Geography Notes
Tidal hypothesis of james jeans | tides| geography.
ADVERTISEMENTS:
Sir James Jeans, a British scientist, propounded his ‘tidal hypothesis’ to explain the origin of the earth in the year 1919 while another British scientist, Harold Jeffreys, suggested modifications in the ‘tidal hypothesis’ in 1929 and thus made it more relevant and significant in the context of increasing knowledge of the cosmogonic ideas of the 1st quarter of the 20th century. Tidal hypothesis is one of the modern hypotheses of the origin of the earth and the solar system.
Jeans postulated his hypothesis on the basis of certain axioms (self-proved facts) as given below:
(1) The solar system was formed from the sun and another intruding star.
(2) In the beginning the sun was a big incandescent gaseous mass of matter.
(3) Besides the sun, there was another star termed as ‘intruding star’ in the universe. This intruding star was much bigger in size than the primitive sun.
(4) The primitive sun was stationary and was rotating on its axis.
(5) The ‘intruding star’ was moving along such a path in such a way that it was destined to come nearer to the primitive sun.
(6) There was a great impact of the tidal force of the intruding star on the surface of the primitive sun.
James Jeans postulated that due to massive gravitational force of the ‘intruding star’, huge amount of matter was ejected from the primitive sun, which later on became the building material of future planets. According to the Newton’s law of universal gravitation (1687) everybody in the universe attracts every other body with a force which is directly proportional to the product of the masses of the two bodies and inversely proportional to the square of the distance between them.
It means that the intensity of the gravitational force of the ‘intruding star’ and the ‘primitive sun’ would depend upon two factors e.g.:
(i) Product of the masses of the intruding star and the primitive sun, and
(ii) The distance between the two stars.
There is direct relation between the product of the masses of the ‘intruding star’ and the primitive sun (equation 3). Gravitation a Product of the Force mass of the two stars………….. (3)
It means that if the product of the masses of the ‘intruding star’ and the ‘primitive sun’ is high, the amount of gravitational force between them will also be high and if the product of masses of the two stars is low the amount of gravitational force will also be low. In simple words, it also means that if the mass of the ‘introding star’ is high, the gravitational force exerted by it will also be high.
Since James Jeans assumed the ‘intruding star’ to be much bigger than the ‘primitive sun’, the former exerted larger amount of gravitational pull on the surface of the ‘primitive sun’ than the gravitational pull of the ‘primitive sun’ which caused ejection Of matter from the ‘primitive sun’.
Secondly, there is an inverse relation between the gravitational force of two stars and the distance between them (equation 4).
It means that as the distance between two stars decreases, the amount of gravitational force between them increases and as the distance between two stars increases, the gravitational force between them decreases. Since the ‘intruding star’ was coming closer to the ‘primitive sun’ and hence the amount of gravitational force between them was also increasing.
Evolution of filament:
According to James Jeans the ‘intruding star’ was continuously moving along such a path that it was coming nearer to the primitive sun and thus exerted gaseous tidal force (gravitational pull) on the surface of the ‘primitive sun’. As the ‘intruding star’ came nearer and nearer to the ‘primitive sun’, its gravitational force went on increasing with the result tidal force also increased.
When the ‘intruding star’ came nearest to the ‘primitive sun’ its gravitational force became maximum, with the result a giant cigar-shaped tide, thousands of kilometres in length, was created on the outer surface of the ‘primitive sun’ and ultimately huge mass of matter, in the shape of a cigar, was ejected from the ‘primitive sun’. James Jeans called this cigar-shaped matter as filament which was much thicker in the centre and thinner and sharper at the ends (tapering ends) (see fig. 2.1).
The shape of the ejected filament with thick middle portion and tapering ends may be explained in the following manner:
(i) When the ‘intruding star’ was at distant place from the ‘primitive sun’ (but not so distant that it could not exert attractional pull on the surface of the ‘primitive sun’) the gravitational attraction exerted by it on the surface of the primitive sun was also low due to greater distance between them. Therefore small quantity of the First part of the incandescent matter came out from the surface of the primitive sun.
(2) When the intruding star came nearest to the primitive sun, maximum amount of gravitational attraction was exerted by it on the surface of the primitive sun due to minimum distance between them. This attractional and tidal force resulted into the ejection of maximum amount of the incandescent matter (which represented the middle portion of the filament).
(3) When the ‘intruding star’ moved away from the primitive sun (but not so away that it could not exert attractional pull on the primitive sun), the gravitational attraction exerted by it on the primitive sun became low due to greater distance between them.
Thus, again small quantity of incandescent matter came out from the surface of the primitive sun. Thus, the shape of matter ejected from the primitive sun became like a cigar which was called by James Jeans as ‘filament.’
(4) Gradually and gradually the intruding star moved far away from the primitive sun and thus went out of the domain of the gravitational field of our primitive sun. In the beginning, when the filament was detached from the primitive sun it followed the intruding star as it was pulled by the intruding star.
The filament tried to keep pace with the intruding star for some time but could not succeed and ultimately it (filament) started revolving around the primitive sun.
