the overall score, we used two tertiles to split the studies into three groups. Studies were classified as having a high (< 34%), moderate (34–67%) or low (> 67%) risk of bias.
Geographic information
Geo-referencing was undertaken through matching to previous health facility geo-coded master facility lists [ 18 ] and where not available using Google Earth or coordinates provided in the original publication. We assumed a representative study area for purposes of matching to modelled predictions of a radial distance of 30 km for each hospital [ 19 ], 10 km for large health centres and 5 km for dispensaries/clinics/health posts [ 20 ].
Data analysis
Empirical data extracted from published reports were annualized, classified as hospital in-patient admissions, out-patient case burdens or community cohort incidence. An African region was assigned to each study based on the country of enrollment. The average endemicity at the start of the study’s surveillance period was defined using the MAP predicted Plasmodium falciparum parasite rate in children aged 2–10 years per site [ 1 ]. Study-level characteristics included the following: quality of study, geographic regions, source of data, reported measure of malaria, average starting parasite prevalence in children aged 2–10 years, sum of residuals, slope and sample size.
For each study, we time-space matched empirically recorded clinical incidence/test positivity rate of P. falciparum with modelled predictions of clinical malaria incidence developed by the MAP [ 1 ]. We extracted the mean clinical malaria incidence for all pixels in the specified raster that fell within the circular buffer for each geo-reference point for each year of the empirical surveillance data.
The correlation ( rho ) between changes in empirical clinical incidence/test positivity rate and modelled predictions of clinical malaria incidence was assessed using Spearman’s Rank correlation. Correlations were then classified as strong positive association ( rho ≥ 0.6), moderate positive association (0.2 < rho < 0.6) or weak association ( rho ≤ 0.2). A random effects meta-analysis was used to summarize the rho (Additional file 2 ); meta-analysis methods weight each study as a function of the between-study variance and within-study variance. We assessed the level of heterogeneity using Cochran’s Q statistic and I 2 . We used the forest plot to display the rho and the confidence intervals. Outliers were identified using the leave-one-out method, whereby we reran the meta-analysis iteratively removing studies. We used the funnel plots and Egger’s test to assess for publication bias [ 21 ].
To explore possible sources of heterogeneity, we performed meta-regression and sub-group analysis. In the sub-group analysis, the pooled correlation in each sub-group and within-group heterogeneity were obtained. In the meta-regression analysis, we examined the relationship between the study-level characteristics and the correlation of the two metrics using study as the unit of analysis [ 22 ]. Data analysis was performed using Stata, version 13 (Stata Corporation, College Station, TX) and R version 3.5.1 (R Core Team (2018), Vienna, Austria).
The initial search yielded 4203 articles (Fig. 1 ). We then examined previous reviews [ 12 , 13 , 14 ] and found additional four articles that were relevant to our review which had been missed using our search terms [ 23 , 24 , 25 , 26 ]. We removed 2098 articles as they were duplicates between different electronic databases. After screening titles and abstracts of the remaining 2109 articles, we excluded 1994 articles because they did not fulfil the inclusion criteria. Following a full-text review of 115 articles, a further 48 articles were excluded because they either reported data from the same site, reported smoothed data, reported parasite prevalence or did not meet the complete 5-year restriction (Fig. 1 ). Finally, the study included data from 67 articles, where in some cases one publication reported data from more than one site. Three articles reported data from the same site but in different time periods, and/or from in-patient or out-patient records [ 27 , 28 , 29 ]. We obtained primary data, not possible to extract directly from the published source, for six publications related to hospital admissions at 24 sites across three countries (Malawi, Uganda and Kenya) (Additional file 3 ) [ 19 , 30 , 31 , 32 , 33 , 34 ]. The level of agreement for study selection between the two authors was 96.4% (Gwet’s AC1 = 0.961), and disagreements were resolved after discussion.
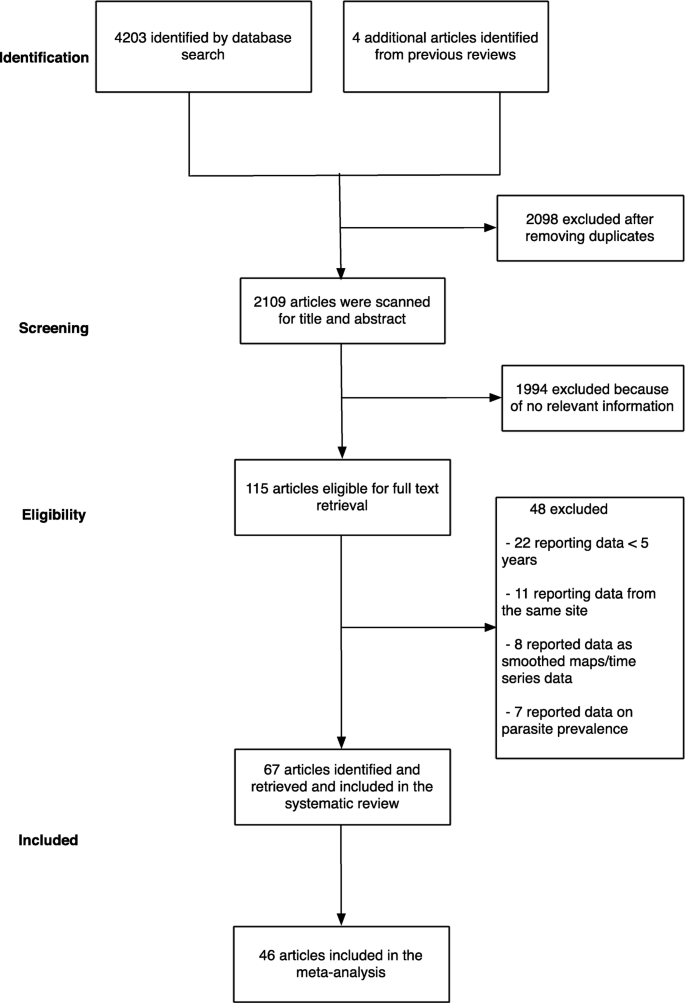
A summary flow of study selection process
Nineteen (28%), twenty-seven (41%) and twenty-one (31%) articles were classified as having high, moderate and low risk of bias, respectively. The 67 articles resulted in a total of 124 geo-referenced locations where data was available for five or more years, covering 24 African countries since 2000 (Fig. 2 ). Data was obtained from the out-patient records (41%), in-patient records (30%), combined out-patient and in-patient records (27%), and cohort studies (2%) (Table 2 ).
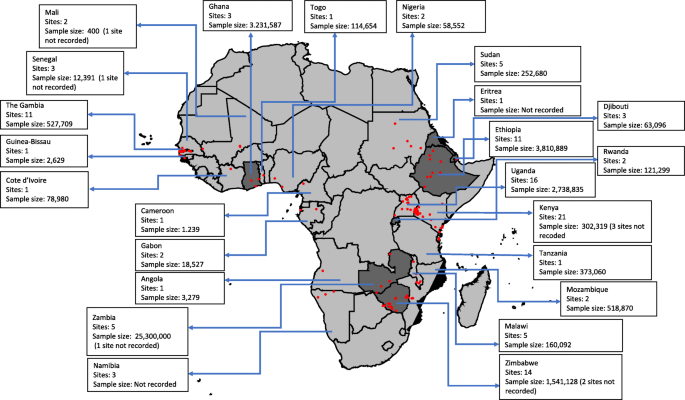
Assembled data included in the review by country, the number of sites and the sample size; dark grey indicates countries that reported national routine malaria case data, and the red dots indicate unique sites where data was identified
In the subsequent comparator analyses of modelled predictions of changing disease burden, 31/124 study sites from 21 publications were not included. The reasons for exclusion included the following: (i) 16 study sites in Ethiopia, Namibia and Rwanda classified as having reliable routine data to estimate malaria burden by WHO since the comparison would not be relevant in countries where surveillance data already feeds into the WHO report; (ii) 13 study sites in Djibouti, Eritrea, Ghana, Sudan, Zambia, Zanzibar and Zimbabwe that reported malaria case at national or regional level, the wide spatial extents of these data with likely inherent large variations in disease risk, precluding comparisons with modelled estimates; and (iii) two study sites (Dielmo, Senegal and Bandiagara, Mali) that reported community-cohort study data which we considered methodologically different from passive case detection (Additional file 3 ).
The remaining 93 sites from 46 publications included 28 (30%) sites that reported 5 years temporal data, 33 (36%) sites reported temporal data between 6 and 9 years and 32 (34%) sites reported data between 10 and 15 years. Three sites (3%) reported data between 2000 and 2005, 32 (34%) sites reported data post-2005 and 58 (63%) sites reported data spanning the period pre- and post-2005. The average starting parasite prevalence in children aged 2–10 years was < 10% in 19 sites, 10–50% in 53 sites and > 50% in 19 sites (Table 3 ). A sample of graphical presentation of extracted data, display of trend lines and the rho values were computed (Additional file 4 ).
Among the 93 sites included in the comparative analysis, 33% (31/93) showed evidence of a rise during the surveillance interval, and 67% (62/93) showed evidence of a decline. The matched modelled estimates predicted a rise in 23 sites and a decline in 70 sites. In 59 (63.4%) sites, the trends in both the empirical data and the modelled prediction were similar. However, 21 sites showed a rise in the empirical data while the modelled predictions showed a decline, and in 13 sites, the empirical data showed a decline while the modelled predictions showed a rise (Gwet’s AC1 = 0.378). In addition, 47% of the studies showed a strong positive association ( rho ≥ 0.6), 15% (14/93) showed a moderate positive association (0.2 < rho < 0.6), and 38% (35/93) showed a weak association ( rho ≤ 0.2) (Additional file 5 ). Eight sites, four in Kenya [ 19 , 35 ], one in Mozambique [ 36 ], two in Uganda [ 28 , 32 ] and one in Zimbabwe [ 37 ], showed strong negative correlations ( rho ≤ − 0.6) (Additional file 5 ) indicating that the trends in the observed data versus the predicted data were markedly different. Four of the eight sites were in low malaria transmission areas and were classified as having a moderate risk of bias score. When we assessed for outliers using the leave-one-out method, there was no single study that was a substantial cause of heterogeneity (Additional file 6 ). Further, there was no evidence of publication bias (Egger’s test: p = 0.73 and Additional file 7 ). The summary meta-analysis of these studies showed a moderate positive association between empirically recorded changes in clinical incidence/test positivity rate and MAP modelled predictions of clinical malaria incidence ( rho = 0.51; 95% CI 0.37, 0.63; p < 0.001; unadjusted I 2 = 69.63%), indicating substantial heterogeneity.
When we explored possible sources of the observed heterogeneity by univariate analysis using meta-regression, we found that the slope of empirically recorded clinical incidence/test positivity rate (i.e. the rate of the empirically observed decline) was the single most important covariate, accounting for a 16% change in I 2 ( rho = − 0.989; 95% CI − 0.998, − 0.939; p < 0.001; residual I 2 = 58.8%) (Table 3 ). The quality of data extracted, geographical region, reported measure of malaria, source of data, average starting parasite prevalence in children aged 2–10 years and slope of modelled clinical malaria incidence were additional sources of heterogeneity. However, the percentage change in I 2 from these factors was minimal (which were responsible for percentage changes from 2% up to 9%, Table 3 ). Sample size and sum of residuals did not seem to explain the heterogeneity observed (Table 3 ).
The slope of clinical incidence/test positivity rate remained robust as a predictor of correlation even after excluding studies with a high risk of bias ( rho = − 0.989; 95% CI − 0.998, − 0.923; p < 0.001) and after adjusting for the other study-level characteristics ( rho = − 0.967; 95% CI − 0.997, − 0.689; p = 0.005; Additional file 8 ). As a sensitivity analysis, we examined stratifying the slope of empirically observed data as showing a decrease (< 0) or increase (≥ 0) and also found a significant association in the mean difference of the correlation between the observed data and the modelled prediction ( rho = − 0.75; 95% CI − 0.86, − 0.60; p < 0.001; Fig. 3 ). We also examined the effect of the method of parasitological diagnosis and found a significant difference in the correlation between the observed data and modelled prediction when we stratified the analysis by the method of diagnosis (Additional file 9 ).

Forest plot of the correlation between empirical malaria cases and MAP clinical incidence stratified by the slope of empirical cases. Blue squares represent the correlation of each study; the error bars through the blue boxes are the uncertainty intervals; the red diamonds show the overall pooled correlation and in each sub-group; the horizontal tips of the red diamonds are the uncertainty level; weights are computed as the inverse of within and between variance; references are listed alphabetically in the Additional file material
The demand for annual progress assessments of key malaria indicators has fueled the use of advanced mathematical models in order to estimate and predict malaria disease burdens and trends [ 1 , 8 , 38 ]. The application of these models has enabled the production of predictive maps of malaria risk [ 1 , 8 ]. This review assesses whether trends in malaria cases from empiric surveillance are in agreement with the predictive model of malaria burden in SSA developed by MAP. We used empirically reported malaria data obtained from 93 health facility-based study sites from 46 published articles.
The empirical data obtain in this review included sites across the spectrum of malaria transmission intensity. The majority (60%) of sites were in moderate malaria transmission areas, 20% in low transmission areas and 20% in high transmission areas. There are previous reviews on the trends of malaria burden in Africa [ 12 , 13 , 14 ]. Our updated review is the first to make a comparison between empirical observations and modelled predictions.
Forty-seven percent of the study sites showed a strong positive association between empirical data and modelled predictions, while 38% of the studies showed a weak association. The single most important factor explaining this variation was the slope of empirically observed data over time (i.e. steep declines were associated with stronger correlations with the predicted estimates). If empirically recorded changes in malaria burden in a specific geographical area were on a downward trend, then the MAP modelled prediction was in agreement with this change (Fig. 3 ). Conversely, MAP modelled predictions showed a weak association with empiric surveillance data in sites that showed a stalling in progress or resurgence in empirically reported malaria burden. Although quality of study seemed to explain some of the variation, it was not a major cause of the heterogeneity observed when tested using meta-regression. One might expect studies classified as having a high risk of bias to correlate poorly with the modelled predictions, but this was not observed in our analysis (Table 3 ).
Although surveillance data on malaria cases recorded by health facilities were used, such data are not yet considered sufficiently reliable to track change in endemic countries in Africa [ 3 ]. This stems from varying clinical definitions of malaria between sites, changing case definition, changes in healthcare access and treatment-seeking behaviour, changes in diagnostic practice and reporting procedures or completeness within health systems which could have potentially affected the trends of malaria burden observed [ 3 ]. As a sensitivity analysis, we examined the effect of the method of diagnosis on the overall correlation. We found that the overall correlation among studies with parasitological confirmation was higher compared to studies with unclear methods of malaria diagnosis (Additional file 9 ). Nevertheless, even among those with documented parasitological confirmation, 41% (29/70) of the sites had rho < 0.5. While not all parasitologically diagnosed fevers are attributable to malaria [ 39 ], both coincidental infection and attributable fever may act as a valuable guide of malaria transmission. We could not evaluate the role of other factors on the impact of observed trends as most of the articles did not report on all the required information. However, our review was limited to data published in peer review journals with the assumption that data quality was examined during the peer review process.
Empirical reporting of malaria indicators should be a vital component of national control programs and now forms a central pillar of the Global Technical Strategy for malaria [ 40 ]. Improvements in routine data capture platforms [ 41 ], fever parasitological testing rates [ 42 ] and geospatial techniques to interpreting routine data [ 43 , 44 ] have improved data availability in Africa [ 3 ]. In our review, assembled data from the published literature was patchy and was disproportionately from sites with research investment. Despite two decades of substantive investment in malaria control in Africa, we were able to identify only 67 published studies on the changing burden of malaria over this period. In many countries, routine data remain imperfect and are often unpublished, and hence, modelling will still be required unless further investments are made in surveillance capacity. Models are valuable in making predictions and generating hypotheses, and it is essential that commensurate investments are made in surveillance data to support and test these predictions. Another option is to develop sentinel hospitals to validate and use alongside current models of disease burden. While there are factors that might impact on the definition of a malaria case, temporal trends in hospitalized malaria cases may serve as a valuable barometer of community trends in disease burden and may have value more broadly than just in malaria [ 45 ].
This review had some limitations. Some articles did not report on all the required information. For example, only 31% of the articles included in this review had a low risk of bias due to the inherent methodological insufficiencies in the published articles. However, we acknowledge that the critical appraisal tool used in this review was based on a scale in which various components of the quality were scored and it is possible that it included fewer items that were critical in maintaining internal validity. In addition, some studies had 5 years’ worth of data which might have led to large uncertainty in the correlation. Secondly, there is limited published literature on trends of malaria risk in certain regions, particularly in central Africa. The studies reported in this review represent just 24 of the 45 countries reported to be endemic for malaria in WHO African region. Thirdly, although there was no evidence of publication bias, we must bear in mind the limitation of using published literature to validate the modelled prediction. For instance, bias might be introduced if publication of data that suggest malaria burden is worsening to encourage further investment in malaria control or data that reflects a decline to justify investment are favoured. More importantly, the use of incidence or number of cases could have led to over- or underestimation of malaria burden since passive surveillance can rarely be conducted with certainty regarding the population accessing care at any specific facility. Hence, variations in the population access to a local facility, behavioural changes or policy changes may lead to artefactual trends in malaria in the absence of actual variation in transmission.
In many locations, both local surveillance data and modelled estimates showed declines in malaria burden and hence similar trends. However, there was spatial heterogeneity and some areas of Africa may have stable case numbers or upward trends. In these areas, there was a weak association between individual surveillance datasets and the modelled predictions. At broad regional scales, models may offer some guide on the overall trends in disease burden but have reduced predictive accuracy at local scales. The paucity of high quality, temporal clinical data in Africa must be redressed, to avoid a continued dependence on models or to help train future models. A public health priority should be high quality and temporally and spatially dense clinical data in Africa to empower national malaria control programs and to strengthen both empiric reporting and modelled predictions.
Availability of data and materials
A geo-coded repository of the data used for analysis is provided in Harvard Dataverse.
Abbreviations
- Malaria Atlas Project
- Plasmodium falciparum
International Prospective Register of Systematic Reviews
- Correlation
Sub-Saharan Africa
Parasite rate
World Health Organization
Preferred Reporting Items for Systematic Reviews and Meta-Analyses
Bhatt S, Weiss DJ, Cameron E, et al. The effect of malaria control on Plasmodium falciparum in Africa between 2000 and 2015. Nature. 2015;526(7572):207–11.
Article CAS PubMed PubMed Central Google Scholar
Snow RW, Sartorius B, Kyalo D, Maina J, Amratia P, Mundia CW, et al. The prevalence of Plasmodium falciparum in sub-Saharan Africa since 1900. Nature. 2017;550(7677):515-8.
WHO. World malaria report. Geneva: World Health Organization; 2019. https://www.who.int/malaria/publications/world-malaria-report-2018/en/ . Accessed 06 Jan 2020.
Google Scholar
Snow RW. Sixty years trying to define the malaria burden in Africa: have we made any progress? BMC Med. 2014;12:227.
Article PubMed PubMed Central Google Scholar
White NJ, Dondorp AM, Faiz A, Mishra S, Hien TT. New global estimates of malaria deaths. Lancet. 2012;380(9841):559–60.
Article CAS PubMed Google Scholar
WHO. Meeting report of the WHO Evidence Review Group on malaria burden estimation methods. Geneva: World Health Organization; 2018. https://www.who.int/malaria/meetings/2018/quantifying-morbidity-mortality/en/ . Accessed 25 Mar 2019.
Cameron E, Battle KE, Bhatt S, et al. Defining the relationship between infection prevalence and clinical incidence of Plasmodium falciparum malaria. Nat Commun. 2015;6:8170.
Article PubMed Google Scholar
Gething PW, Casey DC, Weiss DJ, et al. Mapping Plasmodium falciparum mortality in Africa between 1990 and 2015. N Engl J Med. 2016;375(25):2435–45.
Hay SI, Smith DL, Snow RW. Measuring malaria endemicity from intense to interrupted transmission. Lancet Infect Dis. 2008;8(6):369–78.
WHO. World malaria report. Geneva: World Health Organization; 2017. http://www.who.int/malaria/publications/world-malaria-report-2017/en/ . Accessed 3 Aug 2018.
WHO. World malaria report. Geneva: World Health Organization; 2018. https://www.who.int/malaria/publications/world-malaria-report-2018/en/ . Accessed 22 Jan 2019.
Gething PW, Battle KE, Bhatt S, et al. Declining malaria in Africa: improving the measurement of progress. Malar J. 2014;13:39.
Nkumama IN, O’Meara WP, Osier FHA. Changes in malaria epidemiology in Africa and new challenges for elimination. Trends Parasitol. 2017;33(2):128–40.
O’Meara WP, Mangeni JN, Steketee R, Greenwood B. Changes in the burden of malaria in sub-Saharan Africa. Lancet Infect Dis. 2010;10(8):545–55.
Moher D, Liberati A, Tetzlaff J, Altman DG, Group P. Preferred reporting items for systematic reviews and meta-analyses: the PRISMA statement. Open Med. 2009;3(3):e123–30.
PubMed PubMed Central Google Scholar
Gwet KL. Computing inter-rater reliability and its variance in the presence of high agreement. Br J Math Stat Psychol. 2008;61(Pt 1):29–48.
Munn Z, Moola S, Riitano D, Lisy K. The development of a critical appraisal tool for use in systematic reviews addressing questions of prevalence. Int J Health Policy Manag. 2014;3(3):123–8.
Ouma PO, Maina J, Thuranira PN, et al. Access to emergency hospital care provided by the public sector in sub-Saharan Africa in 2015: a geocoded inventory and spatial analysis. Lancet Glob Health. 2018;6(3):e342–e50.
Okiro EA, Alegana VA, Noor AM, Mutheu JJ, Juma E, Snow RW. Malaria paediatric hospitalization between 1999 and 2008 across Kenya. BMC Med. 2009;7:75.
Noor AM, Alegana VA, Gething PW, Snow RW. A spatial national health facility database for public health sector planning in Kenya in 2008. Int J Health Geogr. 2009;8:13.
Egger M, Davey Smith G, Schneider M, Minder C. Bias in meta-analysis detected by a simple, graphical test. BMJ. 1997;315(7109):629–34.
Thompson SG, Sharp SJ. Explaining heterogeneity in meta-analysis: a comparison of methods. Stat Med. 1999;18(20):2693–708.
Hamel MJ, Adazu K, Obor D, et al. A reversal in reductions of child mortality in western Kenya, 2003-2009. Am J Trop Med Hygiene. 2011;85(4):597–605.
Article Google Scholar
Larru B, Molyneux E, Ter Kuile FO, Taylor T, Molyneux M, Terlouw DJ. Malaria in infants below six months of age: retrospective surveillance of hospital admission records in Blantyre, Malawi. Malar J. 2009;8:310.
Ollivier L, Nevin RL, Darar HY, et al. Malaria in the Republic of Djibouti, 1998-2009. Am J Trop Med Hygiene. 2011;85(3):554–9.
Wragge SE, Toure D, Coetzee M, et al. Malaria control at a gold mine in Sadiola District, Mali, and impact on transmission over 10 years. Trans R Soc Trop Med Hyg. 2015;109(12):755–62.
Ogwang R, Akena G, Yeka A, Osier F, Idro R. The 2015-2016 malaria epidemic in Northern Uganda; what are the implications for malaria control interventions? Acta Trop. 2018;188:27–33.
Raouf S, Mpimbaza A, Kigozi R, et al. Resurgence of malaria following discontinuation of indoor residual spraying of insecticide in an area of Uganda with previously high-transmission intensity. Clin Infect Dis. 2017;65(3):453–60.
Tukei BB, Beke A, Lamadrid-Figueroa H. Assessing the effect of indoor residual spraying (IRS) on malaria morbidity in Northern Uganda: a before and after study. Malar J. 2017;16(1):4.
Chaves LF, Hashizume M, Satake A, Minakawa N. Regime shifts and heterogeneous trends in malaria time series from Western Kenya Highlands. Parasitology. 2012;139(1):14–25.
Okiro EA, Alegana VA, Noor AM, Snow RW. Changing malaria intervention coverage, transmission and hospitalization in Kenya. Malar J. 2010;9:285.
Okiro EA, Bitira D, Mbabazi G, et al. Increasing malaria hospital admissions in Uganda between 1999 and 2009. BMC Med. 2011;9:37.
Okiro EA, Kazembe LN, Kabaria CW, et al. Childhood malaria admission rates to four hospitals in Malawi between 2000 and 2010. PLoS One. 2013;8(4):e62214.
Stern DI, Gething PW, Kabaria CW, et al. Temperature and malaria trends in highland East Africa. PLoS One. 2011;6(9):e24524.
Kapesa A, Kweka EJ, Atieli H, et al. Why some sites are responding better to anti-malarial interventions? A case study from western Kenya. Malar J. 2017;16(1):498.
Ferrao JL, Mendes JM, Painho M, Joao SZ. Spatio-temporal variation and socio-demographic characters of malaria in Chimoio municipality, Mozambique. Mala J. 2016;15:329.
Muchena G, Dube B, Chikodzore R, Pasipamire J, Murugasampillay S, Mberikunashe J. A review of progress towards sub-national malaria elimination in Matabeleland South Province, Zimbabwe (2011-2015): a qualitative study. Malar J. 2018;17(1):146.
Murray CJ, Rosenfeld LC, Lim SS, et al. Global malaria mortality between 1980 and 2010: a systematic analysis. Lancet. 2012;379(9814):413–31.
Dalrymple U, Cameron E, Bhatt S, Weiss DJ, Gupta S, Gething PW. Quantifying the contribution of Plasmodium falciparum malaria to febrile illness amongst African children. Elife. 2017;6:e29198.
WHO. Global technical strategy for malaria 2016-2030. Geneva: World Health Organization; 2016. https://www.who.int/malaria/areas/global_technical_strategy/en/ . Accessed 06 May 2019.
Braa J, Sahay S. The DHIS2 open source software platform: evolution over time and space. In: Celi LAG, Fraser HSF, NikoreV, Osorio JS, Paik K, editors. Global Health Informatics. Principles of eHealth and mHealth to Improve Quality of Care. Cambridge: The MIT Press.
WHO. T3: Test. Treat. Track. Scaling up diagnostic testing, treatment and surveillance for malaria. Geneva: World Health Organization; 2012. http://www.who.int/malaria/publications/atoz/test_treat_track_brochure.pdf?ua=1 . Accessed 28 Mar 2017.
Alegana VA, Atkinson PM, Wright JA, et al. Estimation of malaria incidence in northern Namibia in 2009 using Bayesian conditional-autoregressive spatial-temporal models. Spat Spatiotemporal Epidemiol. 2013;7:25–36.
Bennett A, Yukich J, Miller JM, et al. A methodological framework for the improved use of routine health system data to evaluate national malaria control programs: evidence from Zambia. Popul Health Metrics. 2014;12(1):30.
English M, Mwaniki P, Julius T, et al. Hospital mortality - a neglected but rich source of information supporting the transition to higher quality health systems in low and middle income countries. BMC Med. 2018;16(1):32.
Download references
Acknowledgements
This work was supported through support to RWS as part of his Wellcome Trust Principal Fellowship (103602 and 212176) and DELTAS Africa Initiative [DEL-15-003]. EAO is funded through a Wellcome Trust Intermediate Fellowship (201866). The DELTAS Africa Initiative is an independent funding scheme of the African Academy of Sciences (AAS)’s Alliance for Accelerating Excellence in Science in Africa and supported by the New Partnership for Africa’s Development Planning and Coordinating Agency with funding from the Wellcome Trust [107769] and the UK government. All authors are grateful to the support of the Wellcome Trust to the Kenya Major Overseas Programme (203077).
Author information
Authors and affiliations.
KEMRI-Wellcome Trust Research Programme, Kilifi, Kenya
Alice Kamau, Polycarp Mogeni, Emelda A. Okiro, Robert W. Snow & Philip Bejon
Centre for Tropical Medicine and Global Health, Nuffield Department of Clinical Medicine, University of Oxford, Oxford, UK
Alice Kamau, Robert W. Snow & Philip Bejon
You can also search for this author in PubMed Google Scholar
Contributions
RWS, PB and AK conceived the idea of the study and together developed the protocol. AK and PM did the literature search, selected the studies and extracted the relevant information. AK and RWS synthesized the data. Data were analyzed by AK with input from PB and RWS. EAO provided additional data. AK, PB and RWS wrote the first draft of the paper. AK, PB, RWS, PM and EAO critically revised the successive drafts of the paper and approved the final version.
Corresponding author
Correspondence to Alice Kamau .
Ethics declarations
Ethics approval and consent to participate.
Not applicable
Consent for publication
Competing interests.
The authors declare no competing interests.
Additional information
Publisher’s note.
Springer Nature remains neutral with regard to jurisdictional claims in published maps and institutional affiliations.
Supplementary information
Additional file 1..
Assessment of the quality of all studies included in the review based on Joanna Briggs Institute Prevalence Critical Appraisal Tool: Quality assessment tool for prevalence studies.
Additional file 2.
Schematic outline of the steps taken in the data analysis.
Additional file 3.
Summary characteristics of included studies, the first 31 study sites were not included in the meta-analysis for reasons outlined in the main manuscript.
Additional file 4.
The graphical presentation of extracted data, display of trend lines and the rho values of the published studies and MAP model.
Additional file 5.
Forest plot of ordered correlations between empirically recorded clinical incidence/test positivity rate of P. falciparum and MAP modelled predictions of clinical malaria disease incidence.
Additional file 6.
Plots used to visualize the leave-one-out estimates, to identify outliers, or influential studies. We used a built-in function (Baujat Plot) using the package metafor in R statistical software where the outlying effect sizes were identified.
Additional file 7.
Funnel plot with pseudo 95% confidence limits showing the Fisher’s Z transformed correlation coefficient (arctanh(r)) against the standard errors of arctanh(r).
Additional file 8.
Sources of heterogeneity assessment based on multivariable meta-regression analyses.
Additional file 9.
Forest plot of ordered correlation between empirically recorded clinical incidence/test positivity rate of P. falciparum and MAP modelled predictions of clinical malaria disease incidence stratified by method of diagnosis.
Rights and permissions
Open Access This article is licensed under a Creative Commons Attribution 4.0 International License, which permits use, sharing, adaptation, distribution and reproduction in any medium or format, as long as you give appropriate credit to the original author(s) and the source, provide a link to the Creative Commons licence, and indicate if changes were made. The images or other third party material in this article are included in the article's Creative Commons licence, unless indicated otherwise in a credit line to the material. If material is not included in the article's Creative Commons licence and your intended use is not permitted by statutory regulation or exceeds the permitted use, you will need to obtain permission directly from the copyright holder. To view a copy of this licence, visit http://creativecommons.org/licenses/by/4.0/ . The Creative Commons Public Domain Dedication waiver ( http://creativecommons.org/publicdomain/zero/1.0/ ) applies to the data made available in this article, unless otherwise stated in a credit line to the data.
Reprints and permissions
About this article
Cite this article.
Kamau, A., Mogeni, P., Okiro, E.A. et al. A systematic review of changing malaria disease burden in sub-Saharan Africa since 2000: comparing model predictions and empirical observations. BMC Med 18 , 94 (2020). https://doi.org/10.1186/s12916-020-01559-0
Download citation
Received : 07 January 2020
Accepted : 16 March 2020
Published : 29 April 2020
DOI : https://doi.org/10.1186/s12916-020-01559-0
Share this article
Anyone you share the following link with will be able to read this content:
Sorry, a shareable link is not currently available for this article.
Provided by the Springer Nature SharedIt content-sharing initiative
- Systematic review
BMC Medicine
ISSN: 1741-7015
- Submission enquiries: [email protected]
- General enquiries: [email protected]
- Research article
- Open access
- Published: 23 April 2018
Malaria infection among children under-five: the use of large-scale interventions in Ghana
- Clifford Afoakwah 1 ,
- Xin Deng 1 &
- Ilke Onur 1
BMC Public Health volume 18 , Article number: 536 ( 2018 ) Cite this article
21k Accesses
30 Citations
1 Altmetric
Metrics details
Despite the significant investments to control malaria infection rates over the past years, infection rates remain significant in sub-Saharan Africa. This study investigates the association with use of large-scale malaria interventions such as: Indoor Residual Spraying (IRS), Insecticide Treated bed-Nets (ITN), and Behaviour Change Communication (BCC) strategies, and the prevalence of malaria among children under-five in Ghana.
Cross-sectional data on 2, 449 children aged 6 to 59 months who were tested for malaria, through Rapid Diagnostic Test (RDT), are drawn from the recent wave of the Ghana Demographic and Health Surveys (GDHS 2014). We use a logit model to analyse the heterogeneous association between control measures and malaria infection among under five children of different age cohorts and household poverty statuses.
Our estimates suggest that IRS offers much more protection than ITN use. The odds of malaria infection among children who sleep in IRS is significantly lower (odds ratio [OR] = 0.312; 95% CI -1.47 -0.81; p = 0.00) compared to those who are not protected. This association is even high (odds ratio [OR] = 0.372; 95% CI -1.76 -1.02; p = 0.00) among children in poor households protected by IRS compared to those who have no IRS protection. ITN use did not have a significant association with malaria infection among children, except among children whose mothers have at least secondary education. For such children, the odds of malaria infection are significantly lower ([OR] =0.545; 95% CI = − 0.84 -0.11; p = 0.011) compared to those who are not protected. Regarding BCC strategies, we found that malaria education through television is the best strategy to covey malaria education as it significantly reduces the odds of malaria infection ([OR] =0.715; 95% CI = − 0.55 -0.10; p = 0.005) compared to those who do not received malaria education via television. BCC strategy via print media has a significant but limited protection for children of educated mothers.
Policy makers should direct more resources to IRS, especially in communities where the use of ITN is less likely to be effective, such as poor and rural households. The distribution of ITNs needs to be accompanied with education programs to ensure its best protection.
Peer Review reports
Malaria is a life-threatening disease caused by infection through the bite of a female anopheles mosquito and poses the biggest health threat to children under five and pregnant women in malaria-prone areas such as sub-Saharan Africa. It is considered an endemic disease and a public health problem in Ghana due to the significant death toll associated with it [ 1 ]. It is also leads to poverty and low productivity due to human development, as well as due to its financial burden on households and the economy [ 2 , 3 ]. In Ghana it is estimated that every malaria episode recorded corresponds to an average of 5 workdays lost; 3 days to the patient and 2 days to the caretaker [ 4 ]. Figures from the World Health Organisation (WHO) [ 5 ] also suggest that malaria alone accounts for about 4 sick days in a month, and 6.4% of income loss in Ghana. Concerted efforts by governments, international organisations and charities to combat the disease have resulted in a sharp decline in global malaria morbidity and mortality by 37 and 60% respectively between 2003 and 2015 [ 6 ]. However, nearly half of the world’s population, or 3.2 billion people, are still vulnerable to this disease. Sub-Saharan African regions, in particular, carry a disproportionately high share of the global malaria burden. In 2015, the region experienced approximately 88% of global malaria infections and 90% of malaria deaths [ 6 ].
Over the past decades, there has been a significant increase in investment towards the fight against malaria. WHO reports an increase in resources for malaria control from US$ 960 million in 2005 to US$ 2.5 billion in 2014 globally [ 6 ]. In Ghana, as part of an eight-year malaria strategic plan to bring down the incidence of malaria by 2015, the government pledged to contribute US$ 231 million in 2008. Donors, including The United States Agency for International Development (USAID), Presidents Malaria Initiative (PMI), The World Bank, United Nations Children’s Fund (UNICEF) and the private sector, all financially support malaria control programmes in Ghana [ 7 ]. PMI is an inter-agency initiative led by USAID. Launched in 2005, PMI has a task of reducing malaria-related mortality by 50% across 15 high-burden countries in sub-Saharan Africa including Ghana.
Unfortunately, the rising investment to control the disease does not lead to a significant fall in infection among young children. Contrary to the global trend, malaria infection rates among children under-five in Ghana have been rising despite the efforts and investments. As shown in Table 1 , the number of reported cases of malaria among children under-five has been rising since 2000. Indeed, malaria accounts for more than half of Out Patient Department (OPD) cases of children under-five between 2010 and 2012, a sharp rise from 36.57% in 2000.
Although in 2016 there was a significant reduction in the number of deaths attributable to malaria (1037 in 2015 to 590 in 2016) as well as the decline in Case Fatality Rate (CFR) (0.51 in 2015 to 0.32 in 2016) among children under-five, the proportion of cases attributable to malaria for under-five children remains high at 46.7% [ 8 ].
According to the Ghana National Malaria Control Programme (NMCP), malaria kills at least 3 children every day and also tops OPD cases in Ghana. While it is encouraging to observe a decline in in-patient deaths emanating from malaria infection among children under-five, the statistics on morbidity and in-patient cases remain alarming. This, therefore, raises questions about the role of malaria interventions and the type of effective strategies among children under-five in Ghana. In particular, the challenge is identifying the factors which play an important role in improving the protection offered by the malaria control tools. Utilising a sample of 2449 children in Ghana, we explore the impact of most popular intervention tools on malaria infection among children under-five.
We use an objective malaria infection measure that was not previously available in Ghana. In 2014, for the first time, children under-five were tested for malaria using a RDT in the GDHS. This objective measure makes it possible to test the association between IRS, ITN use and malaria infection in a large scale.
The rest of the paper is organized as follows. Section 2 gives an overview of malaria control tools in Ghana alongside with the literature review. The methodology and data are described in Section 3. Our main results are presented and discussed in Section 4, followed by robustness analysis in Section 5. The last section concludes with policy recommendations.
Malaria control tools and the Ghanaian context
IRS and ITNs are the two main interventions recommended by the Global Malaria Programme in the WHO for malaria control. IRS is regarded as one of the most efficient vector control measures for tackling malaria transmission. It involves the spraying of walls and roofs of houses with long-acting chemical insecticides. This helps to kill the adult mosquitoes that dwell on such surfaces [ 9 ]. The role of IRS in reducing malaria infection is well-established with ample scientific evidence [ 10 , 11 ]. Similarly, an empirical study on Ghana by Fuseini et al. [ 12 ] provide evidence on the efficacy of IRS.
In Ghana, IRS is carried out by the government, private individuals, and non-governmental organisations such as Anglogold Malaria (AGAMal), Global Fund and PMI. The use of IRS has been shown to have economic benefits in Ghana. For example, work absenteeism due to malaria infection has dropped from 6983 man-days in 2005 to 163 in 2010 due to the use of IRS [ 7 ]. However, due to the high cost of new insecticides for spraying, IRS exercise has reduced overtime. The PMI on IRS, for example, has scaled down its exercise from 9 to 4 districts in 2013 [ 7 ].
Similar to IRS, ITNs work as a vector control intervention to reduce malaria and other infections transmitted by insects [ 13 , 14 , 15 ]. Use of ITNs refers to hanging treated nets over one’s sleeping area to prevent mosquito bites [ 16 ]. Ghanaian government started to distribute ITNs to targeted groups through a multi prolonged distribution system in 1998. Beginning 2009, the Universal Coverage strategy was adopted and it aimed at providing one net to every two persons in a household through door-to-door distribution [ 7 ]. Distribution of bed-nets is also targeted at school pupils in order to increase its coverage. Pregnant women are also given ITNs on antenatal visits and all other individuals have the option of buying ITNs from shops/markets, pharmacy stores, and street vendors, among others [ 1 ]. Afoakwah et al. [ 17 ] have also shown that the use of ITNs can reduce under-five mortality by about 18.8% in Northern Ghana. Information on the direct impact of ITN use on malaria infection of the young children is however rare.
In addition to IRS and ITN use, BCC is also shown to be an important strategy through which malaria education is conveyed to communities, especially in malaria prone areas. In Ghana, malaria education in the form of prevention and treatments are conveyed through media sources including the television, radio, newspaper and magazines, posters, leaflets and brochures, and health workers and community volunteers [ 1 ]. The Ministry of Health (MoH) and the NMCP have adopted this approach to disseminate malaria information to Ghanaians in order to increase their knowledge and awareness, and to promote positive behavioural changes towards malaria prevention. Rhee et al. [ 18 ], for example, found that better knowledge levels about malaria significantly increased ITN use among households in Mali. Their controlled trial revealed that ITN use was greater among participants who had received malaria education compared to participants who did not receive any education. Ayi et al. [ 19 ] also found that malaria education improved school children’s perception about malaria in Ghana, and led to an increase in the proportion of adults using treated bed-nets. However, the effect of such efforts on malaria infection among children under-five in Ghana is still unclear. To this end, we include BCC strategies such as television, radio and print media (newspaper) in addition to IRS and ITN to evaluate their association with malaria infection rates among children under-five.
Building on the current literature, this study uses a logit model to estimate the likelihood that a child gets infected with malaria. The model considers child’s attributes and household effects as well as observed malaria control measures including ITN, IRS and BCC. The aim is to control for covariates that theoretically and intuitively impact the child’s propensity to being infected. The corresponding logit model is specified as:
where M ∗ is a latent variable that is unobserved. M is a dummy variable that takes the value of 1 if the test outcome is positive and 0 if negative; I is a vector of variables for individual child attributes such as age, weight and gender. E captures environmental factors such as parental education, rural dwelling, household size and household wealth. MP captures malaria preventive measures, ITN use, IRS, and BCC. u is the error term.
IRS is a dummy variable with the value 1 if the child slept in a room that has been sprayed in the last 6 months. ITN, as captured by the GDHS report, includes factory-treated net that does not need further treatment, or a pre-treated net obtained within the past twelve months, or a soaked net with insecticides within the past twelve months. There are two indicators of ITN use in the data set: mother’s use of ITN and child’s use of ITN. It is common practice in Africa that mothers sleep with their young children [ 20 ]. Therefore the mother and child’s use of ITN is a better measure of the use of ITN as a preventive tool for young children. The data indicated that malaria infection is higher among children who slept under ITN alone than those who did not (49.77% vs 36.89%). A possible explanation is that children are more likely to be put under ITN after they show symptoms of fever or tested positive for malaria. In other words, ITN is used as a treatment method instead of a preventive tool. This could also be due to social desirability on the part of the parents in reporting use of ITNs by their children. Given the fact that it is popular in Ghana for mothers to sleep with their young children, we consider ITN use only when both mother and child use it. Footnote 1
Regarding behavioural change communication, we include media source of malaria education such as audio (radio), audio-visual (television), and print media (newspaper), mainly due to their high utilisation rate among the population. Other sources were not included because this was not a mutually exclusive response, possibly causing multicollinearity. In estimating Eq. ( 1 ), the Maximum Likelihood Estimation (MLE) technique (specifically a logit model that has a flatter tail compared to a probit model) is employed. The logit model is appropriate as the study seeks to provide information on the odds of being infected with malaria.
The study uses secondary data sourced from the current round of the Ghana Demography and Health Survey [ 1 ]. This survey is conducted every five years since 1988, with 2014 being the most recent wave, and it is collected from rural and urban areas in all ten regions in Ghana. These data sets are collected by the Ghana Statistical Service (GSS) and the Ghana Health Service (GHS) with support from the National Public Health Reference Laboratory (NPHRL) of the GHS. During the 2014 survey, field health assistants collected capillary blood samples from children aged 6 to 59 months in half of the households surveyed. The health assistants then tested for malaria on the field using a RDT with consent from the parent or the guardian. The outcome of the test was then recorded as either positive or negative. Children with positive results are considered as having malaria infection. About 2698 children had their blood samples tested, which represents 97% of all eligible children [ 1 ]. Demographic information is also collected via parental self-reports.
The sample used for this study comprises 2449 children aged between 6 and 59 months in Ghana. This represents 90.77% of the total children who were involved in the malaria test exercise. The remaining 9.23% were excluded due to missing information on some of the covariates used for the regression analysis. Descriptive statistics are presented in Table 2 . 40.50% of children have tested positive. The average age is 32 months and 52% are boys, with an average weight around 12 kg. With regards to household attributes, average family size was 6 persons and 60.20% lived in rural areas. A significant proportion (73.20%) lived in poor households. Household wealth index used in this study was constructed by the GSS using Principal Components Analysis (PCA). Items such as television, bicycle or car, as well as dwelling characteristics, such as a source of drinking water, sanitation facilities and type of flooring material are used to compute this index (see [ 1 ] for further details). It is noteworthy that 57.10% of mothers have no secondary education and 32.40% of mothers have no formal education at all. Fathers have significantly higher levels of education compared to mothers.
38.2% of children in our sample were not protected by either ITN or IRS, and 40.20% of children and their mothers slept under ITN the night before the survey and only 13.20% of children slept in a room that has been sprayed. The remaining 8.40% were covered by both ITN and IRS. In terms of malaria education, it was found that most of these households (78.4%) receive malaria education via radio, followed by television (52%) and newspaper (9.10%).
Table 3 presents the prevalence of malaria among children under-five for the entire sample, and for various sub-groups. There is not much difference between boys and girls in terms of infection rate, but the prevalence of malaria among rural children (56.08%) is more than twice of that among urban children (21.71%). Maternal education level is found to be highly correlated with malaria infection among children. Children whose mother has at least secondary education are the least vulnerable groups while children whose mother has no education record the highest prevalence of malaria infection. Relative to ITN use, children who sleep in rooms with IRS are less vulnerable to malaria infection. Children whose carers receive malaria education through television have lower rates of malaria infection than those reporting to have received education through radio or print media (newspaper).
In order to observe how individual attributes, environmental factors and malaria preventive measures interact with risk of malaria infection, a step-wise regression is employed. The results are reported in Table 4 . The Chi-square statistics (a goodness-of-fit test) suggest that all four specifications present regression lines that are a good fit at 1 % significance level. In the first specification, the impact of individual characteristics of the children is tested. Age and weight are the only significant variables, and gender does not play any significant role in malaria infection. We then add the environmental factors and present our results in specification 2. We observe a decrease in the coefficient and the odds ratio for age, but an increase for weight. Moreover, most of the environmental variables are also statistically significant, except for the poorer households when compared the poorest ones, and mother’s primary education when compared to no education. Specification 3 then adds malaria preventive measures to specification 2, followed by addition of interaction variables in the last specification. In specifications 2 to 4, the coefficients and the odds of malaria infection are very similar for the age and weight variables. Weight also has a non-linear effect on the odds of malaria infection. Results for the weight and weight-squared variables suggest an increase in the odds of malaria infection among children weighing up to 11.5 kg but a reduction in the odds for those above this weight limit. The reflection point is computed using the coefficients for the weight and weight-squared variables in Table 4 . Similar to the child attributes, we observe little variation in the coefficient and the odds ratios for the environmental factors when specification 2 results are compared to the ones in specifications 3 and 4. As a result, we focus our discussion on the results for the individual, environmental, and malaria control variables from specifications 3 and 4.
Individual attributes
A child’s odds of being infected by malaria are positively associated with his/her age. This relationship may not be linear and the non-linearity may be explained by weight and weight-squared. Given that the turning point for weight (11.5 kg) corresponds to the mean weight, it suggests that children become more resistant to diseases after reaching a certain weight. This could be an indicator of an improved immune system after 37 months of age as shown in Table 5 of the sensitivity analysis. Also at the age the child weights 11.5 kg, he/she may sleep with his/her mother under one ITN and would receive a better protection from the net. The gender dummy variable is not significant in all four regressions. This finding seems to be different from the experimental observation by Cernetich et al. [ 21 ] where they noticed that females had faster recovery from anaemia, induced weight loss and had reduced mortality.
Environmental factors
Due to the endemic nature of malaria in Ghana, we investigate the influence of the environment surrounding the children. We control for rural dwelling, household size, household wealth and parental education. Each of these contextual factors significantly influences the odds of malaria infection among children under-five. The prevalence of malaria in rural areas is reported to be about 38% compared to 15% for people who live in urban environments [ 1 ]. Consistent with the GDHS [ 1 ] our study shows that living in a rural area increases a child’s odds of being infected. The dichotomous rural dwelling variable is statistically significant in both specifications at 1 % level. Children in rural areas are about 75 to 80% more likely to be infected with malaria than their counterparts in urban settings. This could potentially be due to the low use of intervention tools among rural folks. For example, the GDHS [ 1 ] reports that while 64% of rural dwellers have access to ITN, only 47% use the net. Another reason is that most in rural areas are wetlands used for farming activities [ 22 ] which present breeding places for mosquitoes. This finding emphasizes the need for critical attention to children in rural areas.
With regards to parental education, we show that higher parental education is associated with lower odds of malaria infection among their children. Secondary education of parents has the highest significant effect on malaria prevention. Maternal education is also argued to play a significant role in child health outcomes through increased labour market earnings [ 23 ] and changes in individual behaviour through increased autonomy [ 24 ].
Similarly, children who live in large households are more likely to have malaria infection. Having one additional member in the household increases the likelihood of infection by about 6.5%. With a mean household size of 5.89 (Standard deviation 2.73), a plausible explanation is that the presence of congestion in homes may produce better breeding grounds for mosquitoes and less time and resources for preventive measures. In addition, a larger household means less space for sleeping and this makes it difficult to mount ITNs to protect all household members. It is common in rural Ghana that an area is used for living room in the day time and a bedroom at night. In such cases, the ITN needs to be mounted on the wall every night. This is a burden for the household and may discourage the use of an ITN. Justeson and Kunst [ 25 ] suggest that the risk of disease spread among groups of persons living together is significantly correlated with the level of nucleation that exists among them. Large households might also tend to be busy and early disease symptoms, especially in young children, can remain unnoticed, resulting in delays in treatment and therefore lead to more adverse effects [ 26 ].
We also show that malaria infection is highly correlated with household wealth. The odds of malaria infection among children aged below five years decreases as household wealth rises. Compared to the poorest households, children from richer households are about 77.8% less likely to be infected with malaria, and the odds are 86% lower for children in the richest households, all else being equal. This may be attributed to the fact that wealthier families can afford better goods and services leading to more positive health outcomes. Arthur [ 27 ] found a similar correlation between wealth and antenatal care in Ghana. It is therefore important to note that poorer households should be prioritized in any mass distribution of ITNs as well as IRS campaigns. This form of prioritisation is critical especially since the summary statistics suggest that 54.3% of these children live in poorer households.
Malaria control measures
As mentioned earlier, in Ghana ITNs have been freely distributed to households by public and private organisations since 1998 [ 7 ]. The GDHS report shows that about 85% of ITNs were obtained in this way. IRS is only implemented by government and non-governmental organisations in selected districts of Ghana depending on financial costs and technical feasibility [ 1 ]. Therefore, a significant proportion of households not covered by IRS had to rely on ITN as the main preventive measure.
Of the two main preventive measures, we find no significant association between ITN use and malaria infection among young children. Previous studies identified two main reasons that may undermine the protection offered by ITN use. First, the ITNs used may have lost their insecticidal protection, making the nets less efficient [ 28 ]. Second, some users may not lay out the net correctly due to the perceived heat increase and fear of suffocation that come with the use of ITNs [ 29 , 30 ].
IRS, on the other hand, is associated with reduced incidence of malaria infection. Children who sleep in rooms that have been sprayed in the last 6 months are about 68% less likely to be infected with malaria than children who do not, all else being equal. This finding supports similar evidence presented by Loha et al. [ 10 ] that IRS significantly reduces the incidence of falciparum malaria. The dual use of both ITN and IRS, however, does not provide an added protection.
Although ITN use alone is not significantly linked with a reduction of malaria infection, specification 4 suggests that ITNs used by educated mothers are associated with a lower incidence of malaria. We interact only mother’s education because they are the primary care giver of the child. For children whose mother has at least secondary education, use of ITN reduces their odds of malaria infection by 45.50%. Inasmuch as this finding gives credence to Gary Becker’s human capital theory, the implication here is that educated mothers are more likely to be aware of the benefits of using ITN and are capable of the appropriate use of the net to protect their children. Our finding is consistent with findings that in Botswana and Zimbabwe educated mothers were more successful in reducing the prevalence of diarrhoeal diseases among the children [ 31 ].
With regards to behavioural communication change measures, our study analyses how three forms of media – television, radio and newspaper impacts malaria infection among children. Although a significant percentage of households receive malaria education through the radio (78.4%), no significant association has been identified with radio exposure and malaria infection reduction. Malaria education via television is the only variable with an independent significant link with child’s malaria infection. On average, malaria education through television reduces the odds of infection by 26.9%, keeping all else constant.
While malaria education through print media did not show a significant independent effect, it was significant for children whose mother had at least secondary education. This is not surprising because it is more likely that educated parents can read, understand and communicate information conveyed through print media. These findings suggest that television and print media play a critical role in disseminating information on malaria but providers need to be aware of the audience. Given that nearly 60% of carers were educated at primary level or below, audio-visual (television) forms of malaria education appear to be the most effective method.
Our study has shown that the effect of the IRS is far more than of that for ITN use. We have also demonstrated the important role of mother’s education in facilitating the protection provided by malaria intervention tools, especially ITN use. Thus, our results echo the crucial need to educate the users before implementing an intervention that requires correct use. We also find that an association between mother’s exposure to print media and children’s infection rate, but only if the mother has completed at least secondary education. However, a more effective way of communicating behaviour change towards malaria control is the television.
Sensitivity analysis
It is probable for the effectiveness of the malaria preventive measures as well as some of the explanatory variables to differ depending on the age of the child or the wealth of the household. Thus, as a sensitivity analysis, we run the regressions for various age cohorts and household wealth groups. We run the regressions for both specifications 3 and 4, and report only the odds ratios. For comparison reasons, the main results for specification 3 are presented at the top panel, and the bottom section includes all the variables from specification 4.
For the age-cohort regressions, presented in Table 5 , we divide the sample into three; 6 to 24 months, 25 to 36 months, and 37 to 59 months. Focusing on the results for specification 3, we show that ITN does not have a significant effect on malaria infection in none of the age cohorts. Although the coefficient for the ITN variable is not significant, it has a negative effect on malaria among children up to two years old, which supports the assertion that younger children sleep with their mothers and are protected through proper use. However, IRS has a sizable effect on the odds ratios for all age cohorts. The results for using both ITN and IRS does not differ much compared to the only IRS odds ratios. Although the dual use of both ITN and IRS does not provide an added advantage to the youngest cohort, our results show that for children above 2 years of age, a combination of the two offers better protection.
Child weight has a notable effect on incidence of malaria infection depending on the age cohort. For young children and babies child weight increases the incidence of malaria infection, while we observe the opposite effect for older children aged 37 to 59 months. Weight gain among children aged 37 months and above corresponds to a decline in the odds of malaria infection. Living in a rural area and living in a more crowded household cause an increase in malaria infection rates. The only exception is for the effect of household size on older children; we find no statistical significance. Household wealth has the expected effect on malaria infection and the results are consistent among age cohorts. Richer and the richest households significantly lower the malaria infection incidence irrespective of the child’s age. An interesting finding is the effect of the education variables. More educated parents significantly lower the malaria infection incidence for only the oldest cohort.
Regarding the malaria prevention measures, the IRS variable has a significant effect in lowering malaria incidence, and this effect is slightly lower in specification 4 compared to specification 3 for all age cohorts. ITN and the mother’s education interaction terms are all insignificant except for the 2–3 year old children. Having a more educated mother significantly lowers the malaria incidence for these households. Finally, among the BCC strategies, we observe that television has a significant effect except for the oldest cohort, and the effectiveness of the newspaper depends on the mother’s education level.
To analyse our findings by varying household wealth, in Table 6 , we present the results after dividing the sample into three categories; poor, middle-class, and rich. An interesting observation from specification 3 results is that there is a statistically significant link between IRS and malaria infection of young children among poor and middle-class households, but such a link is absent among the rich households. Moreover, the effect of using both ITN and IRS is significant only for the poor households.
Similar to our previous findings, child weight has a positive effect on malaria incidence while the weight square has a negative effect. Both of these variables, however, are not significant for the rich households. Living in a rural area and in a crowded household again increases the odds of malaria infection except for the effect of household size on children in richer families. Parent’s education matters for the poor households and lowers the malaria incidence rates for children. However, a similar effect of parent’s education on malaria is missing for the middle-class and rich households.
Comparable to our finding in specification 3, the IRS is significant only for the poor and the middle-class households. However, the ITN variable interacted with mother’s education is only significant for the rich households. Similar to IRS, Television as a BCC strategy is only significant for the poor and the middle-class households. Another BCC strategy, newspaper, has a significant effect in lowering malaria incidence when interacted with mother’s education and this holds only for the poor households.
Overall, we find that our explanatory variables and malaria preventive measures have more of a significant effect on the poor households. The significance of our findings diminishes as the household wealth increases, with the least significant effects for the richer households. This is of great importance for the government while identifying the households to target their policies towards. Our findings also have crucial implications in terms of policy recommendations which we discuss extensively in the next section.
This paper explores factors influencing malaria infection among children under-five from three dimensions: individual attributes, living environment and intervention measures. Three conclusions can be drawn. First, we identify vulnerable groups among children under-five. Our results show that children living in a large family with low income in rural areas are most prone to malaria infection. In addition, our results suggest that children under age three are more prone to malaria infection. Therefore, children must be given critical attention during their early formative years until their immune systems have significantly improved. Second, this study has shown that the most effective tool to prevent malaria infection among children under-five is IRS and its protection far exceeds that noted for ITN use. Given that the number of young children protected by IRS is half that of those protected by ITN, policy makers should consider increasing the use of IRS. Such a policy change promises more significant positive results than trying to increase ITN use. This is because, unlike ITN, the effect of IRS is not moderated by any human capital attribute. In a developing country like Ghana, where educational levels are very low especially among women, intensifying IRS is the most efficient way of preventing malaria among children in poor and rural households. Third, mother’s education moderates the protection of ITN use and BCC strategy through print media. Mother’s education was found to be an important ingredient in the campaign to reduce malaria infections. Education enhances the role of ITN use and also strengthens BCC campaigns aiming at improving the efficacy of malaria preventive and curative measures.
Drawing on the findings of this study, we recommend that policy makers implement an intervention program tailored for various social and economic groups. First, prioritise the use of IRS to poorer and rural households where primary carers have low levels of education. Due to the significant cost associated with IRS, resources should be directed to assist the poorest households and communities where the use of ITN is less likely to be effective. Second, distribution of ITNs should be accompanied with a multi-media education program to enhance its protection. Television education offers the best protection compared to other forms. However, given its limitation in providing detailed information, other types of media should also be utilised. Care needs to be taken to ensure that families and carers who are less literate can be assisted to understand the information provided. Thirdly, more attention must be paid to educate mothers and mothers-to-be. The significant impact of an educated mother on the health outcomes of children suggests educating women not only benefits them by enhancing their human capital, but also benefits the family and community with improved child health. More effort and resources should be diverted to lift the education level of women in Ghana to benefit the nation as a whole. Inasmuch as this study does not observe a significant independent effect of ITN use on malaria infection, we recommend that future surveys consider continuous use of the ITN tool in order to ascertain its independent effect on malaria among this vulnerable group.
Although our study has outlined some policy implications, it is limited by the cross sectional nature of our data. Thus, our data makes it difficult to evaluate the efficacy of the intervention tools. Our findings demonstrate the associations rather than confirm effectiveness. Another limitation is associated with the measurement for ITN use. Since the surveyed households were only asked whether the child slept under ITN the night before, our ITN measure does not necessarily mean that the child had been protected by ITN on continuous basis. We would suggest future surveys to include a question on the frequency of the use of ITN in a longer period of time. Finally, the analysis is limited by our inability to control for some cofounders such as areas in Ghana that could have high malaria endemic. However, we believe that any bias coming from such exclusion is mitigated by the inclusion of rural dummy variable since such areas are more likely to be in rural areas.
Preliminary regressions suggested that mother and child use of ITN better explained malaria infection rate compared to when the child alone uses the net. This is due to malaria incidence for children sleeping under ITN alone, as mentioned earlier. The results on ITN use by child alone is available upon request.
Abbreviations
Anglogold Malaria
Behaviour change communication
Ghana demographic and health surveys
Ghana health service
Ghana statistical service
Indoor residual spraying
Insecticide treated bed-nets
Ministry of health
National Malaria control programme
National public health reference laboratory
Out Patient Department
Principal components analysis
Presidents Malaria initiative
Rapid diagnostic test
United Nations children’s fund
United States Agency for International Development
World Health Organization
Ghana Demographic and Health Survey (GDHS 2014): Rockville, Maryland, USA: GSS, GHS, and ICF International. 2015; 2014 http://dhsprogram.com/pubs/pdf/FR307/FR307.pdf . Accessed 4 Mar 2017.
Thuilliez J, d’Albis H, Niangaly H, Doumbo O. Malaria and education: evidence from Mali. J Afr Econ. 2017;26:443–69.
Article Google Scholar
Singleton G, Osei M. Ghana Malaria Prevention, Diagnosis and Data Programme, Annual Review. 2014. https://assets.publishing.service.gov.uk/media/57a089b0ed915d622c000357/Ghana-Malaria-Annual-Review-Report.pdf . Accessed 2 June 2016.
Asenso-Okyere WK, Dzator JA. Household cost of seeking malaria care. A retrospective study of two districts in Ghana. Soc Sci Med. 1997;45:659–67.
Article CAS PubMed Google Scholar
World Health Organisation (WHO). Health dimensions of economic reform. Geneva 1992. http://apps.who.int/iris/handle/10665/38231 . Accessed 3 June 2017.
World Health Organisation (WHO). World Malaria Report 2015. Available from: http://www.who.int/malaria/publications/world_malaria_report/en/ . Accessed 15 Oct 2017.
National Malaria Control Programme (NMCP, 2013) [Ghana]. Ghana Malaria Programme Review-Final Report. NMCP. Accra, Ghana. 2013 . https://www.ghanahealthservice.org/downloads/ghana_malaria_programme_review_final_report_june_2013.pdf . Accessed 4 Mar 2017.
National Malaria Control Programme (NMCP, 2016) [Ghana]. Ghana Malaria Control Programme Periodic Bulletin NMCP. Accra, Ghana. 2016. http://www.ghanahealthservice.org/downloads/NMCP_2016%20-Annual_Bulletin.pdf . Accessed 5 Mar 2018.
World Health Organisation (WHO). Indoor residual spraying: Use of indoor residual spraying for scaling up global malaria control and elimination. 2006. http://apps.who.int/iris/bitstream/10665/69386/1/WHO_HTM_MAL_2006.1112_eng.pdf . Accessed 4 Mar 2017.
Google Scholar
Mabaso ML, Sharp B, Lengeler C. Historical review of malarial control in southern African with emphasis on the use of indoor residual house-spraying. Tropical Med Int Health. 2004;9:846–56.
Loha E, Lunde TM, Lindtjørn B. Effect of bednets and indoor residual spraying on spatio-temporal clustering of malaria in a village in South Ethiopia: a longitudinal study. PLoS One. 2012;7:e47354.
Article CAS PubMed PubMed Central Google Scholar
Fuseini G, Ebsworth P, Jones S, Knight D. The efficacy of ACTELLIC 50 EC, pirimiphos methyl, for indoor residual spraying in Ahafo, Ghana: area of high vector resistance to pyrethroids and organochlorines. J Med Entomol. 2011;48:437–40.
Thomson M, Connor SJ, D’Alessandro U, Rowlingson B, Diggle P, Cresswell, Greenwood B. Predicting malaria infection in Gambian children from satellite data and bed net use surveys: the importance of spatial correlation in the interpretation of results. Am J Trop Med Hyg. 1999;61:2–8.
Killeen GF, Smith TA, Ferguson HM, Mshinda H, Abdulla S, Lengeler C, Kachur SP. Preventing childhood malaria in Africa by protecting adults from mosquitoes with insecticide-treated nets. PLoS Med. 2007;4:e229.
Article PubMed PubMed Central Google Scholar
Komazawa O, Kaneko S, K’Opiyo J, Kiche I, Wanyua S, Shimada M, Karama M. Are long-lasting insecticidal nets effective for preventing childhood deaths among non-net users? A community-based cohort study in western Kenya. PLoS One. 2012;7:e49604.
President’s Malaria Initiative (PMI). Insecticide-Treated Mosquito Nets (ITNs). 2017. https://www.pmi.gov/how-we-work/technical-areas/insecticide-treated-mosquito-nets-(itns)-pmi . Accessed 5 June 2017.
Afoakwah C, Nunoo J, Andoh FK. Effect of insecticide-treated bed net usage on under-five mortality in northern Ghana. Malar J. 2015;14:1–6.
Rhee M, Sissoko M, Perry S, McFarland W, Parsonnet J, Doumbo O. Use of insecticide-treated nets (ITNs) following a malaria education intervention in Piron, Mali: a control trial with systematic allocation of households. Malar J. 2005;4:1.
Ayi I, Nonaka D, Adjovu JK, Hanafusa S, Jimba M, Bosompem KM, Mizoue T, Takeuchi T, Boakye DA, Kobayashi J. School-based participatory health education for malaria control in Ghana: engaging children as health messengers. Malar J. 2010;9:1–12.
Roll Back Malaria Partnership (2015). Gender and Malaria. Available: http://www.rollbackmalaria.org/files/files/about/SDGs/RBM_Gender_Fact_Sheet_170915.pdf . Accessed 5 June 2017.
Cernetich A, Garver LS, Jedlicka AE, Klein PW, Kumar N, Scott AL, Klein SL. Involvement of gonadal steroids and gamma interferon in sex differences in response to blood-stage malaria infection. Infect Immun. 2006;74:3190–203.
Ghana Statistical Service. Ghana Living Standards Survey Round 6 (GLSS 6), Main Report. Accra: Ghana Statistical Service; 2014. http://www.statsghana.gov.gh/docfiles/glss6/GLSS6_Main%20Report.pdf .
Becker GS. Human capital: a theoretical and empirical analysis, with special reference to education. Chicago: University of Chicago Press; 2009.
Caldwell JC. Reflections health transition: the cultural, social and behavioural determinants of health in the third world. Soc Sci Med. 1993;36:125–35.
Justesen A, Kunst A. Postneonatal and child mortality among twins in Southern and Eastern Africa. Int J Epidemiol. 2000;29:678–83.
Heaton TB, Forste R, Hoffmann JP, Flake D. Cross-national variation in family influences on child health. Soc Sci Med. 2005;60:97–108.
Article PubMed Google Scholar
Arthur E. Wealth and antenatal care use: implications for maternal health care utilisation in Ghana. Heal Econ Rev. 2012;2:1–8.
Yakob L, Yan G. Modeling the effects of integrating larval habitat source reduction and insecticide treated nets for malaria control. PLoS One. 2009;4:e6921.
Ntonifor NH, Veyufambom S. Assessing the effective use of mosquito nets in the prevention of malaria in some parts of Mezam division, northwest region Cameroon. Malar J. 2016;15:390.
Pulford J, Hetzel MW, Bryant M, Siba PM, Mueller I. Reported reasons for not using a mosquito net when one is available: a review of the published literature. Malar J. 2011;10:1.
Hobcraft J. Women’s education, child welfare and child survival: a review of the evidence. Health Transit Rev. 1993;3:159–75.
CAS PubMed Google Scholar
Download references
Acknowledgements
The authors wish to thank Rachel Milte for her comments and two referees for their suggestions to improve the manuscript. All errors are the sole responsibility of the authors.
Availability of data and materials
The datasets analysed during the current study are available in the World Bank website repository, http://microdata.worldbank.org/index.php/catalog/2373/get_microdata .
Author information
Authors and affiliations.
School of Commerce, UniSA Business School, University of South Australia, GPO BOX 2471, Adelaide, SA, 5001, Australia
Clifford Afoakwah, Xin Deng & Ilke Onur
You can also search for this author in PubMed Google Scholar
Contributions
CA conceived the idea, performed the analysis and wrote the first version of the manuscript. XD contributed by revising the literature review and discussion of results. IO contributed to the empirical strategy and edited the manuscript. All authors read and approved the final manuscript.
Corresponding author
Correspondence to Clifford Afoakwah .
Ethics declarations
Ethics approval and consent to participate.
Not applicable.
Competing interests
The authors declare that they have no competing interests.
Publisher’s Note
Springer Nature remains neutral with regard to jurisdictional claims in published maps and institutional affiliations.
Rights and permissions
Open Access This article is distributed under the terms of the Creative Commons Attribution 4.0 International License ( http://creativecommons.org/licenses/by/4.0/ ), which permits unrestricted use, distribution, and reproduction in any medium, provided you give appropriate credit to the original author(s) and the source, provide a link to the Creative Commons license, and indicate if changes were made. The Creative Commons Public Domain Dedication waiver ( http://creativecommons.org/publicdomain/zero/1.0/ ) applies to the data made available in this article, unless otherwise stated.