Formation of planets from the filament:
According to James Jeans nine planets of our solar system were formed due to cooling and condensation of the incandescent mass of gaseous matter of the filament. The filament, after being detached from the sun, began to cool down. Thus, the filament started contracting in size on cooling. The contraction of the filament led to its breaking in several pieces and each piece was condensed to form one separate planet. This process led to the formation of nine planets.
The filament of incandescent gaseous matter allowed bigger planets to form in its middle portion (like Jupiter and Saturn) and smaller ones towards its tapering ends. The remaining part of the primitive sun became our sun. The satellites of the planets were formed due to gravitational pull and tidal effect exerted by the sun on the outer surfaces of the newly formed planets.
The processes of the formation of satellites cased when the amount of matter ejected from the planets for the formation of new satellites became so low that it was not able to hold together its matter by its central gravitational force/attraction.
The rate of cooling of the primitive incandescent gaseous planets was dependent upon the size of the planet. The planets of greater mass cooled very slowly while the smaller planets and satellites condensed to liquid and then to solid forms within very short period. This may be the possible reason for larger number of satellites of bigger planets and fewer number of satellites of smaller planets.
Very small planets were cooled and condensed soon, so no matter could be ejected from their surface due to tidal effect and thus no satellite could be formed. This is why Mercury, Venus and Pluto do not have any satellite.

Evidence in Favour of the Hypothesis :
The ‘tidal hypothesis’ of James Jeans has not only explained the origin of the solar system and the earth but has also attempted successfully to some extent, to solve various problems of the solar system related to its shape, size, structure and peculiar motion.
The following characteristics of the solar system are more or less explained on the basis of this hypothesis:
(1) Shape and Ordering of Planets:
The filament ejected from the primitive sun was cigar-shaped. It was thick in the middle portion and thin at the ends. The planets formed from the condensation of the incandescent gaseous matter of the middle portion of the filament were bigger in size than the planets formed from the condensation of matter at the tapering ends of the filament (fig. 2.2).
This arrangement of the planets formed of the filament clearly matches with the present planetary arrangement of our solar system. If we start from the sun, the size of the planets (Mercury, Venus, Earth, Mars-which is an exception) increases upto Jupiter which is the biggest planet and occupies almost the central position in the solar system.
The size of the planets after Jupiter (Saturn, Uranus, and Naptune) again decreases till the location of the smallest planet- Pluto at the extreme end. It may be pointed out that James Jeans propounded his hypothesis before the discovery of Pluto. Thus, the discovery of planet Pluto having smallest size and its location at the extreme end further enhanced the significance of tidal hypothesis.
(2) Ordering and Arrangement of Satellites:
According to this hypothesis the satellites of the planets were formed through the condensation of incandescent gaseous matter ejected from the planets in the same manner as the planets were formed through the condensation of incandescent gaseous matter ejected from the sun. Thus, there is almost the same type of arrangement of satellites around the planets as is the arrangement of planets around the sun in our solar system i.e. smaller ones at the end and bigger ones in the middle.
For example, if a planet has several satellites, the biggest satellite is placed in the middle and smaller ones arc placed at the end. The arrangement of satellites of Jupiter and Saturn confirms the above fact.
(3) Number and Size of Satellites:
According to tidal hypothesis bigger planets remained in gaseous form for longer time in the space because their cooling took longer time due to their large size. On the other hand, smaller planets were cooled and condensed within very short period of time because of their small size.
Thus, larger number of satellites were formed from bigger planets but the size of such satellites was relatively small whereas fewer number of satellites were formed from medium-sized planets but the size of such satellites was relatively larger. Very small planets occupying the end location of planetary system were cooled and condensed within very short period of time and hence no satellites could be formed from them. The existing planetary arrangement of the solar system confirms the above fact.
For example, Jupiter and Saturn (biggest planets) have 14 and 15 satellites respectively while Mercury, Venus and Pluto, which are smallest planets of our solar system do not have any satellite (table 2.1).
(4) Rotation, revolution and inclination of the planets’ axis:
According to James Jeans the planets were formed from the filament which was revolving around the sun. Thus, the planets of our solar system should also revolve around the sun. In our present day solar system all the planets are revolving around the sun in the same direction and also in the same orbital plane (the orbital plane of Pluto is however slightly inclined).
Similarly, almost all their satellites of the planets are also revolving around their respective planets in the same direction as their father planets are revolving around the sun. James Jeans also proposed that the rotational motion of the planets should also be in the same direction. Except Venus and Uranus all the planets of the present solar system rotate on their axis in the same direction as their revolution around the sun.
As per tidal hypothesis the encounter of the primitive sun and the intruding star was not in the same planes of their rotation. The filament, which was ejected from the primitive sun by the gravitational attraction of the intruding star, was slightly inclined. Thus the axis of rotation of the planets, which were formed from the inclined filament (inclined from the plane of the rotation of our sun), should also be inclined. It is apparent from table 2.1 that all the axes of the planets of the present solar system are inclined. However, the planes of the orbital paths of various planets are also not matching with the plane of rotation of our sun.