Reprints and permissions
About this article
Cite this article.
Afoakwah, C., Deng, X. & Onur, I. Malaria infection among children under-five: the use of large-scale interventions in Ghana. BMC Public Health 18 , 536 (2018). https://doi.org/10.1186/s12889-018-5428-3
Download citation
Received : 19 December 2017
Accepted : 10 April 2018
Published : 23 April 2018
DOI : https://doi.org/10.1186/s12889-018-5428-3
Share this article
Anyone you share the following link with will be able to read this content:
Sorry, a shareable link is not currently available for this article.
Provided by the Springer Nature SharedIt content-sharing initiative
- Malaria infection
- Children under-five
- Sub-Saharan Africa
BMC Public Health
ISSN: 1471-2458
- General enquiries: [email protected]
Academia.edu no longer supports Internet Explorer.
To browse Academia.edu and the wider internet faster and more securely, please take a few seconds to upgrade your browser .
Enter the email address you signed up with and we'll email you a reset link.
- We're Hiring!
- Help Center
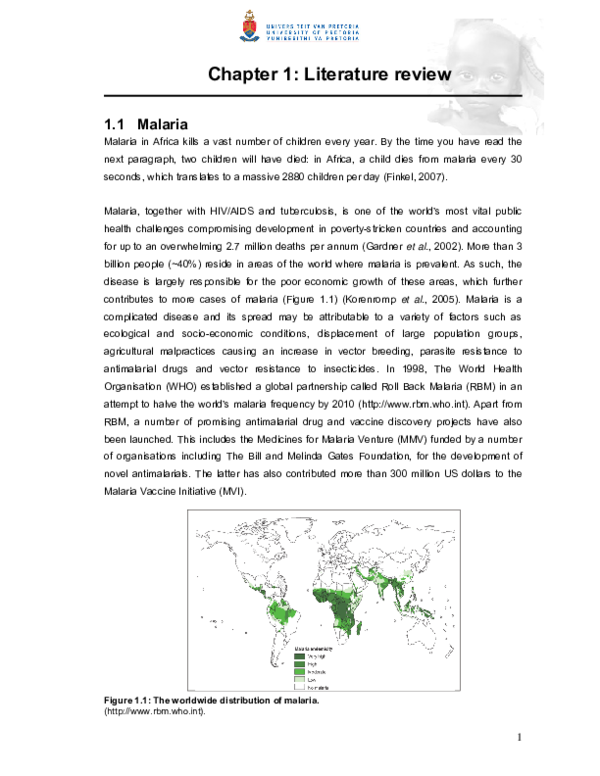
Chapter 1: Literature review 1.1 Malaria

2019, balarabe
i want to have little about aademia
Related Papers
Ranjan Ramasamy
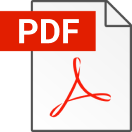
International Journal of Pharmaceutical Sciences Review and Research
NIKHIL Vanjari
Malaria, of the genus Plasmodium, is caused by the protozoan parasites. Most of the transmission is through the bite of an infected female anopheline mosquito. More than 500 million people are affected with malaria each year, resulting in 1-2 million fatalities. Falciparum is responsible for the majority of malaria deaths. Pregnant women, children, and anyone who are immunocompromised have the highest rates of morbidity and mortality. Myalgias or arthralgia, malaise or weakness, headache, and chills are the most common symptoms. Microscopy, antigen detection, and polymerase chain reaction (PCR) are among the procedures used to detect malaria parasites. Malaria in pregnancy is a major cause of severe maternal anemia, low birth weight neonates, preterm delivery, and higher infant and maternal death, with primigravidae experiencing these issues more frequently than multigravidae. Chloroquine is the first-line drug for treating three so-called benign malaria, Plasmodium vivax, Plasmodiu...
Thushara Balasuriya
• Malaria is the most important parasitic disease of mankind. It is now confined to the tropical and subtropical areas of Asia, Africa, South and Central America. Even so, nearly half of the population of the world may be exposed to the risk of malaria. • Four different species of malaria parasites infecting man, • Plasmodium vivax, • P. malariae • P.falciparum • P.ovale o Two species, P.vivax and P.falciparum account for about 95 % of all malaria worldwide, the other two being of relatively minor importance. Vectors o Human malaria is transmitted by the female Anopheles mosquito. The male mosquito feeds exclusively on fruit juices, but the female needs at least two blood meals before the first batch of eggs can be laid.
this is for malaria basic knowledge
Fatema Jawad
FEBS Journal
Renu Tuteja
Dr. Gourab Biswas
Malaria has both socioeconomic and geo-ecological impact. The evidence of malaria incidences were documented about 2500 years before the birth of Christ and after that investigations are going onto fight against this evil fever. According to World Health Organization (WHO) more than 200 million populations are exposed to health risk by malaria parasite and hence imposed a burden to our society. This paper is a review of the journey of malaria researches over the globe which now becomes a subject of concern both for control and awareness of the disease.
melita jukić
Malaria is a severe disease caused by parasites of the genus Plasmodium, which is transmitted to humans by a bite of an infected female mosquito of the species Anopheles. Malaria remains the leading cause of mortality around the world, and early diagnosis and fast-acting treatment prevent unwanted outcomes. It is the most common disease in Africa and some countries of Asia, while in the developed world malaria occurs as imported from endemic areas. The sweet sagewort plant was used as early as the second century BC to treat malaria fever in China. Much later, quinine started being used as an antimalaria drug. A global battle against malaria started in 1955, and Croatia declared 1964 to be the year of eradication of malaria. The World Health Organization carries out a malaria control program on a global scale, focusing on local strengthening of primary health care, early diagnosis of the disease, timely treatment, and disease prevention. Globally, the burden of malaria is lower than ...
Journal of Pharmaceutical Research International
savita pohekar
Introduction: Malaria is caused by the parasitic protozoan Plasmodium. It is a vector-borne disease which is transmitted from person to person via bites from infected mosquitoes. Following a mosquito bite the parasites multiply in the liver and subsequently infect red blood cells. It is a mosquito-borne illness. Fever or flu symptoms include shivering chills, headaches, and muscle pains. Anemia, jaundice, nausea, and diarrhea are some of the symptoms of malaria. Main Symptoms and Important Clinical Findings: A 24-year-old woman was admitted in. Acharya vinoba bhave rural hospital with chief complaint of stomach pain as her primary complaint. Then after several days Fever, pain, fatigue, headache occur. Several diagnostic evaluations done which shows total Red blood cells count: 4.8 million cu mm; white cell count: 11.810 cells mm3; lymphocytes: 11.2% platelets drop on smear, RBCs are moderated. The Main Diagnoses, Therapeutic Interventions, and Outcomes: 24 years old women admitted ...
SAFIYANU HAMISU
Malaria parasite and and their infection
RELATED PAPERS
Revista Educación Superior y Sociedad
Pablo Daniel Garcia
Sabrina Kholiqin
Journal of Vascular Surgery
Revista de História das Ideias
Leonel Ribeiro dos Santos
IEEE Access
Norhan Ahmed
Agronomía Colombiana
Bernardo Silva
Lecture notes in mechanical engineering
Arpad Palotas
MedEdPublish
NIKLAUS QUEK
BioResources
Jakub Gawron
Ahmad Fitriansyah
Journal of Geochemical Exploration
D. Gómez-gras
Revista do Instituto de Medicina Tropical de São Paulo
Fernando Elias
African Journal of Oral Health
Elizabeth Sote
Frontiers in Pediatrics
MOSHE BETSER M.D
Mining Engineering
Roussos Dimitrakopoulos
Chemischer Informationsdienst
David Dalton
16 de Abril
Roger Peralta
faruk yagci
South African Medical Journal
Angela Dell
npj Primary Care Respiratory Medicine
Ioanna Tsiligianni
Maarif Global Education Series
ismail karsantık
Journal of Chemical Research
Sabir Mashraqui
- We're Hiring!
- Help Center
- Find new research papers in:
- Health Sciences
- Earth Sciences
- Cognitive Science
- Mathematics
- Computer Science
- Academia ©2024
Thank you for visiting nature.com. You are using a browser version with limited support for CSS. To obtain the best experience, we recommend you use a more up to date browser (or turn off compatibility mode in Internet Explorer). In the meantime, to ensure continued support, we are displaying the site without styles and JavaScript.
- View all journals
- My Account Login
- Explore content
- About the journal
- Publish with us
- Sign up for alerts
- Open access
- Published: 22 May 2024
Household-level effects of seasonal malaria chemoprevention in the Gambia
- Seyi Soremekun ORCID: orcid.org/0000-0002-5531-0220 1 ,
- Bakary Conteh 2 ,
- Abdoullah Nyassi 2 ,
- Harouna M. Soumare 2 ,
- Blessed Etoketim 2 ,
- Mamadou Ousmane Ndiath 2 ,
- John Bradley ORCID: orcid.org/0000-0002-9449-4608 3 ,
- Umberto D’Alessandro 2 ,
- Teun Bousema ORCID: orcid.org/0000-0003-2666-094X 4 ,
- Annette Erhart 2 ,
- Marta Moreno 1 &
- Chris Drakeley ORCID: orcid.org/0000-0003-4863-075X 1
Communications Medicine volume 4 , Article number: 97 ( 2024 ) Cite this article
198 Accesses
1 Altmetric
Metrics details
- Epidemiology
- Preventive medicine
In 2022 the WHO recommended the discretionary expansion of the eligible age range for seasonal malaria chemoprevention (SMC) to children older than 4 years. Older children are at lower risk of clinical disease and severe malaria so there has been uncertainty about the cost-benefit for national control programmes. However, emerging evidence from laboratory studies suggests protecting school-age children reduces the infectious reservoir for malaria and may significantly impact on transmission. This study aimed to assess whether these effects were detectable in the context of a routinely delivered SMC programme.
In 2021 the Gambia extended the maximum eligible age for SMC from 4 to 9 years. We conducted a prospective population cohort study over the 2021 malaria transmission season covering 2210 inhabitants of 10 communities in the Upper River Region, and used a household-level mixed modelling approach to quantify impacts of SMC on malaria transmission.
We demonstrate that the hazard of clinical malaria in older participants aged 10+ years ineligible for SMC decreases by 20% for each additional SMC round per child 0–9 years in the same household. Older inhabitants also benefit from reduced risk of asymptomatic infections in high SMC coverage households. Spatial autoregression tests show impacts are highly localised, with no detectable spillover from nearby households.
Conclusions
Evidence for the transmission-reducing effects of extended-age SMC from routine programmes implemented at scale has been previously limited. Here we demonstrate benefits to the entire household, indicating such programmes may be more cost-effective than previously estimated.
Plain language summary
Seasonal malaria chemoprevention (SMC) is the provision of monthly, preventative, anti-malaria medication to young children at times when they are most at risk of severe disease. Recently the World Health Organisation recommended expanding SMC to children older than 4 years. Older children with malaria typically remain symptomless so the advantages were unclear. However, laboratory evidence suggests this group continues to transmit malaria to others. We conducted a population study in 2021 in 10 communities in the Gambia where SMC was extended to all children up to 9 years of age for the first time. We found household members aged over 9 years were less likely to get clinical disease when most young children in the same household did receive SMC. This suggests an added protection of SMC for those who do not receive it, potentially increasing cost-effectiveness.
Similar content being viewed by others
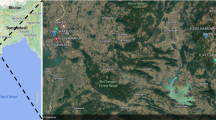
The effectiveness of malaria camps as part of the malaria control program in Odisha, India
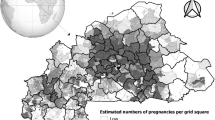
Bayesian Spatiotemporal Modeling of Routinely Collected Data to Assess the Effect of Health Programs in Malaria Incidence During Pregnancy in Burkina Faso
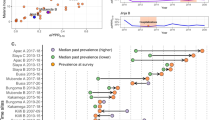
Severe outcomes of malaria in children under time-varying exposure
Introduction.
Seasonal malaria chemoprevention (SMC) with sulphadoxine-pyrimethamine and amodiaquine (SP-AQ) is a World Health Organisation-endorsed strategy to prevent severe malaria and malaria deaths in young children in living in the African Sahel/sub-Sahel, where most transmission occurs within a few months of the year 1 . Since this recommendation, SMC has been implemented in more than 10 countries covering over 20 million children. Trials have shown that SMC reduces clinical malaria in children aged 0–4 years by more than 80% in the first 4 weeks following a dose, and 67% 4–6 weeks following a dose 2 , 3 . In updated 2022 malaria control guidelines the WHO recommended control programmes could increase the SMC-eligible age to children above 4 years 4 to potentially mitigate a potential shift of the burden of severe malaria to older children as malaria transmission declines in endemic settings 5 , 6 , 7 . This was based on evidence from a 2010 stepped-wedge trial in Senegal showing a reduction of 61% in clinical cases in children 5–9 years receiving SMC 4 , 6 . However, more recent studies show mixed impact in this age group 8 , 9 and concerns about programme sustainability and maintenance of effective coverage in the current 0–4 age group have led to reservations about the feasibility and resources required to implement an age increase 10 .
Children aged over 5 years however have an increasing risk of asymptomatic infection and elevated gametocytaemia 11 ; recent studies of controlled mosquito feeding experiments 12 , 13 , 14 , 15 and simulation analyses 16 have demonstrated asymptomatic infections in children in this age group are also often the largest contributors to malaria transmission. This suggests extended-age SMC, besides reducing clinical illness in direct recipients, may have wider impacts on transmission to non-recipients 6 , 17 , 18 . For policy-makers with finite resources, interventions that maximise impact on both disease transmission and clinical disease burden are prioritised, particularly when the goal is elimination 19 . The transmission-reducing impact of vector control interventions are well-described 20 , 21 , 22 and incorporated into models of cost-effectiveness by design as programme impact is frequently measured in the entire population targeted 20 , 23 , 24 . Vector control programmes are predicted to be highly cost-effective and usually prioritised for implementation 19 , 24 . The impacts of SMC programmes are normally measured only in eligible children, thus the true value of extended-age implementation is likely to be persistently underestimated, highlighting a crucial evidence gap for implementation design and policy.
In 2021, the National Malaria Control Programme (NMCP) of the Gambia Ministry of Health recommended increasing the eligible age for SMC to 9 years 25 . Given the highly clustered nature of malaria transmission, particularly in this context 26 , 27 , herd impacts of the SMC programme should be primarily detectable within mixed-age households using household-level statistical approaches. Therefore as part of a larger study of malaria infection and transmission dynamics conducted in 2019–2022, ‘The INDIE Trial’ 28 , we conducted a prospective cohort study collecting population data on SMC coverage and malaria burden across communities in Gambia’s Upper River Region to assess the individual and household-level impacts of the extended programme. We find that in households where most children had received at least one round of SMC, the incidence of clinical malaria and prevalence of asymptomatic infections is lower in adolescents and adults who are themselves non-eligible for SMC compared to households with lower coverage of SMC in children—these results are robust to adjustment. This suggests transmission effects are detectable in routine programmes and extended-age SMC may have additional cost-benefits beyond reducing disease in target children.
The study was implemented in ten communities (Njayel, Banni Kunda, Temanto, Bolibana, Fula Mori Bochi, Madina Samba Sowe, Njum Bakary, Sare Demba Dardo, Sare Biram and Tabajang) in the Upper River Region (URR) of the Gambia (Fig. 1 ). The region has a population of over 250,000 inhabitants and has a distinctly seasonal pattern of malaria transmission, where the most cases occur between June and December, peaking directly following the annual rains between June and September 29 . The main vector species is Anopheles gambiae sensu latu 30 , 31 . During the 2021 season, the mean nightly Anopheles gambiae s.l . and Anopheles funestus catch rate per house in the study communities using Centres for Disease Control light traps rate was 0.97 (standard deviation 1.41) (Supplementary File STable 1 ). The peak temperature during the transmission season was 35.2 °C in 2021 (Supplementary File STable 2 ). A region-wide distribution drive of long lasting insecticide treated nets occurred in 2019. For the first time in July 2021, The NMCP extended the upper eligible age for SMC from 4 to 9 years. Eligible children aged 0–9 years could receive up to four rounds of monthly SMC with sulphadoxine-pyrimethamine (SP) and amodiaquine (AQ) administered between August and November 2021 by community outreach teams.
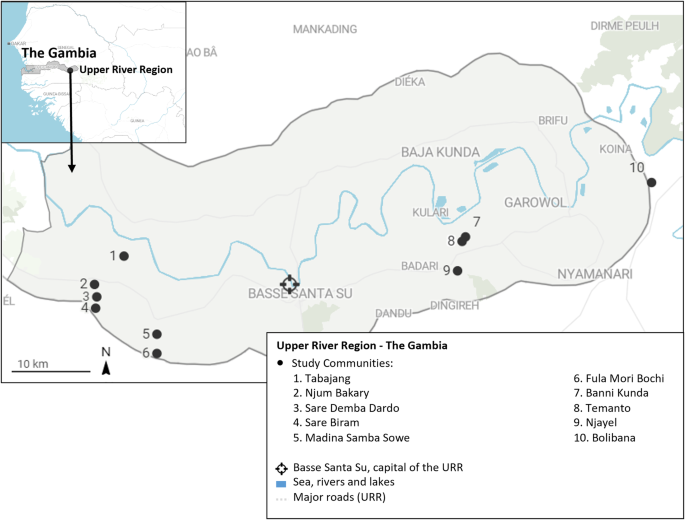
The locations of the ten study communities are denoted by black markers in the Upper River Region of The Gambia. The inset shows the location of the region within the country.
Study design
This prospective observational cohort study was nested within a larger trial measuring the effectiveness of control interventions on malaria infection and transmission, the INDIE Trial ( P. falciparum Infection Dynamics and Transmission to Inform Elimination, clinicaltrials.gov reference NCT04053907) and used data collected between 26th July 2021 and 12th January 2022. Three communities (Njum Bakary, Sare Demba Dardo and Sare Biram) were randomly assigned to receive 3 monthly rounds of mass drug administration with dihydroartemisinin-piperaquine between April and June 2021. In two communities (Madina Samba Sowe and Tabajang) inhabitants were screened weekly for fever—‘fever screen and treat (FSAT)’, those with temperatures above 37.5 °C or a history of fever in the last week received a rapid diagnostic test (RDT) for malaria and positive cases were treated with artemether-lumefantrine. Three communities (Banni Kunda, Temanto and Njayel) were assigned to monthly screening of all residents with ultrasensitive-RDTs, and positive cases treated with artemether-lumefantrine—‘mass screen and treat’ (MSAT). The remaining two communities (Bolibana and Fula Mori Botchi) were assigned to the control group and received standard control interventions. A programme of community case management of malaria was concurrently initiated in all communities. From 26 July 2021, a clinician and support worker (nurse and community health worker) based in each community managed all suspected malaria cases (passive case detection—PCD).
Study procedures
A series of community-based sensitisation activities took place during the INDIE baseline year (2019) to inform community members about the study and its aims, and all inhabitants were invited to take part. Residents who provided informed consent were enroled during a baseline dry season survey in April-May 2021. Cross-sectional surveys covering all enroled community members were carried out on a rolling basis every 8 weeks during the transmission season. Blood samples collected from the enroled population during all surveys were analysed at the MRC Unit The Gambia (MRCG) in Basse by quantitative polymerase chain reaction (qPCR varATS ) to detect malaria infections. Study questionnaires were administered during the surveys to all participants and collected address details and GPS coordinates, demographics including age, gender and ethnicity, symptoms of illness, care seeking and any treatments received for each participant and use of insecticide-treated bed nets the night prior to the survey. Identical data were collected from malaria patients identified by PCD visits although diagnosis was done by RDT only. The dates and number of all SMC rounds received for each child under 10 years were collected from SMC cards or by caregiver report. SMC data were collected in a survey after the last (November) round of SMC, and at two additional capture points: during a final cross-sectional survey in January 2022 and following a review of the SMC database by SS and AN, in a dedicated SMC mop-up survey to address data queries and collect data on additional study children not met in previous surveys (10th–15th March 2022). Entomological surveillance was conducted during the 2021 study period in 6 randomly selected households per community. In each household, Centre for Disease Control (CDC) light traps were hung in a sleeping room for three consecutive nights per month; caught mosquitoes were examined to determine species, sex and gonotrophic status (fed status and gravidity).
Study outcomes
All data from PCD visits, cross-sectional and entomological surveys were collected onto secure handheld devices and stored, cleaned and accessed via a password protected REDcap® server (Vanderbilt University, Nashville Tennessee) hosted at the MRC LSHTM Research Unit in Fajara. Incidence of clinical malaria was defined as the number of passively detected cases per 100 person-months. For each clinical case, 2 weeks of follow up were removed from the denominator. Malaria prevalence was defined as the percentage of participants sampled who were qtPCR-positive during a late season survey (27th September to the 27th November 2021). qtPCR-positive participants sampled during this survey were categorised into high- and low-density infections by age group. High-density infections were those with density above the median of the natural log asexual parasite concentrations per µL blood for the age groups (0–4, 5–9, 10–15 and 16+ years).
Statistical analysis
The impact of SMC status on the incidence of clinical malaria in eligible children was assessed by fitting shared frailty cox models with gamma-distributed random effects specified for person to account for the possibility of repeated clinical episodes within the same person (failures). Where frailty models did not stabilise, robust errors were used instead. To account for ongoing malaria control interventions in each community and background community level risk of malaria, the models pre-specified fixed effects for village ID. Models also included fixed effects for the percentage of all visits to the participant at which an insecticide-treated bed net was used the night before and household ID.
To assess the association between household level coverage of SMC in eligible children and malaria burden in adolescents/adults 10+ years of age, we first defined household SMC coverage in two ways: (i) the percentage of children 0–9 years in each household who received at least 1 round of SMC and (ii) the mean number of rounds of SMC per child. Both definitions were implemented as continuous variables and classed into ‘low’, ‘moderate’ and ‘high’ groups thus: <25%: 25–79%, 80%+ (definition 1) and <0.3, 0.3–2 and >2 rounds/child (definition 2), respectively. The high group cut-offs (80% or >2 rounds) were fixed to be consistent with the optimum minimum level of coverage of child health programmes 32 , 33 , 34 . The low/medium cut-off values were varied for definition 1 in the range 0–55% in 5% increments and for definition 2 in the range 0–0.9 in 0.1 unit increments and implemented in household level models described in Supplementary File 1 STable 3 . The models with low groups of <25% and 0.3 had the lowest Akaike and Bayesian Information Criteria values (AIC and BIC) and were thus selected as the final low-group cut-offs in all household level models.
The impact of household-level coverage of SMC as defined above on clinical malaria episodes in participants 10+ years of age was then assessed by fitting shared frailty Cox models to account for repeated clinical episodes as previously. The impact of household-level coverage of SMC on malaria prevalence in participants 10+ years of age was estimated using logistic regression models with a household-level random effect. We visualised parasite concentration distributions in participants aged 10+ years stratified by household SMC coverage by the generation of kernel density estimate plots and estimated the impacts of household SMC coverage on the prevalence of high-density infections in this group using logistic regression models with a household-level random effect. Equivalent data in children 0–4 and 5–9 years were limited by fewer malaria infections and smaller cell sizes, therefore the additional household-level impacts of SMC on eligible children themselves were assessed in all eligible children aged 0–9 years combined (STables 7 , 8 Supplementary File). Where multilevel models failed to converge, we calculated household-level estimates of malaria burden in this age group and regressed these against household-level SMC coverage using a two-stage technique to adjust for individual-level covariates 35 , 36 . All individual and household-level models of impact of SMC were implemented in Stata version 17.0 (StataCorp, Texas USA).
All household-level models pre-specified fixed effects for village ID, the percentage of all visits to households at which inhabitants used an insecticide-treated bed net the night before, the household level prevalence of malaria at a baseline dry season survey in April-May 2021, age in years, the household ratio of children aged 0–9 years to participants aged 10+ years, the total number of household inhabitants and for prevalence models, the week of survey visit.
To assess the effect of spatial clustering of household-level impacts of SMC, an inverse weighted distance matrix was created describing the Euclidean distances (km) between all households in the study site. We calculated the mean incidence rates of clinical malaria per participant aged 10 years and older per enumerated household, and the mean incidence rate in children 0–9 years per household and attached additional features of SMC coverage, insecticide-treated bed net usage, compound size and age composition, and geolocation (latitude and longitude) to each household. Identical datasets were constructed with the overall prevalence of asymptomatic infection per household during the late-season survey for the same age groups. Visual examples of the resulting datasets are shown in Supplementary Fig. 2 (Supplementary File). The distance matrix was used to calculate global Moran indices and z-scores to test for spatial autoregression under the hypothesis that prior regression estimates of the association between household-averaged outcomes for incidence and prevalence of malaria and household SMC coverage were not influenced by estimates in nearby households. Models were adjusted for household level covariates as previously stated - percentage of all visits to households at which inhabitants used an insecticide-treated bed net the night before, the household level prevalence of malaria at a baseline dry season survey in April-May 2021, the household ratio of children aged 0–9 years to participants aged 10+ years and the total number of household inhabitants. Visualisation of spatial data, construction of distance matrices and spatial analysis was conducted using Seaborn, Geoplot, Geopandas and the Esda_Moran libraries in Python (Python Software Foundation, Python Language Reference, version 3.9).

Ethical approval
This study did not collect any new data or implement additional activities not previously covered by approvals already in place for the INDIE study. Ethical approval for the INDIE study was provided by the Government of The Gambia/The MRC Gambia Joint Ethics Committee, The Gambia, and the Ethics Committee of the LSHTM, UK (reference: 16642).
Reporting summary
Further information on research design is available in the Nature Portfolio Reporting Summary linked to this article.
Characteristics of the study sample
Census data for the site (year 2021) was provided by the Health and Demographic Surveillance System (DSS) team for the Basse District Area. The estimated combined population for the ten study communities was 2839 residents in 144 households, of which 2435 participants in 133 households were enroled into the main INDIE study. The present study included 2210 participants (825 aged 0–9 years and 1,385 participants aged 10+ years) in 129 households who were met during the 2021 malaria transmission season and had complete address data (Fig. 2 ). Supplementary data 1 summarises the characteristics of study households by the categories of coverage of children with 1+ round SMC in children aged 0–9 years, and Supplementary data 2 summarises the same information by categories of the mean number of SMC rounds per child. Most households had at least 1 malaria case over the season and most had inhabitants in each age category, though a smaller proportion of households within the lowest household SMC coverage categories had inhabitants aged 0–4 years (69.2%) compared to the overall proportion (89.9%). These households also tended to be smaller than the average (10 versus 17 mean inhabitants respectively).
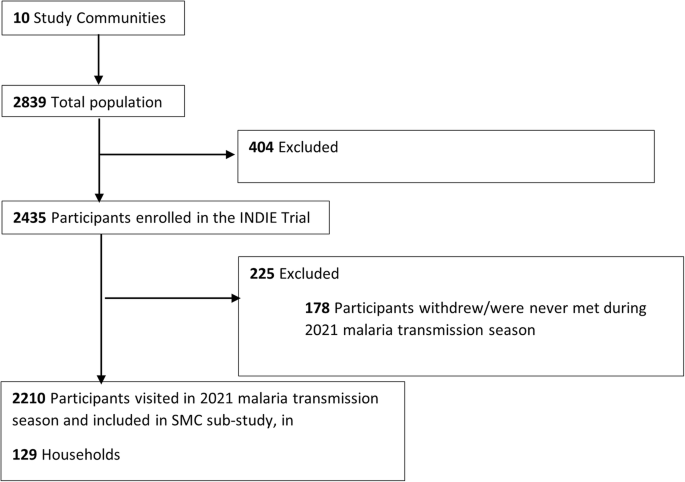
All residents of the study site were eligible to participate in the study and 87% were enroled. Only participants who were present in at least one survey during the 2021 malaria season (July-December) with full address data were included in the final analysis sample.
Impact of SMC status on malaria in eligible children
Dates of SMC rounds were extracted directly from SMC cards for 68.3% of children, and based on caregiver reports for 31.6% of children who had received at least 1 round of SMC. There was no SMC cards available for children who did not receive SMC. Overall, 76.0% of children aged 0–9 years received at least one round of SMC in 2021, and 20.9% received four rounds. Coverage was highest in children aged 0–4 years (90.6% and 31.5% received SMC at least once and all four rounds, respectively) than those aged 5–9 years (64.0% at least one round, 12.1% all four rounds, respectively)(Table 1 ) .
The incidence rates (IR) of clinical malaria over the 2021 season in children eligible for SMC are shown in Table 2 and Kaplan-Meier plots for clinical malaria by SMC coverage are displayed in Supplementary Fig. 1 (Supplementary File). IRs for clinical malaria in children who received at least 1 round of SMC was 1.58 episodes per 100 person-months of follow-up, compared to an IR of 2.89/100 person-months in children who received none. This was highly significant in both unadjusted and adjusted Cox models (adjusted hazard ratio 0.44 95% CI 0.21, 0.93; p value 0.031). The largest reductions were seen in children who received 3–4 rounds of SMC (adjusted hazard ratio 0.38 95% CI 0.16, 0.92; p value 0.031). Reductions were also significant when stratified by age; hazard of clinical infection was very low in children 0–4 years of age who received 1 or more rounds of SMC in the adjusted model. However, associations between SMC and clinical malaria in children aged 5–9 years although consistent with an impact, did not remain significant after adjustment.
STable 4 (Supplementary File) shows malaria prevalence in eligible children by SMC status. Prevalences were low, and lowest in children aged 0–9 years who received 3 or 4 rounds of SMC (2.6%), and higher in those who received only 1–2 rounds (6.5%). Prevalence in children who received no SMC was 4.4%. No impact of SMC coverage on malaria prevalence remained significant in the adjusted models.
Household level effects of SMC coverage
In Cox models with SMC coverage specified as a continuous exposure, there was an inverse and significant association between clinical malaria in participants 10 years or older and coverage of one or more rounds of SMC in younger children in the same household (HR: 0.99; 95% CI: 0.99, 1.00; p = 0.039) and a close to significant inverse association between mean rounds of SMC per child per household and clinical malaria in older participants (HR 0.80; 95% CI: 0.63, 1.02; p = 0.069). Predicted trends from these models indicate a 10% decrease in hazard for a 10% rise in the percentage of children who received any SMC, or a 20% decrease in hazard for each additional round of SMC received per eligible child.
In categorical models, we similarly observed reduced incidence of clinical malaria in participants aged 10 years and older with increasing household coverage of SMC (Fig. 3 ). After adjustment, the reductions were significant or close to significant and of similar magnitude when SMC coverage in the same household was moderate (25–79% of children received 1+ round SMC—adjusted HR: 0.60; 95% CI: 0.34, 1.07; p = 0.084), or high (80% or more received 1+ rounds SMC—adjusted HR: 0.49; 95% CI: 0.28, 0.86; p = 0.014) compared to households where coverage was low (<25%) (Table 3 ) . Similar significant reductions in clinical malaria were observed in households defined by mean number of SMC rounds per child (Fig. 3 and Table 3 ).

Vertical axes start at ‘60% no clinical malaria at time = t’ for clarity. SMC coverage is defined as ( a ) percentage of eligible children in household with 1+ rounds of SMC, where the blue line is participants in households with <25% coverage, the orange line refers to those in households with 25–<80% coverage and the green line those with 80%+ coverage. b Mean number of SMC rounds per eligible child, were blue line is participants in households with <0.3 rounds/child, the orange line refers to those in households with 0.3–<2 rounds per child, and the green line those with 2+ rounds per child. Grey vertical lines indicate the first reported day of each monthly round of SMC.
There was a consistent trend of reduced clinical malaria in children 0–9 years with increasing household coverage of SMC, however, this trend was not significant in the fully adjusted models - except for children who received no SMC residing in households with otherwise high coverage (STable 6 Supplementary File).
Unadjusted continuous mixed effects logistic models estimated significant reductions in malaria prevalence with increasing household SMC coverage in 1056 participants aged 10+ years visited in the late malaria season (unadjusted OR: 0.98; 95% CI: 0.97, 0.10; p = 0.022 and OR: 0.64; 95% CI 0.42, 0.97; p = 0.034 for SMC coverage defined as percentage of children in household with 1+ SMC rounds or mean SMC rounds per child in household, respectively). These effects did not remain significant after adjustment (adjusted OR: 0.99; 95% CI: 0.98, 1.00; p = 0.200 and OR: 0.82: 95% CI: 0.55, 1.23; p = 0.341 for SMC as previously). When SMC coverage was categorised as low, medium or high, malaria prevalence in older participants ranged from 6.8% in high coverage households to 18.8% in low coverage households (Fig. 4a, c ). In categorical mixed effects models, malaria prevalence in participants aged 10+ years was lowest in households in the two highest coverage groups (moderate and high groups) and both unadjusted and adjusted models suggested a strong decreasing trend in prevalence with increasing SMC coverage (Table 4 ). The inverse was observed on parasite densities in infected individuals: as household SMC coverage increased, the distribution of parasite densities skews higher (Fig. 4b, d ) and the risk of high-density infections increases four-fold or more in households in higher coverage groups (Table 4 ).

a Prevalence of asymptomatic infection in all participants aged 10+ years, by the coverage of SMC in young children in the same household. SMC coverage is defined as the percentage of children 0–9 years who received at least one round of SMC in three groups: <25% (blue bar), 25–75% (orange bar) and 80%+ (green bar). b Kernel density plot showing the log (natural) parasite concentrations originally measured in counts per μL in PCR-positive participants aged 10+ years. Plots are stratified by SMC coverage in children 0–9 years in the same household, defined as the percentage of children 0–9 years who received at least one round of SMC in three groups: <25% (blue plot), 25–75% (orange plot) and 80%+ (green plot). Median parasite concentrations for each SMC coverage group are shown by dotted vertical lines (colours to match SMC coverage category); cut-offs for high-density infections (averaged for age groups 10–15 and 16+ years) are shown as solid black lines. c Prevalence of asymptomatic infection in all participants aged 10+ years, by the coverage of SMC in young children in the same household. SMC coverage is defined as the mean number of SMC rounds per child also in three groups: <0.3 (blue bar), 0.3–2 (orange bar) and >2 (green bar). d Kernel density plot showing the log (natural) parasite concentrations originally measured in counts per μL in PCR-positive participants aged 10+ years. Plots are stratified by SMC coverage in children 0–9 years in the same household, defined as the mean number of SMC rounds per child also in three groups: <0.3 (blue plot), 0.3–2 (orange plot) and >2 (green plot). Median parasite concentrations for each SMC coverage group are shown by dotted vertical lines (colours to match SMC coverage category); cut-offs for high-density infections (averaged for age groups 10–15 and 16+ years) are shown as solid black lines.
Malaria prevalence was relatively low in 684 children 0–9 years visited in the same survey (all <6.5% STable 7 Supplementary File). We detected no significant associations between household SMC coverage and malaria prevalence in children 0–9 years in adjusted models.
Spatial impacts of household level SMC coverage
We successfully enumerated 90% (129/144) of all households in the study site. Following regression of mean household IRs or percent prevalence against household level SMC coverage, we assessed the degree of spatial autocorrelation of model residuals using tests of Moran’s I. P values for all models exceeded 0.05 (0.90–0.99) indicating no spatial clustering of our household level modelling outcomes (Supplementary Fig. 3 Supplementary File).
Empirical evidence for the impact of SMC on malaria transmission is limited but has critical implications for programme scale-up 18 . In this study, we have applied a household-level modelling approach to assess the effects of extended-age SMC on older household inhabitants in a pre-elimination setting in the Gambia. In adjusted models, we observed a significant reduction in the incidence of clinical malaria in eligible children who received SMC compared to those who did not. We observed that older participants ineligible for SMC in households with higher coverage of SMC were significantly less likely to contract clinical malaria compared to households where SMC coverage was low. In a survey of the population late in the malaria transmission season we also observed fewer asymptomatic infections in older participants in households with higher SMC coverage. There was no evidence of spatial clustering of these impacts.
Whilst the incidence of clinical malaria in SMC-eligible children aged 0–9 years decreased with increasing household level SMC coverage and irrespective of their individual level SMC status, we could not reliably demonstrate this constituted an additional benefit of SMC in our adjusted models (STable 6 Supplementary File) neither did we detect an impact of household level coverage of SMC on asymptomatic infections in SMC-eligible children (STable 7 Supplementary File).
The impacts observed in this study broadly align with the results of a 2008-2010 pilot stepped wedge trial which experimentally increased the eligible age range for SMC in Senegal to 10 years 6 —to our knowledge this is the only other source of data on clinical impact following SMC in this age group. The authors reported a decrease in the incidence of clinical malaria in eligible children (69%) and in older age groups (26%). The study did not consider the impacts of lower coverage or impact on asymptomatic infections, and was implemented with excellent research fidelity and high SMC coverage over the three years of implementation, where 84–93% of children received 3 rounds of SMC. A key question for decision-making is whether similar impacts on malaria transmission are achievable in routinely delivered large-scale programmes. Following an era of efficacy and effectiveness trials of SMC initially demonstrating high programme coverage and large effects in study populations, emerging data from routine SMC programmes in West Africa suggests mixed, or reduced impact 8 , 9 , 32 , 37 , 38 . In our study, coverage of children receiving 3 or more rounds of SMC was 49% overall and only 36% of children 5–9 years; however this was the first season in which the NMCP of the Gambia extended the eligible age for SMC, and the coverage may reflect the initial complexities in operationalising the change. It nonetheless suggests transmission impacts are detectable in the context of programmes with moderate implementation strength.
There is an extensive theoretical basis from both laboratory and field studies which suggest the infectious reservoir for falciparum malaria in sub-Saharan Africa is normally maintained by children up to 15 years of age 12 , 15 , 16 , and the expected extended benefits of preventative interventions in this age group should be observable in non-eligible adolescents and adults 18 . We justify the use of a household-level models to assess impact of SMC on transmission based on previous research in The Gambia 26 and similar low to moderate endemic settings 27 which indicate malaria transmission is highly localised within household/family groups 39 with negligible contribution from nearby households. This is supported by the null findings from our global Moran tests for spatial autocorrelation in household-level SMC models in the study site. Based on this proposed framework, we hypothesised the mechanism through which herd impacts of SMC arise will be by first reducing infections in the target age group in the same household. We identified a significant reduction in clinical infections in children 0–9 years, but only decreased asymptomatic infections in children with 3 or more rounds of SMC which did not reach significance (Supplementary File 1 STable 4 ). Interestingly children who received only 1–2 rounds of SMC benefitted in terms of reduced incidence of clinical episodes but had increased risk of asymptomatic infections compared to children receiving no SMC, though overall prevalences were low (<6.5%). Children who had received only 1–2 SMC round were also more likely to have received SP in the first half of the season (Supplementary File 1 STable 5 ) perhaps leading to a slightly elevated risk of infection in the following months of rising transmission due to naïve immunity—however, given low prevalence estimates we cannot rule out the potential presence of sampling error. The evidence for the transmission-blocking potential of SP-AQ is conflicting; in vivo and in vitro studies have indicated exposure to SP may increase commitment of blood stage parasites to sexual differentiation resulting in increased gametocyte concentrations and potential increased transmission potential to feeding mosquitoes 40 , 41 , 42 however detailed analysis of this phenomenon suggests impacts occur for a small number of drug classes under a narrow drug concentration window, and are unlikely to result in a net increase in transmission potential 43 . Pyrimethamine has also shown suppressive activity against oocyte production or successful development of sporozoites in the mosquito 44 , 45 , which may offset increased gametocytogenesis with use of SP. Field studies support a theory of increased gametocytogenesis following SP use or SMC but are also characterised by small sample sizes and incomplete accounting for potential confounding, and alone do not resolve this conflict 46 , 47 . Whilst gametocyte concentrations were not available for participants in our study, we did observe a trend of increased parasite concentrations in children with SMC and in older participants in households with higher SMC coverage known to be positively associated with gametocyte density 12 , however, infection rates were too small to confirm this relationship. Other studies have shown that densities of both asexual and gametocyte stages are nonetheless considerably lower in asymptomatic infections compared to clinical disease 48 , 49 . This current body of evidence highlights the mechanisms of action of SP-AQ SMC are complex and not yet fully elucidated; the net product of a trade-off between reduced clinical infection, increased asymptomatic parasitaemia and gametocytaemia and potential suppressive effects on mosquito lifecycle stages may be a reduction in overall transmission potential.
An assessment of the transmission-reducing effects of SMC is most useful for policy if based on results of routinely delivered programmes. This setting better predicts real-life impacts under sub-optimal or heterogenous SMC coverage and in the presence of other nationally implemented malaria interventions. High intervention coverage and strict eligibility criteria for participants and clusters observed in trial settings often do not transfer with high fidelity or effectiveness for this reason. Nonetheless, a limitation of our observational approach is determining the appropriate techniques to account for other exogenous predictors of transmission that may also differ by SMC coverage, not all of which can be measured. We included a priori fixed effects in statistical models to address this. Village ID was used as a proxy for underlying transmission potential for a community, clustering by ethnicity and in the context of the wider INDIE study also allowed us to adjust for ongoing interventions that differed by community. We also included household-level baseline malaria infection prevalence and indicators of individual or household level bed net usage - variation in treated bed net usage can predict mosquito biting rates, local intensity of malaria transmission and/or potential to adhere to other protective behaviours and may correlate with higher acceptability and adherence to SMC programmes. We examined other characteristics of households by SMC coverage group (Supplementary Data 1 , 2 ) to identify potentially confounding characteristics. All else being equal, the household impact of SMC may be influenced by the number of children relative to older inhabitants and/or total household size. These parameters differed by SMC coverage level in this population. We, therefore, addressed these sources of potential confounding by presenting both unadjusted models and models adjusted for the above features with correction of errors to account for clustering at person or household level. We excluded some households from our spatial analysis sample - which detected no geographical clustering of effects of household-level models of SMC - however the vast majority of households (90%, 129/144) across the site were successfully enumerated and the missing (15 households) were spread amongst all communities (Supplementary Fig. 2 ). Given P values for Moran tests were close to 1 it is unlikely data from the 15 excluded households would significantly modify these. As a robustness test, we defined coverage of SMC in two ways, as the percentage of children receiving any SMC, and the mean number of SMC rounds per child with both definitions showing similar patterns of impact. There may be some misclassification of SMC status as the NMCP did not have a policy of directly observed treatment for SMC at the time, and the status for a third of children was based only on caregiver recall. However given the short length of the transmission season and the short length of time between our surveys and the SMC rounds themselves, we expect the levels of misclassification to be relatively low. Our study did not allow us to separate differential herd impacts of SMC coverage by age (0–4 years and 5–9 years), highlighting a potential focus for further research. We also did not perform a detailed assessment of additional herd impacts of household SMC coverage on eligible children irrespective of their own status. In both cases malaria outcomes were relatively less common in this group and in the case of the second initially significant positive effects did not survive adjustment for key confounders. Given wide confidence intervals (STable 6 ) including the potential for both very large reductions or increases in hazard of clinical malaria, it is likely that this sub-analysis is underpowered. Therefore the possible impacts in this age group therefore remain to be established in future studies. Whilst a cost analysis was outside the scope of this study, estimates from the Senegal study where SMC was extended to the same age range suggest the programme can be implemented with an average delivery cost of $0.50 per child per month 6 , however, additional context-specific data of extended programmes from other sites would be useful for policy. Our study was conducted in a moderate/low transmission setting—future studies in higher burden settings will add to this growing evidence base.
In conclusion, we show in the context of a routine extended-age chemoprevention programme with SP-AQ that SMC is associated with a significant reduction in clinical malaria in direct recipients and with a significant reduction in clinical and asymptomatic infection in older household inhabitants. Our data demonstrate these effects are robust to adjustment, are household-specific with little negligible from nearby households. The results support findings of previous laboratory and simulation studies of the importance of children to the infectious reservoir for malaria, addressing a critical evidence gap in demonstrating herd effects in practice. They point to important additional benefits of SMC in reducing overall malaria transmission, highlighting such programmes are likely to be more cost-effective than currently estimated.
Data availability
Study data (for Tables and in-line results) will be made publicly accessible after the publication of the impact paper for the INDIE 1b project. Datasets will be available via the LSHTM Data Compass data repository ( https://datacompass.lshtm.ac.uk/ ), or from the corresponding authors upon reasonable request. The source data behind the graphs in the figures can be found in Supplementary Data 3 .
WHO. WHO Policy Recommendation: Seasonal Malaria Chemoprevention (SMC) for Plasmodium falciparum malaria control in highly seasonal transmission areas of the Sahel sub‐region in Africa March 2012. https://www.who.int/malaria/areas/preventive_therapies/children/en/ (2012).
Cairns, M. et al. Effectiveness of seasonal malaria chemoprevention (SMC) treatments when SMC is implemented at scale: case-control studies in 5 countries. PLoS Med. 18 , e1003727 (2021).
Article PubMed PubMed Central Google Scholar
Baba, E. et al. Effectiveness of seasonal malaria chemoprevention at scale in west and central Africa: an observational study. Lancet 396 , 1829–1840 (2020).
Article Google Scholar
WHO. WHO Consolidated Guidelines for Malaria (World Health Organization, 2022).
Okiro, E. A. et al. Age patterns of severe paediatric malaria and their relationship to Plasmodium falciparum transmission intensity. Malar. J. 8 , 4 (2009).
Cissé, B. et al. Effectiveness of seasonal malaria chemoprevention in children under ten years of age in Senegal: a stepped-wedge cluster-randomised trial. PLoS Med. 13 , e1002175 (2016).
WHO. Updated WHO recommendations for malaria chemoprevention among children and pregnant women. https://www.who.int/news/item/03-06-2022-Updated-WHO-recommendations-for-malaria-chemoprevention-among-children-and-pregnant-women (2022).
Diawara, S. I. et al. Effect of seasonal malaria chemoprevention in children between 5 and 9 years old in Kita and Bafoulabe districts, Mali. Parasite Epidemiol. Control 18 , e00258 (2022).
Konaté, D. et al. Effectiveness and community acceptance of extending seasonal malaria chemoprevention to children 5 to 14 years of age in Dangassa, Mali. Am. J. Trop. Med. Hyg. 106 , 648–654 (2022).
Moukénet, A. et al. Extending delivery of seasonal malaria chemoprevention to children aged 5–10 years in Chad: a mixed-methods study. Glob. Health Sci. Pract. 10 , e2100161 (2022).
Stone, W., Gonçalves, B. P., Bousema, T. & Drakeley, C. Assessing the infectious reservoir of falciparum malaria: past and future. Trends Parasitol 31 , 287–296 (2015).
Article PubMed Google Scholar
Andolina, C. et al. Sources of persistent malaria transmission in a setting with effective malaria control in eastern Uganda: a longitudinal, observational cohort study. Lancet Infect. Dis . 21 , 1568–1578 (2021).
Githeko, A. K. et al. The reservoir of Plasmodium falciparum malaria in a holoendemic area of western Kenya. Trans. R. Soc. Trop. Med. Hyg. 86 , 355–358 (1992).
Article CAS PubMed Google Scholar
Gonçalves, B. P. et al. Examining the human infectious reservoir for Plasmodium falciparum malaria in areas of differing transmission intensity. Nat. Commun. 8 , 1133 (2017).
Rek, J. et al. Asymptomatic school-aged children are important drivers of malaria transmission in a high endemicity setting in Uganda. J. Infect. Dis. 226 , 708–713 (2022).
Coalson, J. E. et al. Simulation models predict that school-age children are responsible for most human-to-mosquito Plasmodium falciparum transmission in southern Malawi. Malar. J. 17 , 147 (2018).
Tagbor, H. et al. Seasonal malaria chemoprevention in an area of extended seasonal transmission in Ashanti, Ghana: an individually randomised clinical trial. Trop. Med. Int. Health 21 , 224–235 (2016).
McCann, R. S., Cohee, L. M., Goupeyou-Youmsi, J. & Laufer, M. K. Maximizing impact: can interventions to prevent clinical malaria reduce parasite transmission? Trends Parasitol. 36 , 906–913 (2020).
World Health Organization. Global Technical Strategy for Malaria 2016–2030 (World Health Organization, 2015).
Binka, F. N., Indome, F. & Smith, T. Impact of spatial distribution of permethrin-impregnated bed nets on child mortality in rural northern Ghana. Am. J. Trop. Med. Hyg. 59 , 80–85 (1998).
Hawley, W. A. et al. Community-wide effects of permethrin-treated bed nets on child mortality and malaria morbidity in western Kenya. Am. J. Trop. Med. Hyg. 68 , 121–127 (2003).
Howard, S. C. et al. Evidence for a mass community effect of insecticide-treated bednets on the incidence of malaria on the Kenyan coast. Trans. R. Soc. Trop. Med. Hyg. 94 , 357–360 (2000).
Conteh, L. et al. Costs and cost-effectiveness of malaria control interventions: a systematic literature review. Value Health 24 , 1213–1222 (2021).
Winskill, P., Walker, P. G., Cibulskis, R. E. & Ghani, A. C. Prioritizing the scale-up of interventions for malaria control and elimination. Malar. J. 18 , 122 (2019).
SMC Alliance. SMC Impact project in the Gambia. SMC, https://www.smc-alliance.org/smc-impact-project-in-the-gambia (2021).
Stresman, G. H. et al. Do hotspots fuel malaria transmission: a village-scale spatio-temporal analysis of a 2-year cohort study in The Gambia. BMC Med 16 , 160 (2018).
Stresman, G. H. et al. Focal screening to identify the subpatent parasite reservoir in an area of low and heterogeneous transmission in the Kenya highlands. J. Infect. Dis. 212 , 1768–1777 (2015).
London School of Hygiene and Tropical Medicine. P. Falciparum infection dynamics and transmission to inform elimination. https://clinicaltrials.gov/ct2/show/NCT04053907 (2022).
Mwesigwa, J. et al. On-going malaria transmission in The Gambia despite high coverage of control interventions: a nationwide cross-sectional survey. Malar. J. 14 , 314 (2015).
Kirby, M. J. et al. Risk factors for house-entry by malaria vectors in a rural town and satellite villages in The Gambia. Malar. J. 7 , 2 (2008).
Caputo, B. et al. Anopheles gambiae complex along The Gambia River, with particular reference to the molecular forms of An. gambiae s.s. Malar. J. 7 , 182 (2008).
ACCESS-SMC Partnership Effectiveness of seasonal malaria chemoprevention at scale in west and central Africa: an observational study. Lancet Lond. Engl. 396 , 1829–1840 (2020).
Boerma, T., AbouZahr, C., Evans, D. & Evans, T. Monitoring intervention coverage in the context of universal health coverage. PLoS Med. 11 , e1001728 (2014).
Requejo, J. et al. Assessing coverage of interventions for reproductive, maternal, newborn, child, and adolescent health and nutrition. BMJ 368 , l6915 (2020).
Hayes, R. & Moulton, L. Cluster Randomised Trials (Chapman and Hall/CRC, 2021).
Eldridge, S. & Kelly, S. Trial reporting. in A Practical Guide to Cluster Randomised Trials in Health Services Research 218–265 (John Wiley & Sons, Ltd, 2012). https://doi.org/10.1002/9781119966241.ch10 .
Richardson, S. et al. Modeled impact of seasonal malaria chemoprevention on district-level suspected and confirmed malaria cases in chad based on routine clinical data (2013–2018). Am. J. Trop. Med. Hyg. 105 , 1712–1721 (2021).
Article CAS PubMed PubMed Central Google Scholar
Diawara, F. et al. Measuring the impact of seasonal malaria chemoprevention as part of routine malaria control in Kita, Mali. Malar. J. 16 , 325 (2017).
Bousema, T. et al. Hitting hotspots: spatial targeting of malaria for control and elimination. PLoS Med 9 , e1001165 (2012).
Peatey, C. L. et al. Effect of antimalarial drugs on plasmodium falciparum gametocytes. J. Infect. Dis. 200 , 1518–1521 (2009).
Portugaliza, H. P., Llorà-Batlle, O., Rosanas-Urgell, A. & Cortés, A. Reporter lines based on the gexp02 promoter enable early quantification of sexual conversion rates in the malaria parasite Plasmodium falciparum. Sci. Rep. 9 , 14595 (2019).
Puta, C. & Manyando, C. Enhanced gametocyte production in Fansidar-treated Plasmodium falciparum malaria patients: implications for malaria transmission control programmes. Trop. Med. Int. Health 2 , 227–229 (1997).
Thommen, B. T. et al. Revisiting the effect of pharmaceuticals on transmission stage formation in the malaria parasite Plasmodium falciparum. Front. Cell. Infect. Microbiol . 12 , 802341 (2022).
Villa, M., Buysse, M., Berthomieu, A. & Rivero, A. The transmission-blocking effects antimalarial drugs revisited: mosquito fitness costs and sporontocidal effects of artesunate and sulfadoxine-pyrimethamine. Int. J. Parasitol. 51 , 279–289, https://doi.org/10.1101/2020.05.19.103408 (2021).
White, N. J. Malaria. in Manson’s Tropical Infectious Diseases, 23rd edn, 532–600.e1 (W.B. Saunders, 2014). https://doi.org/10.1016/B978-0-7020-5101-2.00044-3 .
Ahmad, A. et al. Gametocyte carriage after seasonal malaria chemoprevention in Plasmodium falciparum infected asymptomatic children. Malar. J. 20 , 169 (2021).
Barnes, K. I. et al. Increased gametocytemia after treatment: an early parasitological indicator of emerging sulfadoxine-pyrimethamine resistance in falciparum malaria. J. Infect. Dis. 197 , 1605–1613 (2008).
Bousema, T. & Drakeley, C. Epidemiology and Infectivity of Plasmodium falciparum and Plasmodium vivax gametocytes in relation to malaria control and elimination. Clin. Microbiol. Rev. 24 , 377–410 (2011).
Collins, K. A. et al. A cohort study on the duration of Plasmodium falciparum infections during the dry season in The Gambia. J. Infect. Dis. 226 , 128–137 (2022).
Download references
Acknowledgements
We would like to acknowledge the contribution of all the fieldworkers and participants of the 10 Upper River Region communities who provided data for this study; Isabel Byrne for advice on the spatial analysis approach, and Professor Paul Milligan for information on the status of extended-age SMC pilots in West Africa. This study was funded via a grant to C.D. and T.B. from the Bill and Melinda Gates Foundation (grant ref: OPP1173572). The Foundation did not provide any input to any aspect of the design of the study or development of this manuscript.
Author information
Authors and affiliations.
Department of Infection Biology, London School of Hygiene & Tropical Medicine, Keppel Street, London, UK
Seyi Soremekun, Marta Moreno & Chris Drakeley
Medical Research Council Unit The Gambia at the London School of Hygiene & Tropical Medicine, Banjul, The Gambia
Bakary Conteh, Abdoullah Nyassi, Harouna M. Soumare, Blessed Etoketim, Mamadou Ousmane Ndiath, Umberto D’Alessandro & Annette Erhart
Medical Research Council International Statistics and Epidemiology Group, London School of Hygiene & Tropical Medicine, Keppel Street, London, UK
John Bradley
Department of Medical Microbiology, Radboud University Medical Center, Nijmegen, The Netherlands
Teun Bousema
You can also search for this author in PubMed Google Scholar
Contributions
S.S., B.C., U.D.A., T.B., A.E., M.M. and C.D.—responsible for study concept and design. B.C., M.M., A.E., T.B., C.D.—responsible for fieldwork coordination. A.N., S.S., M.M.—responsible for data management. H.M.S.—responsible for entomological surveillance data collection. B.E., M.O.N.—responsible for laboratory assays. S.S., J.B.—responsible for statistical analysis. S.S. wrote the first draft of the paper and all authors reviewed and approved the manuscript.
Corresponding authors
Correspondence to Seyi Soremekun or Chris Drakeley .
Ethics declarations
Competing interests.
The authors declare no competing interests.
Peer review
Peer review information.
Communications Medicine thanks Pfupajena Machingauta and the other, anonymous, reviewer(s) for their contribution to the peer review of this work. A peer review file is available.
Additional information
Publisher’s note Springer Nature remains neutral with regard to jurisdictional claims in published maps and institutional affiliations.
Supplementary information
Peer review file, supplementary file, description of additional supplementary files, supplementary data 1 and 2, supplementary data 3, reporting summary, rights and permissions.
Open Access This article is licensed under a Creative Commons Attribution 4.0 International License, which permits use, sharing, adaptation, distribution and reproduction in any medium or format, as long as you give appropriate credit to the original author(s) and the source, provide a link to the Creative Commons licence, and indicate if changes were made. The images or other third party material in this article are included in the article’s Creative Commons licence, unless indicated otherwise in a credit line to the material. If material is not included in the article’s Creative Commons licence and your intended use is not permitted by statutory regulation or exceeds the permitted use, you will need to obtain permission directly from the copyright holder. To view a copy of this licence, visit http://creativecommons.org/licenses/by/4.0/ .
Reprints and permissions
About this article
Cite this article.
Soremekun, S., Conteh, B., Nyassi, A. et al. Household-level effects of seasonal malaria chemoprevention in the Gambia. Commun Med 4 , 97 (2024). https://doi.org/10.1038/s43856-024-00503-0
Download citation
Received : 08 August 2023
Accepted : 18 April 2024
Published : 22 May 2024
DOI : https://doi.org/10.1038/s43856-024-00503-0
Share this article
Anyone you share the following link with will be able to read this content:
Sorry, a shareable link is not currently available for this article.
Provided by the Springer Nature SharedIt content-sharing initiative
Quick links
- Explore articles by subject
- Guide to authors
- Editorial policies
Sign up for the Nature Briefing newsletter — what matters in science, free to your inbox daily.

Select Your Interests
Customize your JAMA Network experience by selecting one or more topics from the list below.
- Academic Medicine
- Acid Base, Electrolytes, Fluids
- Allergy and Clinical Immunology
- American Indian or Alaska Natives
- Anesthesiology
- Anticoagulation
- Art and Images in Psychiatry
- Artificial Intelligence
- Assisted Reproduction
- Bleeding and Transfusion
- Caring for the Critically Ill Patient
- Challenges in Clinical Electrocardiography
- Climate and Health
- Climate Change
- Clinical Challenge
- Clinical Decision Support
- Clinical Implications of Basic Neuroscience
- Clinical Pharmacy and Pharmacology
- Complementary and Alternative Medicine
- Consensus Statements
- Coronavirus (COVID-19)
- Critical Care Medicine
- Cultural Competency
- Dental Medicine
- Dermatology
- Diabetes and Endocrinology
- Diagnostic Test Interpretation
- Drug Development
- Electronic Health Records
- Emergency Medicine
- End of Life, Hospice, Palliative Care
- Environmental Health
- Equity, Diversity, and Inclusion
- Facial Plastic Surgery
- Gastroenterology and Hepatology
- Genetics and Genomics
- Genomics and Precision Health
- Global Health
- Guide to Statistics and Methods
- Hair Disorders
- Health Care Delivery Models
- Health Care Economics, Insurance, Payment
- Health Care Quality
- Health Care Reform
- Health Care Safety
- Health Care Workforce
- Health Disparities
- Health Inequities
- Health Policy
- Health Systems Science
- History of Medicine
- Hypertension
- Images in Neurology
- Implementation Science
- Infectious Diseases
- Innovations in Health Care Delivery
- JAMA Infographic
- Law and Medicine
- Leading Change
- Less is More
- LGBTQIA Medicine
- Lifestyle Behaviors
- Medical Coding
- Medical Devices and Equipment
- Medical Education
- Medical Education and Training
- Medical Journals and Publishing
- Mobile Health and Telemedicine
- Narrative Medicine
- Neuroscience and Psychiatry
- Notable Notes
- Nutrition, Obesity, Exercise
- Obstetrics and Gynecology
- Occupational Health
- Ophthalmology
- Orthopedics
- Otolaryngology
- Pain Medicine
- Palliative Care
- Pathology and Laboratory Medicine
- Patient Care
- Patient Information
- Performance Improvement
- Performance Measures
- Perioperative Care and Consultation
- Pharmacoeconomics
- Pharmacoepidemiology
- Pharmacogenetics
- Pharmacy and Clinical Pharmacology
- Physical Medicine and Rehabilitation
- Physical Therapy
- Physician Leadership
- Population Health
- Primary Care
- Professional Well-being
- Professionalism
- Psychiatry and Behavioral Health
- Public Health
- Pulmonary Medicine
- Regulatory Agencies
- Reproductive Health
- Research, Methods, Statistics
- Resuscitation
- Rheumatology
- Risk Management
- Scientific Discovery and the Future of Medicine
- Shared Decision Making and Communication
- Sleep Medicine
- Sports Medicine
- Stem Cell Transplantation
- Substance Use and Addiction Medicine
- Surgical Innovation
- Surgical Pearls
- Teachable Moment
- Technology and Finance
- The Art of JAMA
- The Arts and Medicine
- The Rational Clinical Examination
- Tobacco and e-Cigarettes
- Translational Medicine
- Trauma and Injury
- Treatment Adherence
- Ultrasonography
- Users' Guide to the Medical Literature
- Vaccination
- Venous Thromboembolism
- Veterans Health
- Women's Health
- Workflow and Process
- Wound Care, Infection, Healing
- Download PDF
- Share X Facebook Email LinkedIn
- Permissions
Monoclonal Antibody Protected Majority of Children From Malaria
Current strategies to protect children from malaria—administration of new RTS,S/AS01 or R21/Matrix-M vaccines as well as seasonal medications—require about 20 health care visits by the time a child reaches age 5 years. However, an approach involving the annual administration of a monoclonal antibody might provide similar malaria protection while reducing health care visits, a randomized clinical trial found.
A subcutaneous dose of the monoclonal antibody, known as L9LS, was 70% effective against infection with the Plasmodium falciparum parasite compared with placebo over the 6-month malaria season, according to results from 225 children aged 6 to 10 years in Mali. The treatment was also 77% effective against clinical malaria, defined as symptoms including fever and a large number of parasites in the blood, the researchers reported in the New England Journal of Medicine .
Because factors such as nutritional status and coinfections as well as accessibility could influence the outcomes of people who receive L9LS, studies illustrating real-world value and cost-effectiveness would support the continued development of monoclonal antibodies for treating malaria, the authors of a linked editorial noted.
Published Online: May 24, 2024. doi:10.1001/jama.2024.8636
See More About
Harris E. Monoclonal Antibody Protected Majority of Children From Malaria. JAMA. Published online May 24, 2024. doi:10.1001/jama.2024.8636
Manage citations:
© 2024
Artificial Intelligence Resource Center
Cardiology in JAMA : Read the Latest
Browse and subscribe to JAMA Network podcasts!
Others Also Liked
- Register for email alerts with links to free full-text articles
- Access PDFs of free articles
- Manage your interests
- Save searches and receive search alerts
Volume 30, Number 6—June 2024
Research Letter
Foodborne disease outbreaks linked to foods eligible for irradiation, united states, 2009–2020.

Cite This Article
Food irradiation can reduce foodborne illnesses but is rarely used in the United States. We determined whether outbreaks related to Campylobacter , Salmonella , Escherichia coli , and Listeria monocytogenes were linked to irradiation-eligible foods. Of 482 outbreaks, 155 (32.2%) were linked to an irradiation-eligible food, none of which were known to be irradiated.
Food irradiation has been studied globally for decades and is a safe, effective means of reducing foodborne illness–causing pathogens, sterilizing insects, delaying ripening or sprouting, and extending shelf life ( 1 , 2 ). The US Food and Drug Administration has approved various foods for irradiation, including meat, poultry, fresh shell eggs, and spices ( 2 ) ( Appendix Table). However, irradiation has not been widely adopted in the United States because of large fixed costs and the perception of consumer unwillingness to purchase irradiated food ( 3 ). Estimates of the amount of irradiated food available in the United States are scarce, but as of 2010, approximately one third of spices consumed and <0.1% of imported fruit, vegetables, and meats were irradiated ( 3 ).
Campylobacter , Salmonella , Escherichia coli , and Listeria monocytogenes are among the most common bacterial foodborne pathogens causing illnesses, hospitalizations, and death in the United States ( 4 ) and can be neutralized by irradiation at sufficient doses ( 5 ). We identified outbreaks caused by these pathogens and linked to irradiation-eligible foods; then, we determined whether any of the foods had been irradiated.
In the United States, the Foodborne Disease Outbreak Surveillance System (FDOSS) collects information from state, local, and territorial health departments about foodborne disease outbreaks. The National Outbreak Reporting System, launched in 2009, reports information gathered by FDOSS, including food processing methods such as shredding, pasteurizing, or irradiation. We searched for foodborne disease outbreaks reported and finalized through FDOSS and the National Outbreak Reporting System as of February 4, 2022, for which the date of first illness onset occurred during 2009–2020 and a confirmed pathogen was Campylobacter , Salmonella , E. coli , or Listeria monocytogenes. A foodborne disease outbreak was defined as > 2 illnesses linked to a common exposure with evidence suggesting a food source. FDOSS variables we examined included method of processing, food vehicle, Interagency Food Safety Analytics Collaboration (IFSAC) food category, and the number of estimated primary illnesses, hospitalizations, and deaths. We grouped outbreaks by IFSAC category and irradiation approval status (eligible, some foods eligible, not yet eligible, or undetermined) ( Appendix Table). We conducted a literature review to identify outbreaks not captured through FDOSS. We obtained foods approved for irradiation for pathogen reduction and approval years from the Code of Federal Regulations 21 Part 179 ( Appendix Table).
In FDOSS, we identified 2,153 foodborne outbreaks during 2009–2020 caused by Campylobacter , Salmonella , E. coli , or Listeria monocytogenes . Of those, 482 (22.4%) included information regarding processing methods other than unknown or a missing value; none had irradiation listed as a processing method. Of the 482 outbreaks, 155 (32.2%) were linked to a food eligible for irradiation when the onset of the first reported illness occurred; those outbreaks resulted in 3,512 illnesses, 463 hospitalizations, and 10 deaths ( Appendix Table). The most common sources were chicken (52 outbreaks), beef (31), and eggs (29), comprising 72% (112/155) of outbreaks linked to irradiation-eligible foods.
During our literature search, we identified 1 outbreak linked to food that might have included an irradiated ingredient. During 2009–2010, Salmonella enterica serotype Montevideo was found in imported pepper used in ready-to-eat salami ( 6 ). Some of the manufacturer’s pepper was reportedly irradiated, but some was not. Whether the implicated product contained irradiated pepper is unclear. Irradiation was not reported as a processing method for the outbreak in FDOSS. After consultation with the Centers for Disease Control and Prevention outbreak investigation team, we determined there was insufficient evidence to link that outbreak to irradiated pepper.
The illnesses, hospitalizations, and deaths associated with outbreaks linked to irradiation-eligible foods might have been prevented or reduced had these foods been irradiated. Irradiation has repeatedly been proposed as a strategy to reduce foodborne disease outbreaks ( 5 , 7 , 8 ). Irradiation typically eliminates a large proportion of pathogenic microorganisms. The efficacy of irradiation depends on factors like temperature and water content ( 9 ). Food may become contaminated after irradiation. Irradiation can be a useful tool in improving food safety complementary to existing food safety practices. Consumer demand for irradiated foods may be increased through education ( 10 ).
The first limitation of our study is that IFSAC food categories do not always correspond to food groups approved for irradiation by the US Food and Drug Administration ( Appendix Table); therefore, misclassification of irradiation approval status might have occurred for some foods. Reporting of outbreaks to FDOSS is voluntary, and processing method information was frequently missing, so irradiation might have been underreported or unrecognized by public health partners because of limited knowledge of irradiation or unfamiliarity with labeling. For outbreaks with multiple etiologies including a pathogen other than the 4 of interest, irradiation might not have reduced those pathogens.
We identified 155 Campylobacter , Salmonella , E. coli , or Listeria monocytogenes outbreaks with a known method of processing that were linked to irradiation-eligible foods during 2009–2020; none of the implicated foods were reported as irradiated. These results suggest that some outbreaks could be prevented or mitigated through irradiation. Prioritizing food irradiation efforts, particularly for chicken, beef, and eggs, could substantially reduce outbreaks and illnesses.
Dr. Zlotnick is an Oak Ridge Institute for Science and Education Fellow at the Centers for Disease Control and Prevention, Atlanta. Her work focuses on the prevention of zoonotic disease originating in food or animal contact.
Acknowledgments
We thank Michelle Canning, Laura Gieraltowski, Lane Highbarger, and Jacqueline Roshelli Baker for their assistance.
This project was supported in part by an appointment to the Research Participation Program at the Centers for Disease Control and Prevention administered by the Oak Ridge Institute for Science and Education through an interagency agreement between the US Department of Energy and the Centers for Disease Control and Prevention.
- High-dose irradiation: wholesomeness of food irradiated with doses above 10 kGy. Report of a Joint FAO/IAEA/WHO Study Group. World Health Organ Tech Rep Ser . 1999 ; 890 : i – vi, 1–197 . PubMed Google Scholar
- Food and Drug Administration . Food irradiation: what you need to know. 2022 [ cited 2022 Jun 23 ]. https://www.fda.gov/food/buy-store-serve-safe-food/food-irradiation-what-you-need-know
- Ferrier P . Irradiation of produce imports: small inroads, big obstacles. 2011 [ cited 2022 June 27 ]. https://www.ers.usda.gov/amber-waves/2011/june/irradiation-of-produce-imports
- Scallan E , Hoekstra RM , Angulo FJ , Tauxe RV , Widdowson MA , Roy SL , et al. Foodborne illness acquired in the United States—major pathogens. Emerg Infect Dis . 2011 ; 17 : 7 – 15 . DOI PubMed Google Scholar
- Tauxe RV . Food safety and irradiation: protecting the public from foodborne infections. Emerg Infect Dis . 2001 ; 7 ( Suppl ): 516 – 21 . DOI PubMed Google Scholar
- Gieraltowski L , Julian E , Pringle J , Macdonald K , Quilliam D , Marsden-Haug N , et al. Nationwide outbreak of Salmonella Montevideo infections associated with contaminated imported black and red pepper: warehouse membership cards provide critical clues to identify the source. Epidemiol Infect . 2013 ; 141 : 1244 – 52 . DOI PubMed Google Scholar
- Osterholm MT , Norgan AP . The role of irradiation in food safety. N Engl J Med . 2004 ; 350 : 1898 – 901 . DOI PubMed Google Scholar
- Gunther NW IV , Abdul-Wakeel A , Scullen OJ , Sommers C . The evaluation of gamma irradiation and cold storage for the reduction of Campylobacter jejuni in chicken livers. Food Microbiol . 2019 ; 82 : 249 – 53 . DOI PubMed Google Scholar
- World Health Organization . Safety and nutritional adequacy of irradiated food. Geneva: World Health Organization; 1994 [ cited 2022 Nov 16 ]. https://iris.who.int/handle/10665/39463
- Ablan M , Low MSF , Marshall KE , Devchand R , Koehler L , Hume H , et al. Focus groups exploring U.S. adults’ knowledge, attitudes, and practices related to irradiation as a food safety intervention, 2021. Food Prot Trends . 2023 ; 43 : 448 – 56 .
DOI: 10.3201/eid3006.230922
Table of Contents – Volume 30, Number 6—June 2024
Please use the form below to submit correspondence to the authors or contact them at the following address:
Marta Zlotnick, Centers for Disease Control and Prevention, 1600 Clifton Rd NE, Mailstop H24-11, Atlanta, GA 30329-4018, USA
Comment submitted successfully, thank you for your feedback.
There was an unexpected error. Message not sent.
Exit Notification / Disclaimer Policy
- The Centers for Disease Control and Prevention (CDC) cannot attest to the accuracy of a non-federal website.
- Linking to a non-federal website does not constitute an endorsement by CDC or any of its employees of the sponsors or the information and products presented on the website.
- You will be subject to the destination website's privacy policy when you follow the link.
- CDC is not responsible for Section 508 compliance (accessibility) on other federal or private website.
Article Citations
Highlight and copy the desired format.
Metric Details
Article views: 653.
Data is collected weekly and does not include downloads and attachments. View data is from .
What is the Altmetric Attention Score?
The Altmetric Attention Score for a research output provides an indicator of the amount of attention that it has received. The score is derived from an automated algorithm, and represents a weighted count of the amount of attention Altmetric picked up for a research output.

An official website of the United States government
The .gov means it’s official. Federal government websites often end in .gov or .mil. Before sharing sensitive information, make sure you’re on a federal government site.
The site is secure. The https:// ensures that you are connecting to the official website and that any information you provide is encrypted and transmitted securely.
- Publications
- Account settings
Preview improvements coming to the PMC website in October 2024. Learn More or Try it out now .
- Advanced Search
- Journal List
- HHS Author Manuscripts

An Overview of Malaria in Pregnancy
Melissa bauserman.
1 Department of Pediatrics, University of North Carolina School of Medicine, Chapel Hill, NC
Andrea L Conroy
2 Department of Pediatrics, Indiana University School of Medicine, Indianapolis, IN
Krysten North
3 Department of Pediatrics, University of North Carolina School of Medicine, Chapel Hill, NC
Jackie Patterson
4 Department of Pediatrics, University of North Carolina School of Medicine, Chapel Hill, NC
5 Department of Pediatrics, University of North Carolina School of Medicine, Chapel Hill, NC
Steve Meshnick
6 Department of Epidemiology, University of North Carolina Gilligns School of Global Public Health, Chapel Hill, NC
One hundred twenty-five million pregnant women are at risk for contracting malaria, a preventable cause of maternal and infant morbidity and death. Malaria parasites contribute to adverse pregnancy and birth outcomes due to their preferential accumulation in placental intervillous spaces. Pregnant women are particularly vulnerable to malaria infections, and malaria infections during pregnancy put their fetuses at risk. Malaria in pregnancy is associated with anemia, stillbirth, low birth weight and maternal and fetal death. We review the challenges to diagnosing malaria in pregnancy, as well as strategies to prevent and treat malaria in pregnancy. Finally, we discuss the current gaps in knowledge and potential areas for continued research.
Introduction
Globally, an estimated 125 million pregnant women reside in areas where they are at risk of contracting malaria in pregnancy (MIP), and MIP remains an important preventable cause of adverse birth outcomes. 1 Although there are five species of malaria that infect humans, two main species of Plasmodium contribute to adverse maternal and fetal outcomes in pregnancy, P. falciparum and P. vivax. In sub-Saharan Africa, where the majority of adverse birth outcomes attributable to malaria occur, P. falciparum is the dominant species. However, over half of pregnancies that are potentially exposed to malaria occur in Southeast Asia and the Western Pacific where P. falciparum and P. vivax coexist. Co-existence of P. falciparum and P. vivax also occurs in South America, where 3% of the global total of women at risk for MIP reside. 1
Over the past decade, there has been significant progress in reducing the global prevalence of P. falciparum , particularly in Africa. However, women remain at high risk of MIP, with over 50% of women in high transmission areas having P. falciparum detected in peripheral blood at presentation to antenatal care. 2 , 3 This high prevalence of disease results from an increased risk of contracting malaria among pregnant compared to non-pregnant women. Women who are younger, malnourished, primigravidae/secundigravidae, lack immunity to pregnancy-associated malaria, or living with HIV are at the highest risk of malaria-associated adverse pregnancy outcomes. 4 , 5
Pathophysiology of Malaria in Pregnancy
MIP contributes to adverse pregnancy outcomes, at least in part, due to the preferential accumulation of parasites in the placental intervillous space. Placental sequestration is common in infections with P. falciparum because malaria parasites export a protein, VAR2CSA, to the red blood cell membrane that facilitates adherence to chondroitin-sulfate A (CSA) on syndecan-1, which is anchored in placental tissue. 6 This interaction is associated with the recruitment, retention and activation of mononuclear cells in the placenta—and is thought to mediate malaria’s effect on birth outcomes. 7 Maternal antibodies against VAR2CSA are protective. 5 P. vivax can also lead to placental changes, but to date no studies have unequivocally documented the sequestration of P. vivax infected erythrocytes in the placenta. 8
A number of histological changes in P. falciparum infected placentae have been described, including the infiltration of mononuclear cells, deposition of malaria pigment, thickening of the trophoblast basement membrane, syncytial knotting, and complement deposition. 9 – 11 Inflammation in the placenta has been linked to impaired transplacental transport of glucose 12 and amino acids, 13 and disruption of the insulin-like growth hormone axis. 14 Impaired nutrient transport across the placenta may be further exacerbated by altered placental angiogenesis 15 leading to changes in both the villous architecture 16 and surface area for nutrient exchange, as well as impaired uteroplacental blood flow. 15 , 17 These histologic and functional changes likely contribute to impaired fetal growth. Longitudinal Doppler data support the idea that malaria in the first half of pregnancy can lead to changes in umbilical artery blood flow and fetal growth in pregnancy, and this is affected by both gravidity and nutritional status. 18
Risk Factors for Malaria in Pregnancy
Environmental, parasite, and maternal factors influence the severity of MIP. In areas where malaria transmission is high, the primary burden of malaria is in primigravidae, whereas, in areas of low transmission, all gravidities are at risk. In areas of high transmission, primigravidae develop antibodies to VAR2CSA protein produced by malaria parasites, and are partially protected during subsequent pregnancies; this tends not to happen in areas of low transmission. 19 Overall, pregnant women living in areas with low or unstable (episodic) transmission have little or no immunity to malaria and are at a two-to-three times higher risk of severe disease compared to non-pregnant controls. 20 P. falciparum has typically been associated with more severe MIP than P. vivax , although P. vivax is more likely to occur in a mother with little acquired immunity. 21 Maternal age and gravidity also play a role in the severity of MIP. Younger mothers are at greater risk for severe malaria infection compared to older mothers, who appear to have some protection from severe disease. 4 In high transmission areas, such as sub-Saharan Africa, primigravidae and secundigravidae are at greater risk for severe malaria infection compared to multigravidae, but this is not true in areas of low transmission, where multigravidae have not had prior malaria exposure nor developed immunity. 22
Malnourished pregnant women are at increased risk for adverse birth outcomes with MIP. 23 A meta-analysis using individual patient data from 14,633 pregnancies from Africa and the Western Pacific between 1996–2015 showed that malaria and malnutrition are common exposures, with 35% of women having either of those exposures. Pregnant women with malnutrition and malaria were at an increased risk of LBW compared to women with only 1 of those risk factors. 24 Recent data suggests reduced L-arginine intake is one mechanism through which malnutrition contributes to low birth weight with both nutritional survey data 25 and preclinical models 26 suggesting that L-arginine supplementation may reduce preterm birth 25 and increase fetal viability, placental vascularization, and birth weight. 26
Burden of Malaria
Maternal effects.
The clinical effects of malaria on pregnant women vary from no symptoms to severe anemia and death. Women living in areas of low malaria transmission who have a lower degree of acquired immunity are more likely to experience complications such as renal failure, pulmonary edema, and cerebral malaria. 27 Despite this, the overall maternal mortality rate is similar in low-transmission areas (0.6–12.5%) compared to malaria-endemic areas (0.5–23%). 4 More research is warranted on the topic of malaria-related mortality during pregnancy, as the current data are limited and inconsistent.
Maternal anemia is one of the most common symptoms of MIP. Plasmodium causes anemia through hemolysis, increased splenic clearance of erythrocytes, and reduced red blood cell production. While severe anemia during pregnancy (hemoglobin <7 g/dL) is often multifactorial with significant nutritional components, malaria can play an important role. 28 In one estimate in sub-SaharanAfrica, the population attributable fraction of malaria to severe anemia during pregnancy was 26%. 4 For endemic areas with a 5% baseline prevalence of severe anemia, epidemiological modeling predicts malaria-induced anemia to contribute to nine maternal deaths per 100,000 live births. 4 However, in a population-based study in the Democratic Republic of the Congo, malaria played little or no role as a driver of anemia during pregnancy. 29
Pregnant women are three times more likely to be affected by severe malaria. 30 The World Health Organization defines severe malaria as parasitemia with evidence of end organ dysfunction ( Table 1 ). The presenting features of severe malaria can include severe anemia, hypoglycemia, acute respiratory distress syndrome, renal failure and cerebral malaria. 30 The median mortality of severe MIP is 39% (range 8–100%). 30 Severe malaria must be treated promptly with intensive care and parenteral antimalarial medication to reduce mortality. 31
Treatment Strategies for MIP
Fetal Effects
Malaria is an important cause of stillbirth throughout endemic areas ( Figure 1 ). MIP contributes to 12–20% of stillbirths in endemic regions of sub-Saharan Africa, with lower rates if the mother undergoes treatment. 32 P. falciparum detection both in peripheral blood samples or placental samples at delivery nearly doubles the odds of stillbirth (odds ratio 1.81 and 1.5, respectively). 32 Stillbirth risk is higher in areas with low to intermediate endemicity compared to areas of high endemicity. 33 The risk of stillbirth can be modified with appropriate malaria intervention efforts. For example, the use of insecticide-treated bed nets (ITN) is associated with lower rates of placental malaria and stillbirth (risk ratio 0.67 [95% CI 0.45 to 1.00] for stillbirth). 34

IUGR = Intrauterine growth retardation. Reprinted with permission ( 4 ).
MIP increases the risk of low birth weight (LBW), and approximately 20% of cases of LBW in malaria-endemic areas are attributed to placental infection with malaria ( Figure 1 ). 35 A mother with a malaria-infected placenta is twice as likely to have a baby with LBW. 36 LBW in turn is associated with higher infant mortality rates. In Africa, LBW has been associated with a three - to 20-fold increase in the probability of infant mortality. 36 , 37 Parity is an important factor in LBW. Primigravidae with MIP have two to seven higher odds of LBW and mortality than multigravidae. 38 The timing of infection also seems to play a role in infant size. Second-trimester infection is more likely to result in LBW than third-trimester infection; but data on first-trimester infection are limited. 39
MIP causes LBW due to both intrauterine growth restriction (IUGR) and prematurity. Up to 70% of IUGR in endemic areas is due to malaria, presumably as a result of impaired oxygen and nutrient delivery to the fetus. 35 , 36 The contribution of malaria to preterm birth is also substantial, with up to 36% of prematurity in malaria-endemic areas attributable to Plasmodium infection. Prematurity may result from the host’s immune response to malaria parasites triggering early labor. 33 These adverse fetal effects are species specific, as P. ovale and P. malariae species are not associated with adverse birth outcomes.
Congenital Malaria
Congenital malaria is defined as the identification of asexual P. falciparum parasites in the cord blood or peripheral blood of an infant during the first 7 days of life. 40 The true prevalence of congenital malaria is uncertain. While the prevalence was previously thought to be between <1% and 6%, 40 more recent studies have demonstrated a prevalence rate up to 33% in high-endemicity areas; but it is not clear how many of these congenital infections persist and cause clinical illness. 41 Most descriptive reports of congenital malaria are from infants who are born in non-endemic areas to mothers with a history of travel to endemic areas. Infant symptoms include fever associated with hepatosplenomegaly, hemolytic anemia, thrombocytopenia, and feeding intolerance. 41 These infants often become symptomatic between 10 and 30 days of life, although they can present later. Their symptoms may progress rapidly and can be fatal. 27 , 42 Because, much of the literature on congenital malaria is derived from case reports, more research is needed to better understand the epidemiology and pathophysiology of congenital malaria.
Effects in Early Childhood
Offspring are affected by placental malaria into childhood, ( Figure 1 ). Prenatal malaria exposure is associated with an increased risk of early malaria infection in children as young as four to six months of age. 40 Placental parasitemia may increase the risk of malaria infections in infancy and childhood through several mechanisms. For example, MIP might interfere with maternal antibody passage to offspring, compromising the immunity of the fetus and newborn, making the offspring vulnerable to early malaria infections. 40 In utero exposure to malaria induces the development of T reg cells that lead to fetal immune tolerance to malaria antigens that persists into childhood. 43 Placental malaria has been associated with subsequent susceptibility to non-malaria infections, including measles and tetanus, suggesting additional effects on infant immunity that may be due to the obstruction of antibody passage across the placenta. 44 Acute placental malaria infection has been associated with increased one-year mortality in infants 45 and decreased length and weight gain in the first year of life. 46 , 47 MIP is also associated with anemia during infancy and the infant’s risk for anemia with maternal peripheral parasitemia at delivery is 11.8% and 9.2% with placental malaria infection. 48
The diagnosis of MIP can be challenging due to placental sequestration of parasitized erythrocytes, low circulating levels of parasites and limited resources in malaria endemic areas for advanced diagnostic techniques. Microscopic identification of malaria from the blood by an experienced and well-equipped technician remains the gold standard for malaria diagnosis. 49 However, rapid diagnostic tests (RDTs) that test for malaria antigens, like histidine-rich protein-1 (PfHRP2), are another option for malaria diagnosis. 49 – 51 RDTs are easier than microscopy to perform in low-resource settings, because they are not dependent on highly trained technicians in well-equipped laboratories. Therefore, RDTs might be the most appropriate point of care testing among symptomatic mothers in low-resource settings. 31 , 50 However, the use of RDTs might be insufficient in diagnosing MIP among mothers with asymptomatic infection, because RDTs require a higher circulating parasite burden than microscopy for detection. 52 RDTs are also insufficient to detect low amounts of circulating parasites because of placental sequestration. In research studies, the gold standard for malaria diagnosis has been placental histopathology, but it is not practical in many field sites. 53 , 54 Using microscopy of placental blood as the referent, the sensitivity of RDTs is 81% (95% CI 55–93) and the specificity is 94% (95% CI 76–99). 31 Molecular techniques, such as polymerase chain reaction (PCR) diagnosis, have increased sensitivity of diagnosis when compared to microscopy of placental blood to 94% (95% CI 86–98), but specificity of 94% (95% CI 86–98). 31 A new generation of ultrasensitive RDT’s is now being developed and might mitigate these diagnostic problems. 55
Prevention and Treatment
Prevention strategies in africa.
In malaria-endemic areas in Africa, a combination of vector control (preventing exposure to mosquitos) and chemoprevention (preventive medication-based treatment) strategies are used to prevent MIP. The World Health Organization (WHO) recommends a combined approach using insecticide treated bed nets (ITNs) to reduce exposure to mosquitoes carrying malaria and chemoprevention. 56 ITNs work by providing a physical barrier from mosquitoes, and repelling or killing susceptible mosquitoes, which reduces mosquito density and maintains the nets’ effectiveness even after the integrity of the barrier is compromised. 57 The use of ITNs in Africa have been shown to reduce LBW by 23%, miscarriages and stillbirths by 33%, and placental parasitemia by 23%. 58 Despite the proven efficacy of ITNs, uptake was estimated at only 39% in Africa between 2009 and 2011. 59 Since that time, ITN use has steadily increased, and an estimated 61% of pregnant women at risk for malaria slept under an ITN in 2017 56 , ( Figure 2 ). Malarial resistance to pyrethroids, the most commonly used insecticide in ITNs has been reported across sub-Saharan Africa and might impair future efficacy. 57

Percentage of the population in Sub-Saharan Africa at risk for malaria with access to ITN and using ITNs.
ITN: insecticide-treated bed nets Sources: World Health Organization and Malaria Atlas Project. Reprinted with permission ( 56 ).
Spraying the walls of households with insecticide to reduce human exposure to mosquitos, a procedure known as indoor residual spraying (IRS) is part of a comprehensive vector control program. Globally, IRS usage has declined and only 3% of the population at risk was protected by IRS in 2017, which might be related to a switch in insecticide from pyrethroids to more expensive chemicals. 56 IRS has been shown to improve outcomes of MIP, with women protected by IRS having lower incidence of MIP and lower risk of placental malaria. 60 , 61 When women were protected during greater than 90% of the time of their pregnancies, women had lower risk of preterm birth (risk ratio 0.35, 95% CI, 0.15–0.84). 60 IRS is an important part of a comprehensive vector control program and might contribute to improved birth outcomes in malaria-endemic regions.
Chemoprevention strategies have been successfully used to prevent adverse health outcomes associated with MIP. The most efficacious of these strategies uses a technique of intermittent preventive treatment in pregnancy (IPTp). IPTp consists of providing monthly doses of anti-malarial medication to all pregnant women starting in the second trimester. Women who receive at least 2 courses of IPTp have a relative risk reduction of 40% for moderate to severe anemia, 61% for antenatal parasitemia, 55% for placental parasitemia and 27% for low birthweight. 62 Since 2012, the WHO has recommended monthly IPTp to reduce the incidence of these complications of pregnancy. 63 However, despite WHO recommendations, in 2017, only 22% of pregnant women received three or more doses of IPTp in Sub-Saharan Africa ( Figure 3 ). 64

Percentage of pregnant women receiving IPTp, by dose in Sub-Saharan Africa, 2010–2017.
ANC: antenatal care, IPTp: intermittent preventive treatment in pregnancy, listed by dose. Sources: National Malaria Programme, World Health Organization, US Centers for Disease Control and Prevention. Reprinted with permission ( 56 ).
Sulfadoxine-pyrimethamine (SP) has been the drug of choice for IPTp in women who are HIV-negative. 65 Plasmodium resistance to SP has emerged through multiple mutations in the P. falciparum dihydrofolate reductase (Pfdhfr) and dihydropteroate synthetase (Pfdhps), with penetrance of this haplotype in some areas of greater than 90%. 66 Due to increasingly more resistant organisms, IPTp with SP is less effective at inhibiting parasite growth and preventing fetal growth restriction. 66 In light of increasing resistance, alternative strategies for chemoprevention are being tested, including IPTp with alternative medications and strategies of intermittent screening and treatment in pregnancy (ISTp). ISTp strategies use RDTs to diagnosis MIP at multiple time points and treat only when RDTs are positive. However, these strategies depend on accurate diagnosis of MIP and have not been proven to be effective alternatives to IPTp-SP even in highly resistant areas. 66 IPTp with dihydroartemisinin-piperaquine (DP) might be a promising alternative to IPTp-SP, but more research is needed in this area. 66
Prevention Strategies Outside of Africa
In South America and Asia, where malaria transmission is typically lower than in Africa, data on traditional chemoprophylaxis is limited. Prophylaxis with mefloquine or chloroquine has been efficacious for preventing MIP in pregnant women in Thailand. 67 , 68 Other chemoprophylactic strategies employed outside of Africa have included monthly IPTp-SP with azithromycin and ISTp-SP and artesunate. These strategies show promise in the reduction of low birth weight or maternal parasitemia.
Treatment of uncomplicated malaria
All MIP infections should be treated promptly to avoid complications to the mother and fetus. 31 To ensure safety of treatment in pregnancy, the WHO recommends trimester-specific and species-specific treatment strategies for uncomplicated malaria ( Table 1 ). For first trimester treatment of P. falciparum , the WHO recommends a 7-day treatment course of quinine with clindamycin, and second line treatment includes artemisinin-based combination therapy (ACT) or oral artesunate with clindamycin. 65 First trimester treatment of uncomplicated non- falciparum malaria consists of chloroquine or quinine for chloroquine-resistant infections.
Second and third trimester treatment of uncomplicated malaria follows the same guidelines as treatment for malaria in non-pregnant adults. 31 Therefore, first line treatments with ACTs can be used in MIP. ACTs include a short-acting artemisinin component and a longer acting partner drug, such as SP. 69 The potent artemisinin reduces the number of parasites quickly and the longer acting partner drug acts on the remaining parasites and provides a post-treatment prophylactic effect, preventing new infections. 69 ACTs have achieved cure rates as high as 99.2% for uncomplicated MIP without demonstrating significant safety concerns. 70
Treatment of Severe Malaria
Severe malaria has historically been attributed to infections with P. falciparum , but more recent evidence has included P. vivax as a significant contributor to severe malaria. 30 For pregnant patients with severe malaria, the WHO recommends the same treatments for both P. falciparum and P. vivax infections. The WHO also recommends treatment with primaquine after delivery to achieve cure and prevent relapses by eradicating P. vivax sequestered in the liver, ( Table 1 ). 30
Artemisinins are the most efficacious drugs for severe MIP after the first trimester. Because they are embryotoxic and teratogenic in animal studies, the WHO does not recommend their use in the first trimester for uncomplicated malaria. However, their use is recommended even in the first trimester in cases of severe malaria because of the high risk of maternal mortality. 30 Data on the use of artemisinins in the first trimester in humans show no associated increased risk of adverse pregnancy outcomes. 30 Long-term neurodevelopmental studies are needed to evaluate the safety of different drug combinations on child development.
Quinine is an alternative to artemisinin that has been used for centuries for the treatment of malaria. Although it is not considered embryotoxic or teratogenic in animal studies, it is less well-tolerated in humans. 30 Quinine can prolong the cardiac QT interval and is associated with tinnitus, headache, blurred vision, altered auditory acuity, nausea, diarrhea, and, rarely, massive hemolysis. 65 These side effects reduce compliance with treatment regimens and lead to higher levels of treatment failure. 30
An efficacious vaccine against malaria could be of particular benefit for pregnant women. One vaccine, RTS,S, is now approved by the European Medicines Agency, but is only modestly effective. 71 Efforts are also underway to develop a vaccine targeting the VAR2CSA antigen to protect women against pregnancy-associated malaria. 72
Co-Infection with HIV
Co-infections with malaria and HIV worsen morbidity and mortality for each disease, possibly due to alterations in the balance between the immune response to malaria and stimulation of viral replication. 73 MIP is associated with a two-fold higher HIV viral load. Conversely, women living with HIV experience more placental and peripheral malaria, higher parasite densities, more frequent febrile illnesses, more severe anemia and worse birth outcomes compared to non-HIV infected mothers with MIP. 73 Chemoprevention for women living with HIV in malaria-endemic areas includes daily co-trimoxazole. SP is discouraged for chemoprevention due to potential adverse drug reactions between SP and co-trimoxazole. 74 Although pregnant women living with HIV have the greatest risk of severe MIP, treatment strategies for severe malaria in conjunction with HIV treatments have not been well studied. 30 , 73 The WHO recommends against the use of zidovidine or efavirenz and the use of artesunate amodiaquine due to neutropenia and hepatotoxicity respectively. 65 Pregnant women receiving highly active antiretroviral therapy regimens should receive quinine when cardiac monitoring is available.
Conclusions
Malaria is among the most common and easily preventable causes of poor birth outcomes in the world. IPTp and ITNs distribution programs have helped decrease malaria risk among pregnant women in many parts of the world. However, greater efforts are needed especially in light of increasing drug and insecticide resistance. A better understanding of the pathogenesis of malaria during pregnancy could lead to the development of new interventions to prevent its health consequences.
Gaps in Knowledge (sidebar)
- What are the mechanisms by which malaria during pregnancy affects fetal growth?
- What is the natural history of congenital malaria?
- How can we increase uptake of IPTp and ITN’s by pregnant women?
- When is the optimal gestational age to start IPTp?
- How can we enable women to begin IPTp earlier during pregnancy?
- When malaria prevalence drops, when is it appropriate to stop IPTp?
- When high levels of resistance to SP develop, what should be done to protect pregnant women from malaria?
- Will it be possible to vaccinate primigravidae against pregnancy-associated malaria?
DISCLOSURES
The authors report no proprietary or commercial interest in any product mentioned or concept discussed in this article.
Reference List
- Open access
- Published: 23 May 2024
The behaviour of adult Anopheles gambiae , sub-Saharan Africa’s principal malaria vector, and its relevance to malaria control: a review
- Willem Takken 1 ,
- Derek Charlwood 2 &
- Steve W. Lindsay 3
Malaria Journal volume 23 , Article number: 161 ( 2024 ) Cite this article
441 Accesses
8 Altmetric
Metrics details
Mosquitoes of the Anopheles gambiae complex are one of the major vectors of malaria in sub-Saharan Africa. Their ability to transmit this disease of major public health importance is dependent on their abundance, biting behaviour, susceptibility and their ability to survive long enough to transmit malaria parasites. A deeper understanding of this behaviour can be exploited for improving vector surveillance and malaria control.
Adult mosquitoes emerge from aquatic habitats at dusk. After a 24 h teneral period, in which the cuticle hardens and the adult matures, they may disperse at random and search upwind for a mate or to feed. Mating generally takes place at dusk in swarms that form over species-specific ‘markers’. Well-nourished females may mate before blood-feeding, but the reverse is true for poorly-nourished insects. Females are monogamous and only mate once whilst males, that only feed on nectar, swarm nightly and can potentially mate up to four times. Females are able to locate hosts by following their carbon dioxide and odour gradients. When in close proximity to the host, visual cues, temperature and relative humidity are also used. Most blood-feeding occurs at night, indoors, with mosquitoes entering houses mainly through gaps between the roof and the walls. With the exception of the first feed, females are gonotrophically concordant and a blood meal gives rise to a complete egg batch. Egg development takes two or three days depending on temperature. Gravid females leave their resting sites at dusk. They are attracted by water gradients and volatile chemicals that provide a suitable aquatic habitat in which to lay their eggs.
Whilst traditional interventions, using insecticides, target mosquitoes indoors, additional protection can be achieved using spatial repellents outdoors, attractant traps or house modifications to prevent mosquito entry. Future research on the variability of species-specific behaviour, movement of mosquitoes across the landscape, the importance of light and vision, reproductive barriers to gene flow, male mosquito behaviour and evolutionary changes in mosquito behaviour could lead to an improvement in malaria surveillance and better methods of control reducing the current over-reliance on the indoor application of insecticides.
Members of the Anopheles gambiae complex of mosquitoes have probably been responsible for more human deaths than any other animal (see Appendix), principally because they are exceptionally efficient transmitters of Plasmodium falciparum , the most lethal form of malaria [ 1 , 2 ]. This mosquito complex is a highly efficient vector of disease for four main reasons: (1) they are often highly abundant, (2) frequently bite people, (3) are highly susceptible to infection and (4) are long-lived (for a mosquito) [ 3 ]. Factors such as their propensity to enter houses to feed, the host preference and behaviour after feeding are also important. Anopheles gambiae sensu stricto ( s.s. ) , Anopheles coluzzii and Anopheles arabiensis are the three members of the complex that, with Anopheles funestus , are the primary vectors of malaria in sub-Saharan Africa.
Whilst the malaria parasite has adapted to being transmitted by these mosquitoes, the insects have adapted their ecology and behaviour to exploit humans. People unwittingly create aquatic habitats for the immature stages of the mosquito around their homes and in nearby fields, and the adult female mosquito enters houses and feeds on people at night when they are least able to defend themselves. This review describes the journey of a female An. gambiae from its emergence to locating and feeding on a human host, before eventually laying its eggs. It illustrates how a deeper understanding of these behaviours might lead to the development of novel methods of vector surveillance and control. The review is primarily intended as an introduction to the behaviour of this important vector for students and early career scientists updating earlier reviews on the subject [ 4 , 5 , 6 , 7 , 8 ].
The Anopheles gambiae complex
The Anopheles gambiae species complex consists of at least eight morphologically indistinguishable species, most of which are primarily zoophilic. Two of the most closely related members of the complex, An. gambiae and An. coluzzii, are primary malaria vectors due to their tendency to feed on humans, being long-lived, due to their resting inside traditional thatch roofed houses, and their relatively high abundance [ 9 , 10 , 11 ]. Anopheles gambiae occurs throughout much of sub-Saharan Africa whilst An. coluzzii is limited in its distribution to West Africa [ 12 ]. They are the most recently diverged members of the complex and share many behavioural traits (Fig. 1 ). Anopheles coluzzii was newly reported from East Africa for the first time suggesting that members of the complex are extending their range [ 13 ].
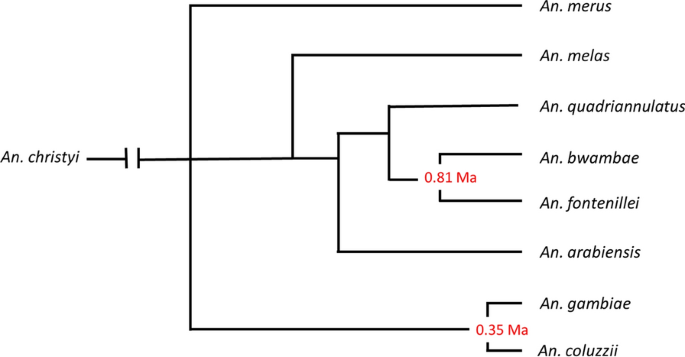
Speciation in the An. gambiae complex (source: Barron et al. [ 10 ]). Note that Anopheles amharicus , closely related to An. quadriannulatus , is not yet included in this figure. Numbers represent the divergence (Ma, million years ago) estimated based on the pairwise distances of the ML phylogeny and assuming a substation rate of 11 × 10 –9 per site, per generation and 10 generations per year [ 38 ]
Until very recently humans improved the niche occupied by malaria vectors. In addition to providing a suitable larval habitat, humans provided a suitable host and so anthropophily (when blood-feeding insects prefer to feed on human blood) developed. As a side effect of anthropophily, as people improved their housing, endophily, the habit of resting inside, evolved [ 14 ]. It was these two behaviours (anthropophily and endophily) that made An. gambiae an exceptional vector of malaria. As pointed out by Gillies and Coetzee [ 15 ] species isolation between members of the complex ‘presumably involves a spatial element’. Spatially separated swarms, where mating takes place, is the mate-recognition system that maintains isolation between members of the An. gambiae complex. It is the aspect of mating behaviour that has been most thoroughly investigated, especially by Diabate and colleagues in West Africa, reviewed in Sawadago et al . [ 16 ].
Anopheles arabiensis is also a primary malaria vector in some circumstances. It has opportunistic feeding habits and will feed on both human and animal hosts, can reach high population densities but is less long lived than the other two vectors due, perhaps, to a tendency to rest outdoors [ 17 ]. It is also more drought resistant, perhaps because of its larger size, than the other two vectors and has a greater geographical distribution [ 18 ]. Due to its tendency to both feed and rest outdoors it is less susceptible to control measures, such as insecticide treated nets or indoor resudual spraying, aimed at insects which feed or rest inside houses. In a number of instances, it has replaced An. gambiae as the most common member of the complex when such control measures have been introduced [ 17 , 19 , 20 ].
Other members of the complex ( Anopheles bwambae, Anopheles merus , Anopheles melas, Anopheles amharicus and Anopheles quadriannulatus and, the recently described, Anopheles fontenillei ) since they are limited in their distribution and are largely zoophilic, are only ever of minor importance as vectors.
Life cycle of Anopheles gambiae
As with all mosquitoes, An. gambiae exploits three habitats: an aquatic environment for early development, an aerial environment where host-seeking and mating takes place and a terrestrial environment where feeding and egg production take place. Eggs are laid singly, hatch within two to three days and pass through four larval stages in seven to 10 days, depending on water temperature, food availability and quality [ 21 , 22 ]. Larvae feed on organic material in the water, gently moving food into their mouths using bristles. This food is used for metabolic energy and build-up of energy reserves needed to survive the first few days as an adult mosquito [ 23 ]. Larval development, which is the only time when growth occurs, is followed by pupation that lasts two to three days [ 24 ]. In the laboratory, the period from laying to adult emergence takes 10–23 days [ 24 , 25 ]. In a natural environment, daily temperature variations affect the duration of the mosquitoes’ development. Perhaps because of their slighter build, males emerge a day or two before females.
Behaviours of Anopheles gambiae
Emergence and dispersal.
Adult anophelines emerge from the pupae soon after dusk [ 26 ] and wait several minutes before inflating their wings and drying out, before flying off. The dispersal flight varies according to wind strength and direction. If there is little or no wind, dispersal can be random, but if there is a strong predominant wind, mosquitoes may be blown with the wind [ 27 ]. In open savanna, 80% of An. gambiae fly less than one metre above the ground [ 28 , 29 ] with a maximum flight speed of 1.4–1.8 ms −1 [ 30 ]. Flight occurs at night and is under control of circadian rhythms [ 31 ]. Studies using flight actographs measuring the activity of individual mosquitoes show a primary peak after sunset, corresponding to the period for dispersal and oviposition, followed by secondary activity with a peak after midnight, corresponding to host location behaviour (Fig. 2 ).
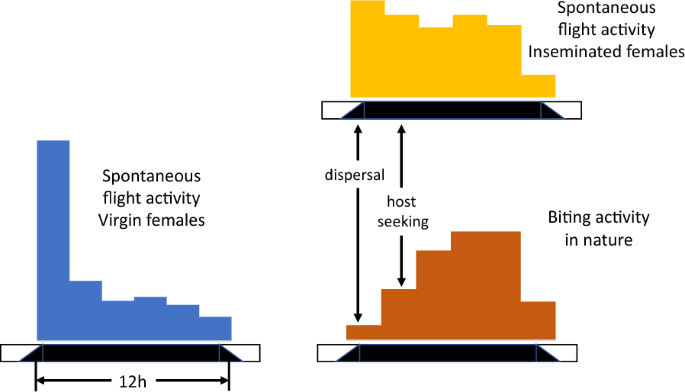
Spontaneous flight, recorded in a flight actograph, and biting activity of individual Anopheles gambiae after [ 31 ]. Source: [ 34 ]
Estimations of the distance Anopheles mosquitoes disperse is challenging given the heterogeneity in techniques used and the large variation in the ecology of sites [ 32 ]. Dispersal depends not only on the proximity, size and abundance of aquatic habitat to human habitation, but also how humans and alternative sources of blood meal are distributed in the landscape, the local vegetation and abiotic environmental conditions (incl. wind, relative humidity). The relative size and proximity of both aquatic habitats and human habitation is key. In the central part of The Gambia, where the river floods during the rainy season producing extensive pooling, An. melas may fly over 2 km from aquatic habitats to villages that are spatially clustered [ 33 ]. Support for this comes from the lack of an effect on indoor mosquito densities where large-scale larviciding was done in a 2 km area surrounding the study villages [ 34 ]. In marked contrast, in a village in western Kenya, aquatic habitats were largely human made and highly clustered within a village where houses were more widely dispersed [ 35 , 36 ], suggesting short flight distances after emergence to blood feeding. When the wind is strong (> 1.2 ms −1 ), as occurs immediately preceding a tropical storm, there may be little flight activity. Passive transportation of a few individuals moving with storm fronts at high altitude has been observed [ 37 , 38 ]. Whether mosquitoes that are lifted up and transported over long distances in this way survive their journey is open for debate. Even short suspension in cages at altitude reduced survival considerably [ 39 ]. Mosquitoes may also be transported long distances by planes, ships, trains and vehicles [ 5 ]. The introduction of An. arabiensis [ 40 ] into Brazil probably occurred by the fast mailboats from West Africa, whilst spread by vehicles was considered so important that fumigation posts were established to prevent the spread of adult mosquitoes [ 41 ].
After emergence, the behaviour of females of the complex is associated with three phases of adult life: mating, blood feeding and oviposition (Fig. 3 ). Some individuals imbibe sugar as well (see below). Newly emerged An. gambiae complex females may exhibit “pre-gravid” behaviour in which a proportion of the females require two blood meals before they can complete egg maturation [ 42 , 43 ]. It has been suggested that the first blood meal is required to build up metabolic energy reserves when females emerge undernourished from the pupa [ 44 ] These females usually also have a smaller body size compared to their well-fed siblings and may feed before mating [ 43 ].
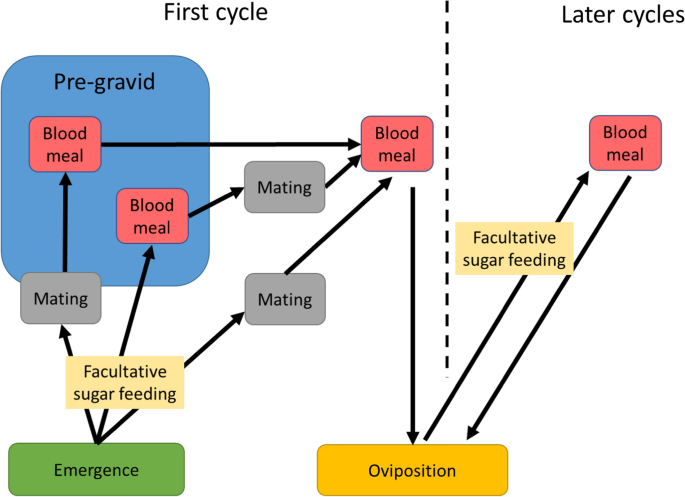
Behaviours of adult mosquitoes. Note that sugar feeding is facultative. Under natural conditions, variable proportions of recently emerged An. gambiae complex females may undergo a pre-gravid phase before completing their gonotrophic cycle. i.e. the period between one egg laying and the next. These females are often undernourished and require two bloodmeals in order to complete their first gonotrophic cycle [ 43 ]. A first bloodmeal may be necessary before mate-seeking behaviour takes place [ 44 ]. In the pre-gravid state (inside the blue box) females do not sugar feed as they derive sufficient nutriments from the first blood meal for mate location
Sugar feeding
Newly-emerged adults fly to a resting site on nearby plants, such as grasses and shrubs, where they may remain until searching for a sugar meal guided by odorant volatiles from plants to which these mosquitoes are attracted [ 45 , 46 , 47 ]. Sugar provides a source of readily available energy whilst blood, necessary for the production of eggs, can also be used for this purpose but less efficiently [ 44 , 48 ]. Sugar may be taken from honeydew of extra-floral nectaries of plants like Mangifera indica (mango), Dolonix regia (flamboyant or rural poinciana), Thevetia neriifolia (yellow oleanda), Cassia siberiana (drumstick tree), Parthenium hysterophorus (Santa Maria feverfew) and others [ 45 , 49 ]. These sugars provide metabolic energy required for flight and mating. After a single sugar meal, females generally switch to mating followed by host-seeking and blood feeding [ 50 ]. As in all biological systems, there occurs some variation in behaviour, and recent evidence suggests that female anophelines may imbibe a sugar meal, after blood feeding [ 51 , 52 ] due to the physiological condition of the female. Males continue to feed on sugar as their only source of nutrition.
Mating behaviour
As in other mosquito species, newly emerged male An. gambiae need to mature sexually before mating. During this process, their genitalia rotate 180° within a day or at most two days [ 53 ]. Females become receptive to males following a nectar feed [ 54 , 55 ], or an initial blood meal, with mating taking place in swarms [ 55 , 56 , 57 , 58 , 59 ].
Swarms are characterized by the males flying in a stationary holding pattern over, or in relation to, some aspect of the environment that is species specific. Thus, An. coluzzii swarms directly over horizontal areas of contrast [ 59 ] whilst An. gambiae tends to swarm to the side of the same markers [ 60 ] ensuring some measure of spatial separation (Fig. 4 ). This behaviour limits hybridization in areas where the two species are sympatric. Although an individual mosquito can swarm, swarms generally contain anything from tens to 100’s of insects. The size of any swarm depends to a certain extent on the mosquito population density and the size and attractiveness of the marker [ 61 , 62 ]. Virgin females respond to the same visual cues as males and in the absence of males will themselves undertake short swarming flights [ 56 , 60 ]. If a swarm is present, then the female will be mated.
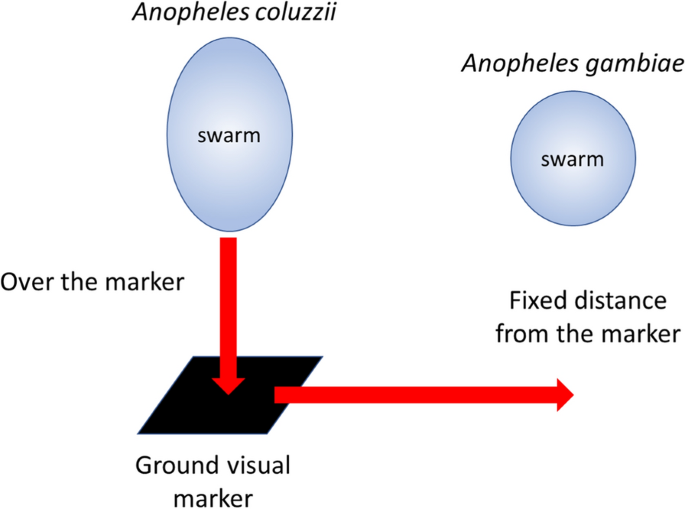
Swarming behaviour of An. gambiae . Note that swarms of An. gambiae are more spherical than An. coluzzii (after [ 60 ])
Swarms form for a limited time, circa 30 min, at dusk [ 16 ]. Males are attracted to the flight tone of the female which they hear using their antennae. Males beat their wings around 600 times a second (600 Hz), whereas females beat their wings at around 440 Hz [ 63 ]. Thus, the flight tones of males and females differ and males do not respond to the flight tone of other males but only to that of the female. They only hear the female when the fibrillae on their antennae are erect, which happens shortly before flight activity and which is under the control of a circadian clock [ 64 ]. As a result, receptivity to the female flight sound is limited to a relatively short period [ 56 , 65 ].
Swarms can sometimes involve hundred and even thousands of individuals, hence their dynamics is particularly intriguing. It has been recently shown that the dynamics of these and other animal aggregations such as of birds, fish and insects can be recreated using relatively simple mechanistic computer models that can simulate flocking in birds, shoaling in fish and swarming in insects (Langton 1996, quoted in [ 66 ]). Thus, males and females, only need to: 1) move towards the perceived centre of mass of the insects in its neighbourhood; 2) match velocities with insects in its neighbourhood; and 3) maintain a minimum distance from other objects in the environment, including other mosquitoes. When a female enters a swarm males will follow rule #2 and match speeds (and therefore their flight tone) with the female [ 67 , 68 ]. Swarm size appears not to affect mating success and it is not clear even if females select a mate [ 69 , 70 ].The timing of mating is fixed throughout the year and occurs shortly before sunset. Between two closely related species, An. gambiae and An. coluzzii , there is small difference in onset of mating, which helps to keep the reproductive isolation of the two species [ 16 ].
Mating has also been reported to occur inside houses but what distinguishes this from the usual process is not known [ 71 , 72 ]. Males can mate multiple times throughout adult life, but given the overall equal sex ratio, and female monogamy, they are unlikely to do so.
During mating, substances from the male accessory glands will form a mating plug inside the bursa copulatrix, which effectively blocks off the entrance to the spermatheca [ 73 , 74 ]. This substance contains the steroid hormone 20-hydroxyecdysone, which induces mating-refractory behaviour in the female and stimulates oviposition [ 75 ].
Host location
On approaching a host, female mosquitoes may track upwind, turning into the odour stream and moving up the concentration gradient. Field experiments indicate that mosquitoes can locate host outdoors, principally carbon dioxide, from a distance of 35 m [ 76 ].
Hosts are recognized by carbon dioxide and olfactory cues (body odours mostly), emanating from the human skin. The major attracter of longer distances is carbon dioxide [ 76 ], which is a general indicator of a mammal, but closer to the host a female will be activated by a range of volatile organic compounds (VOCs) that are produced by a multitude of microbial organisms [ 77 , 78 ]. Human odours include ammonia, l -lactic acid, tetradecanoic acid, 3-methyl-1-butanol and butan-1-amine [ 77 , 79 , 80 ]. Artificial baits, or lures, made from these VOCs, together with carbon dioxide, provide excellent ways to attract mosquitoes. These odorant cues are being recognized by selected neural receptors located on the antennae (Box 1). When stimulated by an odourant molecule, a signal will be passed through the neurons to the mosquito brain, inducing a behavioural response [ 81 , 82 , 83 ].
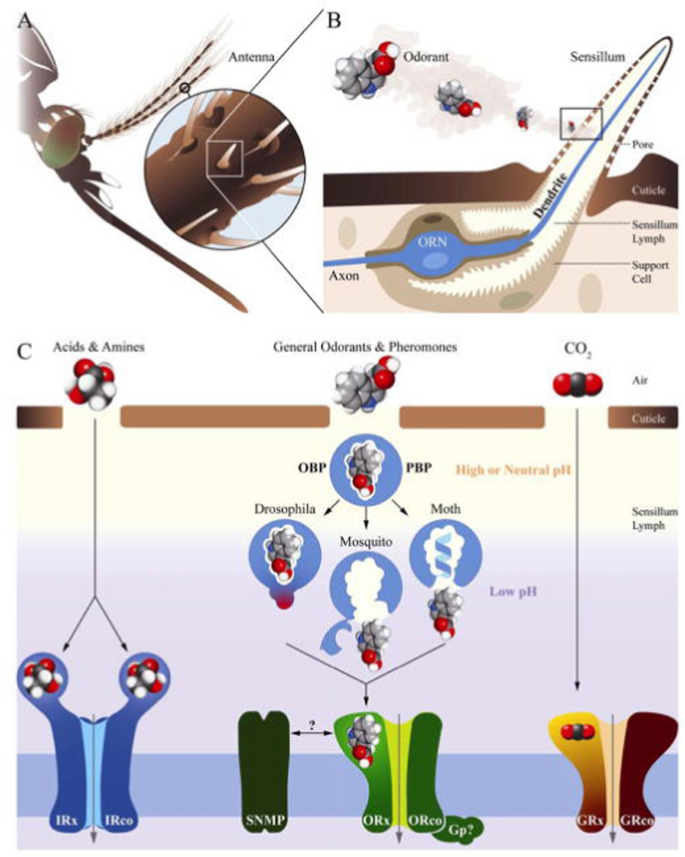
a An insect chemosensory system and molecular models in signal transduction. b An olfactory sensillum housing support cells and an olfactory neuron (blue). c Various groups of chemoreceptors being activated by different classes of odorants. Legend modified after [ 83 ]
Although underexplored, vision is also important for the orientation of mosquitoes. Based on studies on night-flying mosquitoes in the 1970s and 1980s [ 84 , 85 , 86 , 87 , 88 , 89 ], scientists showed that mosquitoes were attracted to visually conspicuous objects at a distance, although they avoided solid objects at close range. Although none of these studies involved An. gambiae , Gillies and Wilkes suggested that house-entering mosquitoes could be attracted to the shape of the house over 15–20 m, particularly if it was isolated from other houses or tall vegetation. Indoor lighting, visible from outside, can also attract An. gambiae into an experimental hut [ 89 ]. In this experiment, 84% more mosquitoes were collected in light-traps in huts with transparent walls than those with opaque walls [ 90 ]. The range of attraction of light is likely to be in the region of 5 m [ 90 , 91 ], and may vary with wavelength and intensity of light. In marked contrast, light added to the Furvela tent-trap resulted in a reduced catch compared to traps without light [ 92 ] and evidence suggests that when used outdoors CDC light-traps, whilst operationally practical, do not adequately sample the outdoor biting fraction of malaria vectors [ 93 ].
As mosquitoes approach an unprotected host, temperature and relative humidity also act as attractants increasing biting rates [ 94 , 95 ]. Feeding on a sitting host is primarily concentrated around the ankles and feet of individuals [ 96 , 97 ].
House entry
The presence of a gap between the top of the wall and the roof, or at the gable ends of a house are common features of traditional thatched-roof African houses (Fig. 5 ). It is through these gaps that host-seeking mosquitoes will enter [ 98 , 99 ]. Recent studies show that the relative attractiveness of a house increases if there is a high concentration of carbon dioxide emanating from inside [ 102 , 103 ]. As might be expected, the density of mosquitoes entering an occupied house increases with an increasing number of residents [ 102 , 103 ]. Increasing ventilation, which dilutes the carbon dioxide, will have the opposite effect reducing house entry by An. gambiae [ 100 , 101 ].
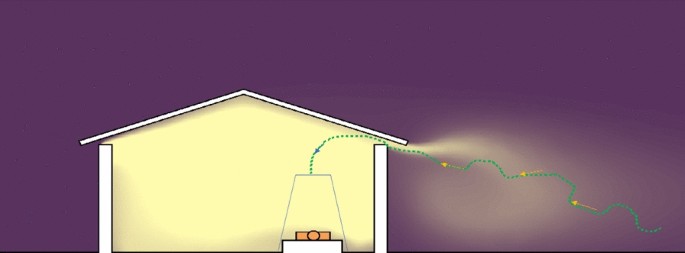
How Anopheles gambiae enters a house through the open eaves. Yellow represents human odours and the dashed line the trajectory of a blood-questing mosquito (After Spitzen et al. [ 104 ]). The human host is protected by a bed net
As a female An. gambiae approaches within several metres from a house, she will gain altitude and enter the building through the open eaves [ 104 ]. Raising a house on stilts several metres above the ground will reduce house entry of An. gambiae , probably due to reduced levels of human odour at ground level and lack of a wall to aid elevated flight [ 105 ]. In The Gambia, an experimental hut three metres above the ground housing two men had 84% fewer An. gambiae than a similar house on the ground. Nonetheless, adding netting or solid walls to an elevated hut will reduce this effect, increasing the numbers of mosquitoes entering the elevated room [ 106 ]. Similarly, in São Tomé, people living in houses built off the ground have significantly fewer An. coluzzii attacking them than people in houses at ground level [ 107 ].
Once inside the bedroom, an An. gambiae female will be attracted to hosts through volatiles produced by the occupants. Video recording shows that 75% of mosquitoes approach a human-occupied insecticide-treated bed net (ITN) from the top of the net around the torso, with a few individuals landing on the sides [ 108 ]. The behaviour of the mosquitoes was categorized into swooping, visiting, bouncing and resting. This behaviour is likely to be due to the warm odours rising from the host rising within the net as indicated using computational fluid dynamic modelling [ 109 ].
Variation in attractiveness to mosquitoes
Adult humans differ markedly in their relative attractiveness to An. gambiae , with some individuals receiving substantially more bites than others [ 110 , 111 , 112 ] with evidence that this variation is partly under genetic control [ 113 ]. Because of their smaller size, children are less attractive to mosquitoes than adults [ 110 , 114 ], whilst pregnant women are more attractive to mosquitoes because of their larger size and more active metabolism compared to their non-pregnant sisters [ 115 , 116 ]. Variation in attractiveness is partly explained by the variation in the skin microbiome, which results in differences in VOCs [ 78 , 117 , 118 ] Odours from human skin may be repellent or attractive [ 78 ], and thus provide an opportunity for selection of cues that could be used for prevention against mosquito bites. People with malaria are more attractive to mosquitoes, although this relationship is not clear. People infected with gametocytes appear to be more attractive to An. gambiae than uninfected people [ 119 , 120 , 121 ]. The increase in attractiveness was associated with raised concentrations of aldehydes.
After An. gambiae has identified a potential host, the combination of odours, skin humidity and body temperature induce a landing response followed by biting. Recent studies showed that odour, body heat and visual stimuli act synergistically in causing a landing response in An. gambiae mosquitoes [ 122 ]. In field studies in Burkina Faso, these stimuli combined attracted significantly more An. gambiae than either stimulus alone. Indeed, a thermal stimulus was required to obtain an optimal result [ 95 , 123 ]. In Malawi, An. arabiensis was similarly attracted to a warm human decoy [ 123 ].
Blood feeding behaviour
When a suitable site for biting has been found, blood feeding can begin, taking up to 3 min to complete. Detailed accounts of the feeding behaviour of mosquitoes include Gordon and Lumsden [ 124 ], Robinson [ 125 ], Griffiths and Gordon [ 126 ], Christophers [ 127 ] and Ribeiro [ 128 ].
The labrum plays a central role in blood uptake, as it encloses the food channel [ 129 ]. Sensory receptor cells located at the tip of the labrum detect blood quality and even direct the labrum towards a blood vessel [ 130 ]. Once blood has been detected, on average, 3 µl of blood is ingested. During feeding, diuresis of the watery parts of blood occurs, considerably reducing excess bodyweight from the blood meal and allowing the mosquito to take flight and search for a resting site. It also allows the mosquito to cool off [ 131 ].
Mosquitoes with malaria parasite in their salivary glands will probe more frequently than uninfected mosquitoes [ 132 , 133 ]. This behaviour results from destruction of parts of the salivary gland, reducing apyrase production, an important enzyme that prevents blood clotting. This behaviour has been observed with malaria-infective An. gambiae [ 134 ] and would partly explain multiple cases of malaria in the same house at the same time [ 135 , 136 ].
Diel biting patterns
The circadian activity rhythms (Fig. 2 ), distance and abundance of aquatic habitats and human population, topography, vegetation, housing quality and weather can affect the shape of the biting cycles of An. gambiae. This probably explains why biting patterns can vary markedly from site to site [ 5 , 137 ]. Yet, summarizing 92 recent studies from sub-Saharan Africa [ 138 ] showed that there was a clear tendency for An. gambiae biting activity to increase from dusk to a maximum at 01.00 h before slowly declining until 05.00 h, before dropping precipitously to low levels at 07.00 h (Fig. 6 ).
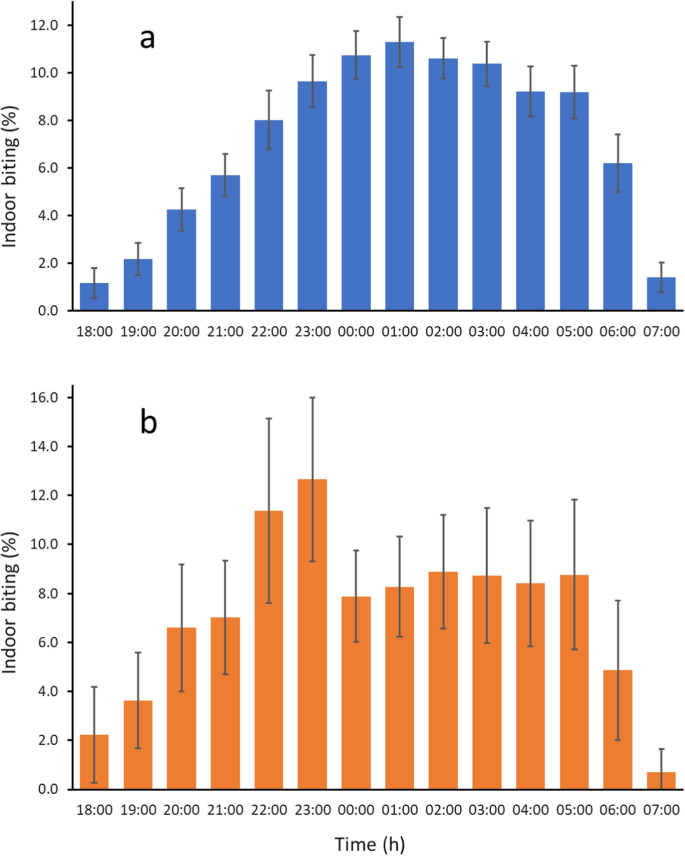
Diel biting activity of a, An. gambiae s.l. and b, An. arabiensis . Error bars are 95% confidence intervals. Data based on 92 and 17 data sets respectively. Data from Sherrard-Smith et al. [ 137 ] (data collected from 1978 to 2017)
This analysis also showed that An. arabiensis tends to bite earlier in the night than An. gambiae and An. coluzzii (Fig. 6 b). Indeed in some cases there is marked outdoor biting early in the evening [ 138 , 139 ]. In Sierra Leone young (nulliparous) An. gambiae bit earlier than parous ones [ 140 ]. In general, however, parous and nulliparous biting cycles are similar [ 5 ]. Mating does not appear to influence host-seeking behaviour in the An. coluzzii from the archipelago of São Tomé and Príncipe [ 141 ].
The paradigm of night feeding of An. gambiae (both An gambiae s.s. and An. coluzzii ) may need to be revised, however, following the results of a recent study in the Central Africa Republic [ 142 ]. It is reported that in this study 10 to 30% of indoor bites occurs during daytime. However, this activity need not necessarily be linked to the insects circadian activity (and so is a facultative behaviour, rather than an intrinsic one). This study demonstrates that studies on An. gambiae feeding behaviour should be expanded to other regions to explore the nature of these behavioural changes.
Behavioural differences
There is a tendency to provide labels of behaviour which appear fixed, such as when mosquitoes feed on animals (zoophagy) or human hosts (anthropophagy), feed inside (endophagy) or outside houses (exophagy) or rest inside (endophily) or outside houses (exophily). This is often not the case, An. gambiae can be highly variable in its behaviour throughout its range. For example, if houses are well built, with few if any holes, An. coluzzii , that might otherwise feed on humans indoors, will feed and rest outside, on whatever hosts are available, as is the case on the archipelago of São Tomé and Principe. As pointed out by Levèvre et al . [ 143 ] ‘the highly anthropophilic label given to An. gambiae s.s. must be carefully interpreted and refer to populations rather than the whole sibling species. The same is true for endophagy and exophagy. Thus, although often considered to bite predominantly outdoors, one in three blood meals among An. arabiensis from Tanzania took place indoors [ 145 ]. Similarly, in a study site from Ethiopia, where people slept outdoors next to their cattle, 46% of resting An. arabiensis collected outdoors had fed on humans despite the high cattle: human ratio (17:1) [ 145 ]. Such results suggest that the An. arabiensis population was inherently anthropophagic, although this was counterbalanced by exophagic and exophilic tendencies in the mosquito [ 146 ]. Although in An. gambiae and An. coluzzii most blood feeding occurs indoors, when people are in the house and readily available as blood host, at certain times of the year blood feeding can occur outdoors, particularly when people spend the evening sitting outdoors [ 139 ]. Although An. arabiensis tends to feed outdoors, when hosts are scarce, they may also feed indoors [ 144 , 145 , 146 ]. On some occasions An. gambiae s.s. may feed on non-human hosts, including dogs and cattle [ 147 ], presumably as a fitness strategy. These events, however, are not common and in general humans are the preferred host for this species.
Indoor insecticide use
The use of insecticides for control of African malaria vectors through ITNs and indoor residual spraying (IRS) is directed at indoor-biting mosquitoes, mostly An. gambiae , An. coluzzii and An. funestus . It has been suggested that the extensive use of insecticides throughout sub-Saharan Africa may result in a behavioural change in the mosquitoes, leading to early-evening and outdoor biting when people are unprotected mostly outdoors where the mosquitoes are not exposed to the insecticides on interior walls or bed nets [ 20 , 144 , 148 , 149 , 150 ]. Whether such changes are due to behavioural traits selected for by the use of insecticides or simply normal variation in the behaviour of different populations needs further study. What is not debatable is that extensive use of ITNs has led to a collapse of An. gambiae s.s. populations in East Africa [ 151 , 152 , 153 ].
Indoor resting
After taking a blood meal, most female mosquitoes will rest indoors for two to three days while the blood meal is digested and eggs are developed. With most females, egg laying occurs after one meal, but with young mosquitoes may require two meals [ 44 ] (Fig. 3 ). Anopheles gambiae ’s habit of resting indoors is a behaviour that is likely to increase the survival of a mosquito since the traditional thatched-roofed house protects mosquitoes against the extremely high temperatures experienced outdoors in the late afternoon and is an environment with relatively few predators. Metal-roofed houses, which are becoming increasingly common, are considerably hotter than thatched-roofed houses during the day and this results in decreased survival among indoor-resting mosquitoes [ 154 , 155 ]. At hot times of the year, mosquitoes will move to the cooler darker and moister spaces at the bottom of the wall. Reduced mosquito survival in metal-roof houses may contribute to a decline in malaria transmission in sub-Saharan Africa.
In traditional houses, mosquitoes typically rest on the roof or base of the wall, particularly where water is stored in clay pots providing a suitable micro-climate. Blood feeding depresses mosquito activity for two to three days, and these mosquitoes are far less active than other gonotrophic stages [ 31 , 156 ] resulting in most blood-feds remaining inside the house in which they fed, and only occasionally moving into neighbouring houses [ 157 ].
- Oviposition
Eggs mature in blood-fed females within two to three days after a blood meal, after which they are ready to be laid on suitable oviposition sites. Gravid mosquitoes leave the house after dusk to find an aquatic habitat in which to oviposit. Female anophelines oviposit on still water, or on aquatic vegetation on the water surface. A median of 52 eggs are laid by individual mosquitoes, in the laboratory, and many practise skip overposition, laying their eggs in several containers [ 158 ]. Anopheles gambiae are generalists when it comes to selection of a site in which to lay their eggs and they are found in a wide range of habitats not just the easy-to-find hoofprint, footprint and tyre puddles. Indeed, in many places these sites are large semi-permanent or permanent water bodies in the shade or open to the sun, with clean and dirty water—although not highly odiferous water [ 159 ]. Experiments have shown that an ovipositing mosquito is attracted by water vapour [ 160 ] and by specific odours, like cedrol found in a large number of plants [ 161 , 162 ] and nonane, a product from soil bacteria [ 163 ]. There are likely to be other chemical attractants and visual cues that also play a role in this vitally important part of the insect’s lifecycle. Future studies may lead to the development of novel ‘attract and kill strategies for malaria control.
Malaria transmission
In order for malaria transmission to occur a female An. gambiae needs to feed on a human that carries ‘male’ and ‘female’ (micro and macro-) Plasmodium gametocytes. Once in the stomach of the mosquito, the development of a flagella by the male gamete is triggered by xanthurenic acid which is a by-product of tryptophan metabolism. Exflagellation, where the eight motile gametes rupture the erythrocyte which contains them happens as a result of the drop in temperature in the mosquito. The micro-gametes move through the blood meal until they encounter a macro-gamete and fuse with it to form a motile, amoeba-like, oocyte. At this point in the life cycle the parasite is a diploid organism. At all other times it is haploid. The oocyte migrates through the midgut wall and there turns into an oocyst on the outer midgut wall. The extrinsic period of development depends critically on the environmental temperature [ 164 ]. For Plasmodium falciparum development of sporozoites in mosquitoes takes 11–12 days and for Plasmodium vivax 8–9 days at 26–27.5 °C. Plasmodium falciparum fails to develop at temperatures below 19 °C, whilst the lower limit for P. vivax is 15 °C [ 165 ]. Temperatures above 32 °C are lethal [ 158 ]. When the sporozoites are mature, oocysts rupture and sporozoites migrate through the haemocoel to the salivary glands after which the mosquito becomes infectious can pass on the parasites [ 166 ]. The percentage of female mosquitoes with sporozoites (the sporozoite rate) varies greatly, from 0 to 10% or even higher. Perhaps the factor that most affects the ability of a mosquito to be vector of disease is its survival rate. In order to become a malaria vector the mosquito has to survive through the extrinsic cycle of the parasite. This means that the mosquito, after taking an infectious meal, has to survive through four, or more, gonotrophic cycles before it will transmit.
At each phase of the gonotrophic cycle the mosquito faces different risks, each of which incurs a chance of dying. Thus, a defensive host may kill a mosquito attempting to blood feed, whilst the search for a suitable oviposition site and oviposition itself have their own risks and will depend on local conditions. For example, the risks to a mosquito that has to fly considerable distance to locate a potential oviposition site are much greater than for one where sites are to be found in close proximity to the feeding site. The risk of dying following feeding may also depend on the nature of the resting site. Mosquitoes that complete gonotrophic development inside traditional thatched roofed houses are in a more equitable environment than those that rest outdoors and so may be at less risk of desiccation. Thus, survival rate per gonotrophic cycle is important and has been estimated on a number of occasions using a variety of techniques, including dissection of the females’ ovaries to determine parous rates, mark-release-recapture experiments and estimates based on the delayed infection rate of mosquitoes [ 8 ]. In order to know the epidemiologically important figure of survival rate per day an estimate of the duration of the cycle needs to be obtained. Variations in both survival rate per cycle and cycle duration can have profound effects on the proportion of the population that might potentially become vectors. Importantly, small changes in daily survival rate can have huge consequences for the vectorial capacity of a vector [ 167 ].
On the other hand, as pointed out by [ 168 , 169 , 170 ], daily survival rates, determined by dissection, are remarkably similar between malaria vectors from different continents, which suggests that survival may be independent of the duration of the gonotrophic cycle. Cycle duration may, however, vary considerably with environmental factors [ 171 ].
In their meta-analysis of survival rate estimates according to methods used, Matthews, Bethel and Osei [ 172 ] obtained daily survival rates from dissections (vertical) of 0.83 (95% CI [0.80–0.86]), which was similar to the results obtained during population declines in the absence of recruitment [ 173 ]. Mark Release Recapture studies gave a lower value of 0.73 (95% CI [0.66–079]) whilst delayed infection rates (parasitological) gave 0.92, (CI [0.86–095]). Such differences result in large differences in estimates of vectorial capacity. Which of these methods provides the most accurate estimate is, however, not known. In many studies the effect of an intervention on survival is required, or estimates between different areas are needed. In such cases, for as long as similar methods are used for the estimates, absolute estimates are not needed.
Exploiting mosquito behaviour for surveillance and control
Over the past 20 years there has been an upsurge of interest in studying the behaviour and ecology of An. gambiae . How has this knowledge improved the control of this medically important insect? And can this be exploited to markedly enhance the toolbox of malaria control tools [ 174 , 175 ]? Here are a number of potential new tools that exploit a knowledge of mosquito behaviour and may contribute to improved malaria control (Fig. 7 ).
Screening houses
One of the most effective methods of malaria control is prevention of parasite transmission from mosquitoes to humans by preventing the entry of mosquitoes into houses [ 176 ]. When a mosquito cannot reach or find a human host, parasite transmission is effectively interrupted, and eventually malaria will die out while uninfected mosquitoes may continue to be present as they can feed elsewhere. Interruption of transmission can be achieved by making houses mosquito proof, by closing off all possible sites of entry such as eaves, windows, doors and even cracks in the wall. In such a mosquito proof house, mosquitoes cannot enter, and hence the occupants are protected from mosquito bites and their associated Plasmodium infections. There is a growing body of evidence suggesting that house modification (mainly screening) is protective against malaria [ 177 ].
Carbon dioxide is the principal cue attracting mosquitoes [ 76 , 178 ], and in traditional houses carbon dioxide from human hosts is often leaking from a house through windows, doors and eaves. Understanding the movement of carbon dioxide from a house allows one to modify the design of a house to reduce carbon dixode leakage and produce a ‘stealth house’. For example, by increasing the ventilation in a house by installing three screened windows on opposite walls reduced indoor entry of An. gambiae by 95% [ 109 ]. Alternatively, when one makes all the walls air permeable, but not mosquito permeable, there was a 99% reduction in An. arabiensis compared with the comparator experimental hut with solid walls [ 90 ]. This finding is likely to results from a substantial drop in indoor carbon dioxide concentration, making it more difficult for mosquitoes to locate a host.
Limitations: the construction of mosquito-proof houses requires the householder to pay, unlike other interventions which are given freely. Behavioural changes are needed to keep doors closed at night.
Repellents have been used as a means to prevent mosquito bites for centuries. The sensory organs of mosquitoes are activated by the repellent compound(s) leading to aversion behaviour. Historically, botanical products were used for this purpose, and since the 1950s the chemical compound DEET has been used worldwide as a skin-treatment repelling mosquitoes [ 179 , 180 ]. Novel repellents, to replace DEET, are in development at a large scale, with emphasis on human safety, residual activity and ease of application. Repellents can be used in different ways with promising results as tools for malaria prevention [ 181 , 182 , 183 ]. Long-lasting spatial repellents offer promise in the future as they can provide an area protection against mosquitoes. Recently, promising results were reported with transfluthrin-based passive emanator designed to release transfluthrin into the air. The emanators were placed indoors [ 184 , 185 ].
Limitations: topical repellents are mostly effective as personal protection, and currently few products are available that provide community protection.
Incorporating highly toxic insecticide on the rooftop of ITNs
This technology is based on the observation that, when bed nets are used, mosquitoes tend to first land on top of the net [ 186 ]. They are then exposed to an insecticide before flying off. As they spend longer on top of net than when hovering around the net, they are likely to pick up a larger dose of insecticide than when landing on the sides of the net. The discovery of increased mosquito activity at the top of the net could lead to bed nets produced with more human toxic insecticides applied to the top of the net.
Limitations: the availability of novel effective, safe, toxicants and the additional costs that require combinations of active ingredients and a net which is more complicated to construct.
Oviposition attractants
Mosquitoes ready to oviposit, find oviposition sites based on selected olfactory cues. In Kenya, the compound cedrol was found to be highly attractive to gravid An. gambiae [ 187 ]. In Tanzania, the compound nonane in combination with soil microbials led to significantly more An. gambiae eggs laid in treated water bodies than in water bodies lacking this attractant [ 163 ]. These experiments demonstrate that baiting water bodies with selective attractive cues can be used as a strategy to reduce or even eliminate An. gambiae populations. This can be done when the oviposition attractants are combined with a pesticide such as temephos or Bacillus thuringiensis israeliensis which will kill all larvae when they emerge from the eggs [ 188 ]. Studies are now needed to examine the effect of these attractants on malaria prevalence and incidence over a large area.
Limitations: although oviposition attractants have the potential to reduce mosquito populations, studies are needed to demonstrate their feasibility. Competition with a plethora of natural sites may limit effectiveness.
Toxic sugar baits
Sugar baits operate on the same principle as odour-baited traps: an attractive odour source is placed in the environment, attracting malaria vectors. By adding a toxic substance to the traps, usually a pyrethroid insecticide, mosquitoes making contact with the bait are killed. In this way, the adult mosquito population can be reduced. When critical density thresholds are reached, the malaria risk will have been significantly reduced as well [ 189 ]. A number of field trials measuring the efficacy of toxic sugar baits are currently in progress.
Potential limitations: competition with natural sugar sources may restrict the usefulness of TSBs and there is a need for long-lasting products.
Odour-baited traps
Mosquito trapping is one of the main tools used for malaria surveillance. Routine trap catches can help design and target more effective interventions. From these collections, data on species composition, nutritional state, age distribution and associated Plasmodium infections are obtained and, are used for estimating malaria transmission risk [ 190 ]. Transmission risk captured as the number of infective bites likely to be received during a malaria season or year, often expressed as the entomological inoculation rate (EIR), is one of the main parameters on which decisions on malaria control are based: when transmission risk is high, interventions are needed to reduce new infections and malaria incidence. This is particularly important in urban areas where it is necessary to distinguish between local transmission and imported cases.
Odour-baited traps have been used to lower malaria transmission risk by removing the vector population with baited traps. By employing traps over a large area of several weeks, each day a fraction of the adult mosquitoes will be removed, thereby reducing the population of infectious mosquitoes continuously. Employing odour-baited traps in western Kenya, the percentage of people with malaria was reduced by more than 30% within 1 year [ 191 ]. In this study, carbon dioxide the most effective mosquito attractant, was not used. It is believed that addition of carbon dioxide or a carbon dioxide mimic may lead to strongly enhanced reduction of malaria than was achieved in this study. Odour-baited traps can also be used for mosquito surveillance, replacing the human-biting catch (HBC), which since surveillance began, has been the most-widely used trapping method [ 192 ].
Limitations: for this tool to be effective, strong attractants need to be identified, which preferably affect more than one Anopheles species. Also, durable and affordable traps should be readily available, that can be distributed across large areas. The generation of large quantities of carbon dioxide will contribute to global warming.
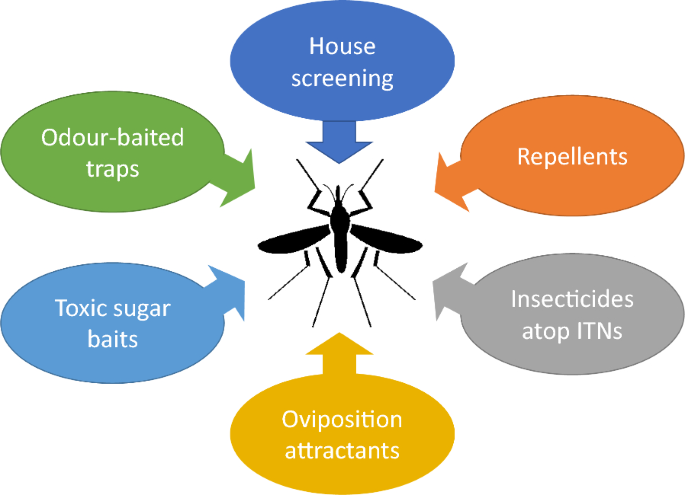
Tools for use in alternative malaria prevention or control strategies. Where ITN = insecticide-treated nets
All these interventions have the potential to select for behavioural and, where there is an active ingredient involved, physiological resistance. Behavioural resistance may occur due to avoiding an active ingredient, or in the case of house screening, exophily.
Future behavioural research
Whilst there has been a considerable improvement in the understanding of the behaviour of An. gambiae , particularly over the past 20 years, there are a number of key areas of research where little, if anything, is known, some of which are highly relevant to malaria control. These include:
Variability of behaviour
One of the major themes of this review is that there is considerable variation in behaviour between and within species of the An. gambiae complex. Yet, as far as the authors are aware, there have been no systematic reviews and meta-analyses of the behaviour of members An. gambiae in different parts of their range and over time. Such reviews are likely to provide important insights into the adaptability of this complex. A large number of publications on this behavioual variation have been written, and it will be interesting to explore if of concensus about a common trend can be reached. Climate change is an additional force that will affect mosquito behaviour, and development of parasites and their transmission. The huge variability in behaviour, as described in this paper, will no doubt be much affected by climate change and future studies will need to include this in understanding how this impacts Anopheles behaviour.
Movement across the landscape
Long-distance movement of individual mosquitoes from their aquatic habitats to finding their host is poorly understood. The shape and texture of the landscape may provide natural barriers to dispersal or provide funnels to channel mosquitoes towards hosts. A deeper understanding may help us reduce malaria transmission in some areas.
Light and vision
The role of visual attraction in host location is poorly understood in the field particularly for a nocturnal species like An. gambiae . Do mosquitoes navigate along intersections of light and dark, are they attracted to the outline of houses, and, if so does moonlight or electric lighting with tungsten or LED bulbs influence attraction or deterrence? With a rapid increase in electric lighting in parts of rural Africa it is important to understand how this may affect mosquito house entry and trapping with light traps.
Behavioural barriers to gene flow
To help accelerate the deployment of gene drive mosquitoes in the field [ 193 ] it is important to understand the barriers to mating that occur in wild populations of mosquitoes and between laboratory-reared and wild mosquitoes. Whilst scientists may anticipate rapid spread of genes through a population of wild mosquitoes there are likely to be many natural behavioural barriers that restrict or prevent gene flow. For example, in a recent study in Burkina Faso, genetically-modified mosquitoes expressed reduced fitness and survival compared to wild mosquitoes [ 194 ].
Male mosquito behaviour
Although understandably, most behavioural research has focused on adult females since only the female transmits malaria, there is little known bout the behaviour of adult males, apart from swarming and feeding. Although male mosquitoes use chemical cues for orientation, details about sensory behaviour of males are little known. Closer study of male behaviour may provide alternative targets for control.
Evolutionary changes
Although it is well recognized that mosquitoes can adapt their behaviour in consequence of the large-scale use of insecticides [ 152 ], little consideration is given to how An. gambiae adapts to new environments. Africa has the fastest urban growth worldwide [ 195 ]. By 2050, the current population of 1.4 billion people is expected to increase by nearly one billion, most of these living in growing towns and cities. In some places, An. gambiae has already adapted to this new habitat. In the 1980s, a study in Accra, showed that An. gambiae had adapted to breeding in water-filled domestic containers and more polluted aquatic habitats [ 196 ]. In Dar es Salaam, a high proportion of transmission by An. gambiae sensu lato ( s.l .) occurs outdoors [ 197 ]. Whether this represents a selected change in behaviour or one that results from houses with few entry points or both is uncertain. Nonetheless, it is important to detect changes in behaviour that may potentially favour malaria transmission. There is also the need to understand whether mosquitoes can adapt both behaviourally and physiological to extreme climate conditions [ 198 ].
Alternatives to insecticide-based interventions
For the past 20 years, ITNs and IRS have been the main tools used for malaria control in sub-Saharan Africa. They have been remarkably successful at reducing the prevalence of malaria, but this control has stalled over the past 5–7 years and the number of malaria cases each year remains static. Increasingly, target mosquitoes develop resistance to the insecticides used, and massive research in the development of “novel” insecticides, and combination use of different insecticides, have been used to maintain the status quo of reduced Plasmodium parasite transmission. This race against the evolutionary strategy is likely to be lost, as it has done clearly in agriculture [ 199 , 200 ]. Will the same strategy be used or the next 20 years or should one use alternative methods? With the growing urban populations, it is not feasible to deploy ITNs or IRS on a large scale due to poor compliance and some homes in informal settlements being too small to hang ITNs. Alternative forms of control need to be provided that are not reliant on synthetic insecticides, such as baited traps, push–pull systems.
This review illustrates the complex series of behaviours that female An. gambiae use on their journey from an aquatic habitat to a blood meal, resting and oviposition. It also underlines the huge variability in behaviours seen both between species and within species underscoring the adaptability of this mosquito to different environments. Adult mosquitoes navigate these behaviours by being exquisitely tuned to environmental cues, principally semiochemicals. A century of research has illuminated our understanding of the behaviour of this important insect and has allowed us to target vector control interventions where the mosquito is most vulnerable. Many of these interventions also apply to other mosquito vector species. Basic research on mosquito behaviour underpins applied studies on vector control and it is important that funding for this research continues to be supported in the future.
Data availability
Data availability All data supporting the findings of this study are available from corresponding author on reasonable request.
Boyd MF. Malariology: a comprehensive survey of all aspects of this group of diseases from a global standpoint, vol. 1. Saunders: University of Michigan; 1949. p. 1643.
Google Scholar
Wernsdorfer WH, McGregor I. Malaria. Principles and Practice of Malariology. Vols 1 and 2. Churchill Livingstone, Edinburgh, 1988:20208 pp.
Gillies MT. Anopheline mosquitos: vector behaviour and bionomics. In: Wernsdorfer WH, McGregor I (eds.); Malaria - Principles and Practice of Malariology. Vol. 1. Churchill Livingstone, Edinburgh. 1988:453–85
White GB. Anopheles gambiae complex and disease transmission in Africa. Trans R Soc Trop Med Hyg. 1974;68:278–99.
Article CAS PubMed Google Scholar
Gillies MT, De Meillon B. The Anophelinae of Africa South of the Sahara (Ethiopian Zoogeographical Region). 2nd edn. S Afr Inst Med Res. 1968.
White BJ, Collins FH, Besansky NJ. Evolution of Anopheles gambiae in relation to humans and malaria. Annu Rev Ecol Evol Syst. 2011;42:111–32.
Article Google Scholar
Godfray HCJ. Mosquito ecology and control of malaria. J Animal Ecol. 2013;82:15–25.
Charlwood JD. The Ecology of Malaria Vectors. Boca Raton: CRC Press, Taylor & Francis Group, LLC; 2020.
Coetzee M. Distribution of the African malaria vectors of the Anopheles gambiae complex. Am J Trop Med Hyg. 2004;70:103–4.
Article PubMed Google Scholar
Barron M, Paupy C, Rahola N, Akone-Ella O, Ngangue MF, Wilson-Bahun T, et al. A new species in the major malaria vector complex sheds light on reticulated species evolution. Sci Rep. 2019;9:14753.
Article PubMed PubMed Central Google Scholar
Niang EA, Konate L, Faye O, Diallo M, Dia I. Vector bionomics and malaria transmission in an area of sympatry of An. arabiensis , An. coluzzii and An. gambiae . Acta Trop. 2019;189:129–36.
Sinka ME, Golding N, Massey NC, Wiebe A, Huang Z, Hay SI, et al. Modelling the relative abundance of the primary African vectors of malaria before and after the implementation of indoor, insecticide-based vector control. Malar J. 2016;15:142.
Kamau L, Bennett KL, Ochomo E, Herren J, Agumba S, Otieno S, Omoke D, Matoke-Muhia D, Mburu D, Mwangangi J, Ramaita E, Juma EO, Mbobo C, Barasa S, Miles A. The Anopheles coluzzii range extends into Kenya: detection, insecticide resistance profiles and population genetic structure in relation to conspecific populations in West and Central Africa Malar J. 2024:122
Fornadel CM, Norris DE. Increased endophily by the malaria vector Anopheles arabiensis in southern Zambia and identification of digested blood meals. Am J Trop Med Hyg. 2010;83:838–42.
Gillies MT, Coetzee M. A supplement to the Anophelinae of Africa South of the Sahara. S Afr Inst Med Res. 1987.
Sawadogo PS, Namountougou M, Toé KH, Rouamba J, Maïga H, Ouédraogo KR, et al. Swarming behaviour in natural populations of Anopheles gambiae and An. coluzzii : review of 4 years survey in rural areas of sympatry, Burkina Faso (West Africa). Acta Trop. 2014;132(Suppl):S42-52.
Charlwood JD, Kessy E, Yohannes K, Protopopoff N, Rowland M, LeClair C. Studies on the resting behaviour and host choice of Anopheles gambiae and An. arabiensis from Muleba, Tanzania. Med Vet Entomol. 2018;32:263–70.
Sinka M, Bangs M, Manguin S, Coetzee M, Mbogo C, Hemingway J, et al. The dominant Anopheles vectors of human malaria in Africa, Europe and the Middle East: occurrence data, distribution maps and bionomic precis. Parasit Vectors. 2010;3:117.
Mwangangi JM, Mbogo CM, Orindi BO, Muturi EJ, Midega JT, Nzovu J, et al. Shifts in malaria vector species composition and transmission dynamics along the Kenyan coast over the past 20 years. Malar J. 2013;12:13.
Russell TL, Govella NJ, Azizi S, Drakeley CJ, Kachur SP, Killeen GF. Increased proportions of outdoor feeding among residual malaria vector populations following increased use of insecticide-treated nets in rural Tanzania. Malar J. 2011;10:80.
Munga S, Minakawa N, Zhou G, Githeko AK, Yan G. Survivorship of immature stages of Anopheles gambiae s.l. (Diptera: culicidae) in natural habitats in western Kenya highlands. J Med Entomol. 2007;44:758–64.
Takken W, Smallegange R, Vigneau A, Johnston V, Brown M, Mordue-Luntz A, et al. Larval nutrition differentially affects adult fitness and Plasmodium development in the malaria vectors Anopheles gambiae and Anopheles stephensi . Parasit Vectors. 2013;6:345.
Takken W, Klowden MJ, Chambers GM. Effect of body size on host seeking and blood meal utilization in Anopheles gambiae sensu stricto (Diptera: Culicidae): the disadvantage of being small. J Med Entomol. 1998;35:639–45.
Bayoh MN, Lindsay SW. Temperature-related duration of aquatic stages of the Afrotropical malaria vector mosquito Anopheles gambiae in the laboratory. Med Vet Entomol. 2004;18:174–9.
Lyons CL, Coetzee M, Chown SL. Stable and fluctuating temperature effects on the development rate and survival of two malaria vectors, Anopheles arabiensis and Anopheles funestus . Parasit Vectors. 2013;6:104.
Reiter P, Jones DR. An eclosion timing mechanism in the mosquito Anopheles gambiae . Physiol Entomol. 2009;50:161–8.
Lindsay SW, Armstrong Schellenberg JR, Zeiler HA, Daly RJ, Salum FM, Wilkins HA. Exposure of Gambian children to Anopheles gambiae malaria vectors in an irrigated rice production area. Med Vet Entomol. 1995;9:50–8.
Gillies MT, Wilkes TJ. The effect of high fences on dispersal of some West-African mosquitoes (Diptera-Culicidae). Bull Entomol Res. 1978;68:401–8.
Snow WF. Studies of house-entering habits of mosquitoes in The Gambia, West Africa: experiments with prefabricated huts with various wall apertures. Med Vet Entomol. 1987;1:9–21.
Gillies MT, Wilkes TJ. Field experiments with a wind-tunnel on the flight speed of some West-African Mosquitoes (Diptera, Culicidae). Bull Entomol Res. 1981;71:65–70.
Jones MDR, Gubbins SJ. Changes in the circadian flight activity of the mosquito Anopheles gambiae in relation to insemination, feeding and oviposition. Physiol Entomol. 1978;3:213–20.
Guerra CA, Reiner RC, Perkins TA, Lindsay SW, Midega JT, Brady OJ, et al. A global assembly of adult female mosquito mark-release-recapture data to inform the control of mosquito-borne pathogens. Parasit Vectors. 2014;7:276.
Bogh C, Lindsay SW, Clarke SE, Dean A, Jawara M, Pinder M, et al. High spatial resolution mapping of malaria transmission risk in the Gambia, West Africa, using LANDSAT™ satellite imagery. Am J Trop Med Hyg. 2007;76:875–81.
Majambere S, Pinder M, Fillinger U, Ameh D, Conway DJ, Green C, et al. Is mosquito larval source management appropriate for reducing malaria in areas of extensive flooding in the Gambia? a cross-over intervention trial. Am J Trop Med Hyg. 2010;82:176–84.
Mutuku FM, Alaii JA, Bayoh MN, Gimnig JE, Vulule JM, Walker ED, et al. Distribution, description, and local knowledge of larval habitats of Anopheles gambiae s.l. in a village in western Kenya. Am J Trop Med Hyg. 2006;74:44–53.
Imbahale SS, Paaijmans KP, Mukabana WR, van Lammeren R, Githeko AK, Takken W. A longitudinal study on Anopheles mosquito larval abundance in distinct geographical and environmental settings in western Kenya. Malar J. 2011;10:81.
Lehmann T, Weetman D, Huestis DL, Yaro AS, Kassogue Y, Diallo M, et al. Tracing the origin of the early wet-season Anopheles coluzzii in the Sahel. Evol Appl. 2017;10:704–17.
Article CAS PubMed PubMed Central Google Scholar
Dao A, Yaro AS, Diallo M, Timbine S, Huestis DL, Kassogue Y, et al. Signatures of aestivation and migration in Sahelian malaria mosquito populations. Nature. 2014;516:387–90.
Atieli HE, Zhou G, Zhong D, Wang X, Lee MC, Yaro AS, et al. Wind-assisted high-altitude dispersal of mosquitoes and other insects in East Africa. J Med Entomol. 2023;60:698–707.
Parmakelis A, Russello MA, Caccone A, Marcondes CB, Costa J, Forattini OP, et al. Historical analysis of a near disaster: Anopheles gambiae in Brazil. Am J Trop Med Hyg. 2008;78:176–8.
Soper FL, Wilson DB. Anopheles gambiae in Brazil 1930 to 1940. Rockefeller Foundation, 1943.
Gillies MT. The recognition of age-groups within populations of Anopheles gambiae by the pre-gravid rate and the sporozoite rate. Ann Trop Med Parasitol. 1954;48:58–74.
Charlwood JD, Pinto J, Sousa CA, Ferreira C, Petrarca V, do E Rosario V. 'A mate for a meal'- Pre-gravid behaviour of female Anopheles gambiae from the islands of Sao Tome and Principe, West Africa. Malar J. 2003;2:9.
Scott TW, Takken W. Feeding strategies of anthropophilic mosquitoes result in increased risk of pathogen transmission. Trends Parasitol. 2012;28:114–21.
Manda H, Gouagna LC, Nyandat E, Kabiru EW, Jackson RR, Foster WA, et al. Discriminative feeding behaviour of Anopheles gambiae s.s. on endemic plants in western Kenya. Med Vet Entomol. 2007;21:103–11.
Nyasembe VO, Torto B. Volatile phytochemicals as mosquito semiochemicals. Phytochem Lett. 2014;8:196–201.
Nyasembe VO, Tchouassi DP, Pirk CWW, Sole CL, Torto B. Host plant forensics and olfactory-based detection in Afro-tropical mosquito disease vectors. PLoS Negl Trop Dis. 2018;12: e0006185.
Gary RE, Foster WA. Effects of available sugar on the reproductive fitness and vectorial capacity of the malaria vector Anopheles gambiae (Diptera: Culicidae). J Med Entomol. 2001;38:22–8.
Gouagna LC, Poueme RS, Dabire KR, Ouedraogo JB, Fontenille D, Simard F. Patterns of sugar feeding and host plant preferences in adult males of An. gambiae (Diptera: Culicidae). J Vector Ecol. 2010;35:267–76.
Foster WA, Takken W. Nectar-related vs. human-related volatiles: behavioural response and choice by female and male Anopheles gambiae (Diptera: Culicidae) between emergence and first feeding. Bull Entomol Res. 2004;94:145–57.
Klowden MJ. Endogenous factors regulating mosquito host-seeking behaviour. Ciba Foundation Symposium 200. Olfaction in mosquito-host interactions. Davis EE (ed.). 1996.
Omondi S, Kosgei J, Agumba S, Polo B, Yalla N, Moshi V, et al. Natural feeding rates of Anopheles mosquitoes collected by different methods in western Kenya. Sci Rep. 2022;12:20596.
Dahan YL, Koekemoer LL. Analysis of the genitalia rotation in the male Anopheles funestus (Diptera: Culicidae). Acta Trop. 2014;132:S20–5.
Gary RE, Cannon JW, Foster WA. Effect of sugar on male Anopheles gambiae mating performance, as modified by temperature, space, and body size. Parasit Vectors. 2009;2:19.
Nignan C, Niang A, Maiga H, Sawadogo SP, Poda BS, Gnankine O, et al. Comparison of swarming, mating performance and longevity of males Anopheles coluzzii between individuals fed with different natural fruit juices in laboratory and semi-field conditions. Malar J. 2020;19:173.
Charlwood JD, Jones MDR. Mating in the mosquito, Anopheles-gambiae s.l. II. Swarming behaviour. Physiol Entomol. 1980;5:315–20.
Butail S, Manoukis NC, Diallo M, Ribeiro JMC, Paley DA. The dance of male Anopheles gambiae in wild mating swarms. J Med Entomol. 2013;50:552–9.
Baeshen R. Swarming behavior in Anopheles gambiae (sensu lato): current knowledge and future outlook. J Med Entomol. 2022;59:56–66.
Charlwood JD, Pinto J, Sousa CA, Madsen H, Ferreira C. do Rosario VE: The swarming and mating behaviour of Anopheles gambiae s.s. (Diptera: Culicidae) from São Tomé Island. J Vector Ecol. 2002;27:178–83.
CAS PubMed Google Scholar
Poda SB, Nignan C, Gnankiné O, Dabiré RK, Diabaté A, Roux O. Sex aggregation and species segregation cues in swarming mosquitoes: role of ground visual markers. Parasit Vectors. 2019;12:589.
Downes JA. The swarming and mating flight of Diptera. Ann Rev Entomol. 1969;14:271–98.
Charlwood JD, Thompson R, Madsen H. Observations on the swarming and mating behaviour of Anopheles funestus from southern Mozambique. Malar J. 2003;2:2.
Cator LJ, Ng’Habi KR, Hoy RR, Harrington LC. Sizing up a mate: variation in production and response to acoustic signals in Anopheles gambiae . Behavior Ecol. 2010;21:1033–9.
Charlwood JD, Jones MDR. Mating behaviour in the mosquito, Anopheles gambiae s.l. I. Close range and contact behaviour. Physiol Entomol. 1979;4:111–20.
Somers J, Georgiades M, Su MP, Bagi J, Andres M, Alampounti A, et al. Hitting the right note at the right time: circadian control of audibility in Anopheles mosquito mating swarms is mediated by flight tones. Sci Adv. 2022;8:eabl4844.
Sterelny K, Griffiths P (1999) Sex and death. An introduction to philosophy of biology. Chicago: University of Chicago Press.
Cator L, Hoy RR, Harrington LC. Harmonic convergence and the sexy sons hypothesis in Aedes aegypti . Am J Trop Med Hyg. 2010;83:181.
Gibson G, Warren B, Russell IJ. Humming in tune: sex and species recognition by mosquitoes on the wing. J Assoc Res Otolaryngol. 2010;11:527–40.
Diabaté A, Yaro AS, Dao A, Diallo M, Huestis DL, Lehmann T. Spatial distribution and male mating success of Anopheles gambiae swarms. BMC Evol Biol. 2011;11:184.
Charlwood JD. Swarming and mate selection in Anopheles gambiae mosquitoes (Diptera: Culicidae). J Med Entomol. 2023;60:857–64.
Dao A, Adamou A, Yaro AS, Maïga HM, Kassogue Y, Traoré SF, et al. Assessment of alternative mating strategies in Anopheles gambiae : does mating occur indoors? J Med Entomol. 2008;45:643–52.
PubMed Google Scholar
Nambunga IH, Msugupakulya BJ, Hape EE, Mshani IH, Kahamba NF, Mkandawile G, et al. Wild populations of malaria vectors can mate both inside and outside human dwellings. Parasit Vectors. 2021;14:514.
Gillies MT. A new character for the recognition of nulliparous females of Anopheles gambiae . Bull World Health Organ. 1956;15:451–9.
CAS PubMed PubMed Central Google Scholar
Klowden MJ. Sexual receptivity in Anopheles gambiae mosquitoes: absence of control by male accessory gland substances. J Insect Physiol. 2001;47:661–6.
Gabrieli P, Smidler A, Catteruccia F. Engineering the control of mosquito-borne infectious diseases. Genome Biol. 2014;15:535.
Gillies MT. The role of carbon dioxide in host-finding by mosquitoes (Diptera:Culicidae): a review. Bull Entomol Res. 1980;70:525–32.
Lucas-Barbosa D, DeGennaro M, Mathis A, Verhulst NO. Skin bacterial volatiles: propelling the future of vector control. Trends Parasitol. 2022;38:15–22.
Showering A, Martinez J, Benavente ED, Gezan SA, Jones RT, Oke C, et al. Skin microbiome alters attractiveness to Anopheles mosquitoes. BMC Microbiol. 2022;22:98.
van Loon JJA, Smallegange RC, Bukovinszkiné-Kiss G, Jacobs F, De Rijk M, Mukabana WR, et al. Mosquito attraction: crucial role of carbon dioxide in formulation of a five-component blend of human-derived volatiles. J Chem Ecol. 2015;41:567–73.
Verhulst NO, Umanets A, Weldegergis BT, Maas JPA, Visser TM, Dicke M, et al. Do apes smell like humans? The role of skin bacteria and volatiles of primates in mosquito host selection. J Exp Biol. 2018;221:jeb185959.
Montell C, Zwiebel LJ. Mosquito sensory systems. In: Raikhel AS (eds) Progress in mosquito research. Adv Insect Physiol. 2016;51:293–328.
Konopka JK, Task D, Afify A, Raji J, Deibel K, Maguire S, et al. Olfaction in Anopheles mosquitoes. Chem Senses. 2021;46:bjab021.
Suh E, Bohbot JD, Zwiebel LJ. Peripheral olfactory signaling in insects. Curr Opin Insect Sci. 2014;6:86–92.
Bidlingmayer WL. The influence of environmetal factors and physiological stage on flight patterns of mosquitoes taken in the vehicle aspirator and truck, suction, bait and New Jersey light traps. J Med Entomol. 1974;2:119–46.
Bidlingmayer WL, Hem DG. The range of visual attraction and the effect of competitive visual attractants upon mosquito (Diptera: Culicidae) flight. Bull Entomol Res. 1980;70:321–42.
Bidlingmayer WL. Mosquito flight paths in relation to environment. 1. Illumination levels, orientation, and resting areas. Ann Entomol Soc Am. 1971;64:1121–31.
Bidlingmayer WL. Mosquito flight paths in relation to environment—effect of vertical and horizontal visual barriers. Ann Entomol Soc Am. 1975;68:51–7.
Bidlingmayer WL, Hem DG. Mosquito (Diptera, Culicidae) flight behavior near conspicuous objects. Bull Entomol Res. 1979;69:691–5.
Gillies MT, Wilkes TJ. Responses of host-seeking Mansonia and Anopheles mosquitoes (Diptera: culicidae) in West Africa to visual features of a target. J Med Entomol. 1982;19:68–71.
Mmbando AS, Bradley J, Kazimbaya D, Kasubiri R, Knudsen J, Siria D, et al. The effect of light and ventilation on house entry by Anopheles arabiensis sampled using light traps in Tanzania: an experimental hut study. Malar J. 2022;21:36.
Odetoyinbo JA. Preliminary investigation on the use of a light-trap for sampling malaria vectors in the Gambia. Bull World Health Organ. 1969;40:547–60.
Charlwood JD, Rowland M, Protopopoff N, Le Clair C. The Furvela tent-trap Mk 1.1 for the collection of outdoor biting mosquitoes. PeerJ. 2017;5:e3848.
Fornadel CM, Norris LC, Norris DE. Centers for Disease Control light traps for monitoring Anopheles arabiensis human biting rates in an area with low vector density and high insecticide-treated bed net use. Am J Trop Med Hyg. 2010;83:838–42.
Olanga EA, Okal MN, Mbadi PA, Kokwaro ED, Mukabana WR. Attraction of Anopheles gambiae to odour baits augmented with heat and moisture. Malar J. 2010;9:6.
Hawkes FM, Dabire RK, Sawadogo SP, Torr SJ, Gibson G. Exploiting Anopheles responses to thermal, odour and visual stimuli to improve surveillance and control of malaria. Sci Rep. 2017;7:17283.
De Jong R, Knols BGJ. Selection of biting sites by mosquitoes. In: Olfaction in mosquito host interactions. Bock GR, Cardew G (eds). CIBA Found Symp. 1996;200:89–103.
Braack L, Hunt R, Koekemoer LL, Gericke A, Munhenga G, Haddow AD, et al. Biting behaviour of African malaria vectors: 1. Where do the main vector species bite on the human body? Parasit Vectors. 2015;8:76.
Lindsay SW, Snow RW. The trouble with eaves; house entry by vectors of malaria. Trans R Soc Trop Med Hyg. 1988;82:645–6.
Njie M, Dilger E, Lindsay SW, Kirby MJ. Importance of eaves to house entry by Anopheline, but not Culicine, mosquitoes. J Med Entomol. 2009;46:505–10.
Mshamu S, Mmbando A, Meta J, Bradley J, Bojstrup TC, Day NPJ, et al. Assessing the impact of a novel house design on the incidence of malaria in children in rural Africa: study protocol for a household-cluster randomized controlled superiority trial. Trials. 2022;23:519.
Jatta E, Jawara M, Bradley J, Jeffries D, Kandeh B, Knudsen JB, et al. How house design affects malaria mosquito density, temperature, and relative humidity: an experimental study in rural Gambia. Lancet Planet Health. 2018;2:e498–508.
Haddow AJ. The mosquito fauna and climate of native huts at Kisumu, Kenya. Bull Entomol Res. 1942;33:91–142.
Kaindoa EW, Mkandawile G, Ligamba G, Kelly-Hope LA, Okumu FO. Correlations between household occupancy and malaria vector biting risk in rural Tanzanian villages: implications for high-resolution spatial targeting of control interventions. Malar J. 2016;15:199.
Spitzen J, Koelewijn T, Mukabana WR, Takken W. Visualization of house-entry behaviour of malaria mosquitoes. Malar J. 2016;15:233.
Carrasco-Tenezaca M, Jawara M, Abdi MY, Bradley J, Brittain OS, Ceesay S, et al. The relationship between house height and mosquito house entry: an experimental study in rural Gambia. J R Soc Interface. 2021;18:20210256.
Carrasco-Tenezaca M, Jawara M, Lee DSH, Holmes MS, Ceesay S, McCall P, et al. Effect of passive and active ventilation on malaria mosquito house entry and human comfort: an experimental study in rural Gambia. J R Soc Interface. 2023;20:20220794.
Charlwood JD, Pinto J, Ferrara PR, Sousa CA, Ferreira C, Gil V, et al. Raised houses reduce mosquito bites. Malar J. 2003;2:45.
Parker JEA, Angarita-Jaimes N, Abe M, Towers CE, Towers D, McCall PJ. Infrared video tracking of Anopheles gambiae at insecticide-treated bed nets reveals rapid decisive impact after brief localised net contact. Sci Rep. 2015;5:13392.
Jatta E, Carrasco-Tenezaca M, Jawara M, Bradley J, Ceesay S, D’Alessandro U, et al. Impact of increased ventilation on indoor temperature and malaria mosquito density: an experimental study in The Gambia. J R Soc Interface. 2021;18:178.
Carnevale P, Frezil JL, Bosseno MF, Le Pont F, Lancien J. Etude de l’agressivité d’ Anopheles gambiae A en fonction de l’age et du sexe des sujets humains. Bull World Health Organ. 1978;56:147–54.
Lindsay SW, Adiamah JH, Miller JE, Pleass RJ, Armstrong JR. Variation in attractiveness of human subjects to malaria mosquitoes (Diptera: Culicidae) in The Gambia. J Med Entomol. 1993;30:368–73.
Knols BGJ, De Jong R, Takken W. Differential attractiveness of isolated humans to mosquitoes in Tanzania. Trans R Soc Trop Med Hyg. 1995;89:604–6.
Fernandez-Grandon GM, Gezan SA, Armour JAL, Pickett JA, Logan JG. Heritability of attractiveness to mosquitoes. PLoS ONE 215;10(4): e0122716.
Port GR, Boreham PFL, Bryan JH. The relationship of host size to feeding by mosquitoes of the Anopheles gambiae Giles complex (Diptera: Culicidae). Bull Entomol Res. 1980;70:133–44.
Lindsay S, Ansell J, Selman C, Cox V, Hamilton K, Walraven G. Effect of pregnancy on exposure to malaria mosquitoes. Lancet. 2000;355:1972.
Ansell J, Hamilton KA, Pinder M, Walraven GE, Lindsay SW. Short-range attractiveness of pregnant women to Anopheles gambiae mosquitoes. Trans R Soc Trop Med Hyg. 2002;96:113–6.
Verhulst NO, Andriessen R, Groenhagen U, Kiss GB, Schulz S, Takken W, et al. Differential attraction of malaria mosquitoes to volatile blends produced by human skin bacteria. PLoS ONE. 2010;5: e15829.
Busula AO, Takken W, De Boer JG, Mukabana WR, Verhulst NO. Variation in host preferences of malaria mosquitoes is mediated by skin bacterial volatiles. Med Vet Entomol. 2017;31:320–6.
Lacroix R, Mukabana WR, Gouagna LC, Koella JC. Malaria infection increases attractiveness of humans to mosquitoes. PLoS Biol. 2005;3: e298.
Busula AO, Bousema T, Mweresa CK, Masiga D, Logan JG, Sauerwein RW, et al. Gametocytemia and attractiveness of Plasmodium falciparum -infected Kenyan children to Anopheles gambiae mosquitoes. J Infect Dis. 2017;216:291–5.
Robinson A, Busula AO, Voets MA, Beshir KB, Caulfield JC, Powers SJ, et al. Plasmodium -associated changes in human odor attract mosquitoes. Proc Natl Acad Sci USA. 2018;115:E4209–18.
Carnaghi M, Belmain SR, Hopkins RJ, Hawkes FM. Multimodal synergisms in host stimuli drive landing response in malaria mosquitoes. Sci Rep. 2021;11:7379.
Zembere K, Chirombo J, Nasoni P, McDermott DP, Tchongwe-Divala L, Hawkes FM, et al. The human-baited host decoy trap (HDT) is an efficient sampling device for exophagic Anopheles arabiensis within irrigated lands in southern Malawi. Sci Rep. 2022;12:3428.
Gordon RM, Lumsden WHR. A study of the behaviour of the mouth-parts of mosquitoes when taking up blood from living tissue; together with some observations on the ingestion of microfilariae. Ann Trop Med Parasitol. 1939;33:259–78.
Robinson GG. The mouthparts and their function in the female mosquito, Anopheles maculipennis . Parasitology. 1939;31:212–42.
Griffiths RB, Gordon RM. An apparatus which enables the process of feeding by mosquitoes to be observed in the tissues of a live rodent; together with an account of the ejection of saliva and its significance in malaria. Ann Trop Med Parasitol. 1952;46:311–9.
Christophers SR. The yellow fever mosquito. Cambridge: Cambridge University Press; 1960.
Ribeiro JMC. Blood-feeding arthropods - live syringes or invertebrate pharmacologists. Infect Agents Dis. 1995;4:143–52.
Clements AN. The biology of mosquitoes. London: Chapman & Hall; 1992.
Choo YM, Buss GK, Tan KM, Leal WS. Multitasking roles of mosquito labrum in oviposition and blood feeding. Front Physiol. 2015;6:306.
Lahondère C, Lazzari CR. Mosquitoes cool down during blood feeding to avoid overheating. Curr Biol. 2012;22:40–5.
Ribeiro JMC, Rossignol PA, Spielman A. Role of mosquito saliva in blood vessel location. J Exp Biol. 1984;108:1–7.
Rossignol PA, Ribeiro JM, Spielman A. Increased biting rate and reduced fertility in sporozoite-infected mosquitoes. Am J Trop Med Hyg. 1986;35:277–9.
Koella JC, Sorensen FL, Anderson RA. The malaria parasite, Plasmodium falciparum , increases the frequency of multiple feeding of its mosquito vector, Anopheles gambiae . Proc Biol Sci. 1998;265:763–8.
Lindsay SW, Campbell H, Adiamah JH, Greenwood AM, Bangali JE, Greenwood BM. Malaria in a peri-urban area of The Gambia. Ann Trop Med Parasitol. 1990;84:553–62.
Kabbale FG, Akol AM, Kaddu JB, Onapa AW. Biting patterns and seasonality of Anopheles gambiae sensu lato and Anopheles funestus mosquitoes in Kamuli District, Uganda. Parasit Vectors. 2013;6:340.
Sherrard-Smith E, Hogan AB, Hamlet A, Watson OJ, Whittaker C, Winskill P, et al. The potential public health consequences of COVID-19 on malaria in Africa. Nat Med. 2020;26:1411–6.
Yohannes M, Haile M, Ghebreyesus TA, Witten KH, Getachew A, Byass P, et al. Can source reduction of mosquito larval habitat reduce malaria transmission in Tigray, Ethiopia? Trop Med Int Health. 2005;10:1274–85.
Mburu MM, Mzilahowa T, Amoah B, Chifundo D, Phiri KS, van den Berg H, et al. Biting patterns of malaria vectors of the lower Shire valley, southern Malawi. Acta Trop. 2019;197: 105059.
Bockarie MJ, Alexander N, Bockarie F, Ibam E, Barnish G, Alpers M. The late biting habit of parous Anopheles mosquitoes and pre-bedtime exposure of humans to infective female mosquitoes. Trans R Soc Trop Med Hyg. 1996;90:23–5.
Charlwood JD, Pinto J, Sousa CA, Ferreira C, Gil V, Do Rosario VE. Mating does not affect the biting behaviour of Anopheles gambiae from the islands of Sao Tome and Principe, West Africa. Ann Trop Med Parasitol. 2003;97:751–6.
Sangbakembi-Ngounou C, Costantini C, Longo-Pendy NM, Goagouni C, Akoni-Ella O, Rahola N, et al. Diurnal biting of malaria mosquitoes in the Central African Republic indicates residual transmission may be “out of control.” Proc Natl Acad Sci USA. 2022;119: e2104282119.
Lefevre T, Gouagna LC, Dabire KR, Elguero E, Fontenille D, Costantini C, et al. Evolutionary lability of odour-mediated host preference by the malaria vector Anopheles gambiae . Trop Med Int Health. 2009;14:228–36.
Killeen GF, Govella NJ, Lwetoijera DW, Okumu FO. Most outdoor malaria transmission by behaviourally-resistant Anopheles arabiensis is mediated by mosquitoes that have previously been inside houses. Malar J. 2016;15:225.
Tirados I, Costantini C, Gibson G, Torr SJ. Blood-feeding behaviour of the malarial mosquito Anopheles arabiensis : implications for vector control. Med Vet Entomol. 2006;20:425–37.
Mburu MM, Zembere K, Mzilahowa T, Terlouw AD, Malenga T, van den Berg H, et al. Impact of cattle on the abundance of indoor and outdoor resting malaria vectors in southern Malawi. Malar J. 2021;20:353.
Sousa CA, Pinto J, Almeida PG, Ferreira C, Do Rosário VEJ, Charlwood JD. J Med Entomol. 2001;38:122–5.
Gatton ML, Chitnis N, Churcher T, Donnelly MJ, Ghani AC, Godfray HCJ, et al. The importance of mosquito behavioural adaptations to malaria control in Africa. Evolution. 2013;67:1218–30.
Kreppel KS, Viana M, Main BJ, Johnson PCD, Govella NJ, Lee Y, et al. Emergence of behavioural avoidance strategies of malaria vectors in areas of high LLIN coverage in Tanzania. Sci Rep. 2020;10:14527.
Perugini E, Guelbeogo WM, Calzetta M, Manzi S, Virgillito C, Caputo B, et al. Behavioural plasticity of Anopheles coluzzii and Anopheles arabiensis undermines LLIN community protective effect in a Sudanese-savannah village in Burkina Faso. Parasit Vectors. 2020;13:277.
Killeen GF, Seyoum A, Sikaala C, Zomboko AS, Gimnig JE, Govella NJ, et al. Eliminating malaria vectors. Parasit Vectors. 2013;6:172.
Bayoh MN, Mathias D, Odiere M, Mutuku F, Kamau L, Gimnig J, et al. Anopheles gambiae : historical population decline associated with regional distribution of insecticide-treated bed nets in western Nyanza Province, Kenya. Malar J. 2010;9:62.
Finda MF, Limwagu AJ, Ngowo HS, Matowo NS, Swai JK, Kaindoa E, et al. Dramatic decreases of malaria transmission intensities in Ifakara, south-eastern Tanzania since early 2000s. Malar J. 2018;17:362.
Lindsay SW, Jawara M, Mwesigwa J, Achan J, Bayoh N, Bradley J, et al. Reduced mosquito survival in metal-roof houses may contribute to a decline in malaria transmission in sub-Saharan Africa. Sci Rep. 2019;9:7770.
Lindsay SW, Davies M, Alabaster G, Altamirano H, Jatta E, Jawara M, et al. Recommendations for building out mosquito-transmitted diseases in sub-Saharan Africa: the DELIVER mnemonic. Philos Trans R Soc Lond B Biol Sci. 2021;376:20190814.
Takken W, Van Loon JJA, Adam W. Inhibition of host-seeking response and olfactory responsiveness in Anopheles gambiae following blood feeding. J Insect Physiol. 2001;47:303–10.
Boreham PFL, Port GR. The distribution and movement of engorged females of Anopheles gambiae Giles (Diptera: Culicidae) in a Gambian village. Bull Ent Res. 1982;72:489–95.
Okal MN, Lindh JM, Torr SJ, Masinde E, Orindi B, Lindsay SW, et al. Analysing the oviposition behaviour of malaria mosquitoes: design considerations for improving two-choice egg count experiments. Malar J. 2015;14:250.
Fillinger U, Lindsay SW. Larval source management for malaria control in Africa: myths and reality. Malar J. 2011;10:353.
Okal MN, Francis B, Herrera-Varela M, Fillinger U, Lindsay SW. Water vapour is a pre-oviposition attractant for the malaria vector Anopheles gambiae sensu stricto . Malar J. 2013;12:365.
Lindh JM, Okal MN, Herrera-Varela M, Borg-Karlson A-K, Torto B, Lindsay SW, et al. Discovery of an oviposition attractant for gravid malaria vectors of the Anopheles gambiae species complex. Malar J. 2015;14:119.
Eneh LK, Fillinger U, Karlson AKB, Rajarao GK, Lindh J. Anopheles arabiensis oviposition site selection in response to habitat persistence and associated physicochemical parameters, bacteria and volatile profiles. Med Vet Entomol. 2019;33:56–67.
Schoelitsz B, Mwingira V, Mboera LEG, Beijleveld H, Koenraadt CJM, Spitzen J, et al. Chemical mediation of oviposition by Anopheles mosquitoes: a push-pull system driven by volatiles associated with larval stages. J Chem Ecol. 2020;46:397–409.
MacDonald G. The epidemiology and control of malaria. London: Oxford University Press; 1957.
Warrell DA, Gilles HM. Bruce-Chwatt’s Essential Malariology. 4th ed. Boca Raton, Florida, USA: CRC Press; 2002.
Josling GA, Llinas M. Sexual development in Plasmodium parasites: knowing when it’s time to commit. Nat Rev Microbiol. 2015;13:573–87.
Garrett-Jones C, Shidrawi GR. Malaria vectorial capacity of a population of Anopheles gambiae . Bull World Health Organ. 1969;40:531–45.
Hii JLK, Birley MH, Sang VY. Estimation of survival rate and oviposition interval of Anopheles balabacensis mosquitos from mark recapture experiments in Sabah, Malaysia. Med Vet Entomol. 1990;4:135–40.
Hii JL. Evidence for the existence of genetic variability in the tendency of Anopheles balabacensis to rest in houses and to bite man, Southeast Asian. J Trop Med Public Health. 1985;16:173–82.
CAS Google Scholar
Hii JL, Kan S, Vun YS, Chin KF, Lye MS, Mak JW, et al. Anopheles flavirostris incriminated as a vector of malaria and Bancroftian filariasis in Banggi Island, Sabah, Malaysia. Trans R Soc Trop Med Hyg. 1985;79:677–80.
Beck-Johnson LM, Nelson WA, Paaijmans KP, Read AF, Thomas MB, Bjornstad ON. The effect of temperature on Anopheles mosquito population dynamics and the potential for malaria transmission. PLoS ONE. 2013;8: e79276.
Matthews J, Bethel A, Osei G. An overview of malarial Anopheles mosquito survival estimates in relation to methodology. Parasit Vectors. 2020;13:233.
Charlwood JD, Birley MH, Dagaro H, Paru R, Holmes PR. Assessing survival rate of Anopheles farauti (Diptera: Culicidae) from New Guinea. J Anim Ecol. 1985;54:1003–16.
Killeen GF, Masalu JP, Chinula D, Fotakis EA, Kavishe DR, Malone D, et al. Control of malaria vector mosquitoes by insecticide-treated combinations of window screens and eave baffles. Emerg Infect Dis. 2017;23:782–9.
Williams YA, Tusting LS, Hocini S, Graves PM, Killeen GF, Kleinschmidt I, et al. Expanding the vector control toolbox for malaria elimination: a systematic review of the evidence. Adv Parasitol. 2018;99:345–79.
WHO. Guidelines for malaria. Geneva: World Health Organization; 2023.
Fox T, Furnival-Adams J, Chaplin M, Napier M, Olanga EA. House modifications for preventing malaria. Cochrane Database Syst Rev. 2022;2022: CD013398.
PubMed Central Google Scholar
Mboera LEG, Takken W. Carbon dioxide chemotropism in mosquitoes (Diptera: Culicidae) and its potential in vector surveillance and management programmes. Med Vet Entomol. 1997;85:355–68.
Bohbot JD, Dickens JC. Insect repellents: modulators of mosquito odorant receptor activity. PLoS ONE. 2010;5: e12138.
Afify A, Potter CJ. Insect repellents mediate species-specific olfactory behaviours in mosquitoes. Malar J. 2020;19:127.
Mmbando AS, Batista EPA, Kilalangongono M, Finda MF, Mwanga EP, Kaindoa EW, et al. Evaluation of a push-pull system consisting of transfluthrin-treated eave ribbons and odour-baited traps for control of indoor- and outdoor-biting malaria vectors. Malar J. 2019;18:87.
Masalu JP, Finda M, Killeen GF, Ngowo HS, Pinda PG, Okumu FO. Creating mosquito-free outdoor spaces using transfluthrin-treated chairs and ribbons. Malar J. 2020;19:109.
Njoroge MM, Hiscox A, Saddler A, Takken W, van Loon JJA, Fillinger U. Less is more: repellent-treated fabric strips as a substitute for full screening of open eave gaps for indoor and outdoor protection from malaria mosquito bites. Parasit Vectors. 2022;15:259.
Syafruddin D, Asih PBS, Rozi IE, Permana DH, Nur Hidayati AP, Syahrani L, et al. Efficacy of a spatial repellent for control of malaria in indonesia: a cluster-randomized controlled trial. Am J Trop Med Hyg. 2020;103:344–58.
Achee NL, Perkins TA, Moore SM, Liu F, Sagara I, Van Hulle S, et al Spatial repellents: the current roadmap to global recommendation of spatial repellents for public health use. Curr Res Parasitol Vector Borne Dis. 2022;9:100107.
Jones J, Murray GPD, McCall PJ. A minimal 3D model of mosquito flight behaviour around the human baited bed net. Malar J. 2021;20:24.
Eneh LK, Saijo H, Borg-Karlson AK, Lindh JM, Rajarao GK. Cedrol, a malaria mosquito oviposition attractant is produced by fungi isolated from rhizomes of the grass Cyperus rotundus . Malar J. 2016;15:478.
Mwingira V, Dicke M, Takken W. Exploiting the chemical ecology of mosquito-oviposition surveillance and control: a review. J Vect Ecol. 2020;12:155–79.
Traore MM, Junnila A, Traore SF, Doumbia S, Revay EE, Kravchenko VD, et al. Large-scale field trial of attractive toxic sugar baits (ATSB) for the control of malaria vector mosquitoes in Mali, West Africa. Malar J. 2020;19:72.
Amoah B, McCann RS, Kabaghe AN, Mburu M, Chipeta MG, Moraga P, et al. Identifying Plasmodium falciparum transmission patterns through parasite prevalence and entomological inoculation rate. Elife. 2021;10: e65682.
Homan T, Hiscox A, Mweresa CK, Masiga D, Mukabana WR, Oria P, et al. The effect of mass mosquito trapping on malaria transmission and disease burden (SolarMal): a stepped-wedge cluster-randomised trial. Lancet. 2016;388:1193–201.
Hawkes F, Manin BO, Ng SH, Torr SJ, Drakeley C, Chua TH, Ferguson HM. Evaluation of electric nets as means to sample mosquito vectors host-seeking on humans and primates. Parasit Vectors. 2017;10:338.
James SL, Dass B, Quemada H. Regulatory and policy consideration for the implementation of gene drive-modified mosquitos to prevent malaria transmission. Transgenic Res. 2023;32:17–32.
Yao FA, Millogo AA, Epopa PS, North A, Noulin F, Dao K, et al. Mark-release-recapture experiment in Burkina Faso demonstrates reduced fitness and dispersal of genetically-modified sterile malaria mosquitoes. Nat Commun. 2022;13:796.
United Nations. World Urbanization Prospects. The 2018 Revision. 2019:103pp.
Chinery WA. Effects of ecological changes on the malaria vector Anopheles funestus and the Anopheles gambiae complex of mosquitoes in Accra, Ghana. J Trop Med Hyg. 1984;87:75–81.
Geissbühler Y, Chaki P, Emidi B, Govella NJ, Shirima R, Mayagaya V, et al. Interdependence of domestic malaria prevention measures and mosquito-human interactions in urban Dar es Salaam, Tanzania. Malar J. 2007;6:126.
Sternberg ED, Thomas MB. Local adaptation to temperature and the implications for vector-borne diseases. Trends Parasitol. 2014;30:115–22.
Thomas MB, Godfray HCJ, Read AF, van den Berg H, Tabashnik BE, van Lenteren JC, et al. Lessons from agriculture for the sustainable management of malaria vectors. PLoS Med. 2012;9: e1001262.
Sternberg ED, Thomas MB. Insights from agriculture for the management of insecticide resistance in disease vectors. Evol Appl. 2018;11:404–14.
Patin E, Qiuntana-Murci L. The demographic and adaptive history of central African hunter-gatherers and farmers. Curr Opin Genet Develop. 2018;53:90–7.
Article CAS Google Scholar
Patin E, Siddle KJ, Laval G, Quach H, Harmant C, Becker N, Froment A, et al. The impact of agricultural emergence on the genetic history of African rainforest hunter-gatherers and agriculturalists. Nat Commun. 2014;5:3163.
Aimé C, Laval G, Patin E, Verdu P, Ségurel L, Chaix R, et al. Human genetic data reveal contrasting demographic patterns between sedentary and nomadic populations that predate the emergence of farming. Mol Biol Evol. 2013;30:2629–44.
Snow RW, Trape JF, Marsh K. The past, present and future of childhood malaria mortality in Africa. Trends Parasitol. 2001;17:593–7.
Livi-Bacci M. A Concise History of World Population, 6th Edn. Wiley-Blackwell; 2017.
Manning P. The impact of agricultural emergence on the genetic history of African rainforest hunter-gatherers and agriculturalists. In: African Economic History Conference. Vancouver, 2013:13.
Tabutin D, Schoumaker B. The demography of sub-saharan Africa from the 1950s to the 2000s: a survey of changes and a statistical assessment. Population. 2004;59:97.
Thornton JK. Demography and history in the kingdom of Kongo, 1550–1750. J Afr Hist. 1977;18:507–30.
WHO. World malaria report 2022. Geneva: World Health Organization; 2022.
Bolt J, van Zanden JL. Maddison Project Database 2020. Groningen Growth and Development Centre, Groningen, 2021.
Download references
Acknowledgements
We thank Maureen Coetzee for advice on the Anopheles gambiae species complex. Jeroen Spitzen is thanked for his comments on the house-entry section.
Author information
Authors and affiliations.
Laboratory of Entomology, Wageningen University & Research, PO Box 16, 6700 AA, Wageningen, The Netherlands
Willem Takken
Global Health and Tropical Medicine, Instituto de Hygiene e Medicina Tropical, Lisbon, Portugal
Derek Charlwood
Department of Biosciences, Durham University, Durham, UK
Steve W. Lindsay
You can also search for this author in PubMed Google Scholar
Contributions
All authors contributed equally to the manuscript.
Corresponding author
Correspondence to Willem Takken .
Ethics declarations
Competing interests.
The authors declare no competing interests.
Additional information
Publisher's note.
Springer Nature remains neutral with regard to jurisdictional claims in published maps and institutional affiliations.
Estimating the number of malaria deaths attributed to Anopheles gambiae s.l.
Whilst it is impossible to make precise estimates of the number of deaths from malaria attributed to An. gambiae , it is likely to be considerable. It is estimated that about 241 million children under five years old have died from this disease in sub-Saharan Africa between 3000 BC and 2000 AD, making it the world’s greatest assassin. Part of the reason for this enormous attrition is because, unusually for a mosquito, An. gambiae evolved a propensity for feeding on people, presumably when humankind began cultivating crops, becoming relatively sedentary, with an increase in group size and sleeping in the same place for several years, making it more likely that vector populations increased in abundance increasing malaria. Shifting agriculture was the earliest form of agriculture practised in the forests of Gabon around 5000 years ago [ 201 , 202 , 203 ]. In this system the forest was cleared to grow crops for two to three years before moving on when soil nutrients were depleted. When the people moved to neighbouring forests, mosquitoes followed with them as humans formed a reliable food source, unlike wildlife which were relatively scarce in the rainforest with larger home ranges [ 203 ]. It is likely this would have led to an increase in malaria in early ancestral populations.
The accumulated number of malaria deaths for those aged 0–4 years old was calculated using median values of mortality determined by Snow et al . [ 204 ]. For the entire period pre-1960 the rate of 9.5/10,000 based on data from six studies. Was used. The population of sub-Saharan Africa was determined annually from 3000 BCE to 2000 CE by linear interpolation from estimates for specific years obtained from the literature [ 205 , 206 ]. It was assumed that in 10,000 BCE that half the world’s population was living in sub-Saharan Africa, representing 3 million people. Since malaria is largely a rural disease, the rural population for Africa was estimated using data from FAOSTAT (accessed 27th April 2022) between 1950 and 2000. The 1950 estimate for 86% of the population of Africa for earlier years was used in 1950, 42.3% of the population were 0–4 years old compared to 44.3% in 2000 [ 207 ]. Annual values between 1950 and 2000 were linearly interpolated. For earlier years, the proportion of 0–4 years old were estimated from a historical study in the Kongo from 1550 to 1750 [ 203 ]. In this study the birth rate was 47 births/1000 with a crude mortality rate in children of about 25%. It is assumed that half of these deaths (12.5%) were due to causes other than malaria. Since the total African rural population was 2,580,000 (total population in Africa = 3,000,000 × 0.86 proportion living in rural areas) there would be 121,260 rural births each year with 530,512 births every 5 years (121,260) with 12.5% of these dying from causes other than malaria during this period, resulting in 397,884 survivors, ~ 15.5% of the population (i.e. 464,198/3,000,000). The assumption was that the proportion was constant from year to year before the increase in the population seen in sub-Saharan Africa from 1900 onwards. After 1900, annual values were interpolated linearly using the values given by Tabutin and Schoumaker [ 208 ].
It is estimated that 241 million children aged 0–4 years old died from malaria between 3000 BCE and 2000 CE. Making the conservative assumption that 50% of malaria deaths were due to An. gambiae mosquitoes feeding on people suggest that this mosquito was responsible for 120.5 M deaths. In 2021, of the 625,000 malaria deaths that occurred globally, 96% occurred in sub-Saharan Africa [ 209 ], living testament to the remarkable ability of this mosquito species, and to An. funestus , to transmit malaria. It should be appreciated that the estimation of deaths from malaria attributed to these mosquitoes is, understandably, an imprecise science with a wide variation in estimates. There are variations between annual population estimates by different authors [ 205 , 210 ]. Estimating the proportion of children aged 0–4 years old in historical records is based on only one data source and is therefore a biased sample. Importantly, malaria deaths in older age groups or the large number of malaria deaths that would have occurred during pregnancy have been left out of these calculations.
Rights and permissions
Open Access This article is licensed under a Creative Commons Attribution 4.0 International License, which permits use, sharing, adaptation, distribution and reproduction in any medium or format, as long as you give appropriate credit to the original author(s) and the source, provide a link to the Creative Commons licence, and indicate if changes were made. The images or other third party material in this article are included in the article's Creative Commons licence, unless indicated otherwise in a credit line to the material. If material is not included in the article's Creative Commons licence and your intended use is not permitted by statutory regulation or exceeds the permitted use, you will need to obtain permission directly from the copyright holder. To view a copy of this licence, visit http://creativecommons.org/licenses/by/4.0/ . The Creative Commons Public Domain Dedication waiver ( http://creativecommons.org/publicdomain/zero/1.0/ ) applies to the data made available in this article, unless otherwise stated in a credit line to the data.
Reprints and permissions
About this article
Cite this article.
Takken, W., Charlwood, D. & Lindsay, S.W. The behaviour of adult Anopheles gambiae , sub-Saharan Africa’s principal malaria vector, and its relevance to malaria control: a review. Malar J 23 , 161 (2024). https://doi.org/10.1186/s12936-024-04982-3
Download citation
Received : 03 October 2023
Accepted : 11 May 2024
Published : 23 May 2024
DOI : https://doi.org/10.1186/s12936-024-04982-3
Share this article
Anyone you share the following link with will be able to read this content:
Sorry, a shareable link is not currently available for this article.
Provided by the Springer Nature SharedIt content-sharing initiative
- Anopheles gambiae
- Malaria control
- Malaria surveillance
Malaria Journal
ISSN: 1475-2875
- Submission enquiries: [email protected]

IMAGES
VIDEO
COMMENTS
1. Introduction. Malaria affected an estimated 219 million people causing 435,000 deaths in 2017 globally. This burden of morbidity and mortality is a result of more than a century of global effort and research aimed at improving the prevention, diagnosis, and treatment of malaria [].Malaria is the most common disease in Africa and some countries in Asia with the highest number of indigenous ...
Literature review 1.1 Malaria in Africa Malaria is a common and life-threatening disease in many tropical and subtropical developing countries. There were an estimated 881 000 malaria deaths during 2006, of which 91% were in Africa and 85% were of children under 5 years of age (WHO, 2008). Malaria is responsible
HIV-infected adults are at an increased risk of severe malaria and death 38. The overall prevalence of helminth ... amount of literature on the impressive ... review malaria biology and the ...
Background Malaria is a major public health problem in sub-Saharan Africa, and children are especially vulnerable. In 2019, an estimated 409,000 people died of malaria, most (274,000) were young children and 94% of the cases and deaths were in Africa. Prior studies in Ethiopia focused on the adult population and high transmission areas. Hence, this study aimed to determine the prevalence and ...
For studies to be included in the review, they had to fulfill the following criteria: (i) articles reporting data from SSA; (ii) articles that included data on the following outcomes of interest: clinical malaria test positivity rate, malaria case period prevalence, incidence from facility-based surveillance, or community-based disease surveillance; and (iii) articles reporting continuous data ...
Literature on integrated malaria prevention, defined as the use of two or more malaria prevention methods holistically, was searched from 1st January 2001 to 31st July 2021. The primary outcome variables were malaria incidence and prevalence, while the secondary outcome measures were human biting and entomological inoculation rates, and ...
This scoping review aims to map evidence of the prevalence, contextual factors and health education interventions of malaria amongst children under 5 years (UN5) in SSA.
Reduction in malaria prevalence between 2000 and 2015 in Africa. ... The review of the literature suggests that the use of Artemisia annua tea does not seem to be a solution because it tends to ...
The prevalence of child malaria in this study area was 35.4%, which was equivalent to the national malaria prevalence in 2017 (36%) and just slightly lower than malaria prevalence in central and rural Malawi, at 39.7 and 40.6%, respectively. This finding raises concerns regarding whether long-lasting insecticidal nets (LLINs) and sulfadoxine ...
Although in 2016 there was a significant reduction in the number of deaths attributable to malaria (1037 in 2015 to 590 in 2016) as well as the decline in Case Fatality Rate (CFR) (0.51 in 2015 to 0.32 in 2016) among children under-five, the proportion of cases attributable to malaria for under-five children remains high at 46.7% [].According to the Ghana National Malaria Control Programme ...
According to the Ghana demographic and health survey, malaria prevalence ranges from 11.2 to 40.0% . In this study, the prevalence of malaria was found to be 20.9%. This is consistent with a study conducted in Ghana on the prevalence of malaria-positive rapid diagnostic tests and antimalarial treatment which reported a 21.58% prevalence rate .
The result of this systematic review and meta-analysis was much higher than the recent national prevalence of malaria among the general population in Ethiopia. Parasite prevalence in Ethiopia was 0.5% by microscopy and 1.2% by RDTs for areas below 2,000 meters and less than 0.1% prevalence above 2,000 meters [ 21 ].
The overall prevalence of malaria over the 7 years was 1.7% (257/14888). ... This review is based on extensive review of literature on both the POR strategies and elimination schemes of countries ...
It was also 1.41 times higher in males than in females. Moreover, the odds of malaria prevalence were 1.60, 1.64, 2.45 and 1.82 times higher in the age group of < 5, 5-14, 15-24 and 25-54 ...
See Full PDFDownload PDF. 1 Chapter 1: Literature review 1.1 Malaria Malaria in Africa kills a vast number of children every year. By the time you have read the next paragraph, two children will have died: in Africa, a child dies from malaria every 30 seconds, which translates to a massive 2880 children per day (Finkel, 2007).
In this review, we sought to assess the extent and quality of qualitative research published between 2011 and 2021 pertaining to the non-use of all types of mosquito nets and, drawing on the findings from this literature, update current understanding of reasons for mosquito net non-use in malaria-endemic countries.
There is limited literature on studies comparing malaria prevalence using months after IRS, many ... malaria prevalence and incidence using pirimiphos-methyl in the context of pyrethroid resistance ... malaria prevention in low- and middle-income countries: a systematic review. Malaria Journal. 2023;22(1):79. 34. Ministry of Health. National ...
Current malaria prevalence was higher with microscopy (67.33%) than that of RDT (23.33%). ... A high-level review was conducted of the literature pertaining to the challenges and opportunities for ...
Malaria prevalence was relatively low in 684 children 0-9 years visited in the same survey (all <6.5% STable 7 Supplementary File). We detected no significant associations between household SMC ...
Chemoprevention strategies reduce malaria disease and death, but the efficacy of anti-malarial drugs used for chemoprevention is perennially threatened by drug resistance. This review examines the current impact of chemoprevention on the emergence and spread of drug resistant malaria, and the impact of drug resistance on the efficacy of each of the chemoprevention strategies currently ...
Current strategies to protect children from malaria—administration of new RTS,S/AS01 or R21/Matrix-M vaccines as well as seasonal medications—require about 20 health care visits by the time a child reaches age 5 years. However, an approach involving the annual administration of a monoclonal antibody...
During our literature search, we identified 1 outbreak linked to food that might have included an irradiated ingredient. During 2009-2010, Salmonella enterica serotype Montevideo was found in imported pepper used in ready-to-eat salami . Some of the manufacturer's pepper was reportedly irradiated, but some was not.
Congenital malaria is defined as the identification of asexual P. falciparum parasites in the cord blood or peripheral blood of an infant during the first 7 days of life. 40 The true prevalence of congenital malaria is uncertain. While the prevalence was previously thought to be between <1% and 6%, 40 more recent studies have demonstrated a ...
The prevalence of anaemia in India remains high in children, especially those in rural areas, and in women of childbearing age, and its impairment of neurological development can have serious lifelong effects. It is concerning that the most recent official data (2019-21) indicate an increased prevalence compared with 2015-16. There is also considerable variability in childhood anaemia ...
Mosquitoes of the Anopheles gambiae complex are one of the major vectors of malaria in sub-Saharan Africa. Their ability to transmit this disease of major public health importance is dependent on their abundance, biting behaviour, susceptibility and their ability to survive long enough to transmit malaria parasites. A deeper understanding of this behaviour can be exploited for improving vector ...