Modification by Jeffreys :
Harold Jeffreys, a British scientist, modified the original tidal hypothesis of James Jeans in 1929 and presented his concept as ‘collision hypothesis’. According to Jeffreys there were three stars in the universe before the origin of our solar system. One was our primitive sun, the second one was its ‘companion star’ and the third one was ‘intruding star’ which was moving towards ‘companion star’.
Thus, the intruding star collided against the ‘companion star’. Due to head-on collision the companion star was completely smashed and shattered, some shattered portions were scattered in the sky while remaining debris started revolving around the primitive sun. However, the impact of collision and explosion enabled the intruding star to clear itself off from the gravitational attraction of the primitive sun and gradually vanished in the universe.
The planets of our solar system were formed from the remaining debris of the companion star. It may be pointed out that Jeffreys suggested modifications in the tidal hypothesis of James Jeans with the intention to remove major inherent weak points of the tidal hypothesis so that it can withstand the criticisms of the modern scientific world.
Evaluation :
The tidal hypothesis as propounded by James Jeans and modified by Harold Jeffreys enjoyed a long lease of popularity and wide appreciation till the end of the 1st half of the 20th century because of its simplicity, inherent logic and scientific appeal but the hypothesis was severely criticised on various grounds. Even Jeffreys accepted in Guttenberg (1951) that his modified version of tidal hypothesis needed substantial modifications and in some places was completely wrong.
The following criticisms have been labelled against this hypothesis:
(1) According to B. Levin the universe is infinite in space and time and the stars are so distant from each other that such a close encounter between them is a remote possibility.
(2) James Jeans did not explain the where about and destiny of the intruding star/which caused tidal, eruption on the surface of the primitive sun in the form of filament.
(3) N.N. Parisky has proved on the basis of mathematical calculation that tidal hypothesis fails to explain the real distances between the sun and the planets in our present solar system. Hoyle has maintained that if ‘we reduce the solar system to scale, representing the sun by a ball of the size of a large grape fruit, the bulk of the planetary material lies at a distance of some 100 yards from it.’
That is why American astronomer Russell expressed serious doubts about the fact that such a huge amount of material of the filament would have come out from the primitive sun to form planets at much greater distances from the sun, some times more than 500 to 3000 times the diameter of the sun (the distances of Jupiter and Naptune from the sun respectively). In fact, according to this hypothesis the planets should have been very close to the sun but in reality they are far away from the sun.
(4) According to Wooldrige and Morgan ‘the fundamental difficulty is that the planets comprising but a small fraction of the total mass of the system, yet at great distances from the sun, carry most of the angular momentum of the system’. Many modern astrophysicists are of the opinion that the angular momentum imparted by the intruding star to the planets was not high enough to match the existing angular momentum of the planets of our present solar system.
(5) The planets of our solar system are largely formed of the elements having high atomic weight but the constituent elements of the sun (from which the planets are supposed to have been formed) are of lighter atomic weight e.g. hydrogen and helium. The tidal hypothesis fails to offer convincing explanation for such anomalous situation,
(6) Another problem is that of the formation of satellites from the planets. If for the time being we accept the view that satellites were formed from the tidal effect exerted by the primitive sun on the newly born planets, then question arises, why no satellite was formed from Mercury and Venus though these planets were nearest to the sun.
In the circumstances the sun should have exerted maximum gravitational force on the outer surfaces of Mercury and Venus. The argument that small planets were condensed to solid form within very short period of time and hence there was no time for the ejection of matter from these planets does not appear sound.
(7) James Jeans could not elaborate the process and mechanism of the condensation of matter ejected from the primitive sun.
Related Articles:
- Origin of the Solar System and Earth | Geography
- Ocean Tides: Introduction, Origin and Types | Ocean Tides | Geography
- What Causes the Tide to Rise and Fall? | Tides | Geography
- Notes on Tidal Bores | Seawater | Geography
Geography , Tidal Hypothesis , Tidal Hypothesis of James Jeans , Tides
Privacy Overview
Cookie | Duration | Description |
---|---|---|
cookielawinfo-checkbox-analytics | 11 months | This cookie is set by GDPR Cookie Consent plugin. The cookie is used to store the user consent for the cookies in the category "Analytics". |
cookielawinfo-checkbox-functional | 11 months | The cookie is set by GDPR cookie consent to record the user consent for the cookies in the category "Functional". |
cookielawinfo-checkbox-necessary | 11 months | This cookie is set by GDPR Cookie Consent plugin. The cookies is used to store the user consent for the cookies in the category "Necessary". |
cookielawinfo-checkbox-others | 11 months | This cookie is set by GDPR Cookie Consent plugin. The cookie is used to store the user consent for the cookies in the category "Other. |
cookielawinfo-checkbox-performance | 11 months | This cookie is set by GDPR Cookie Consent plugin. The cookie is used to store the user consent for the cookies in the category "Performance". |
viewed_cookie_policy | 11 months | The cookie is set by the GDPR Cookie Consent plugin and is used to store whether or not user has consented to the use of cookies. It does not store any personal data. |
- Subscriber Services
- For Authors
- Publications
- Archaeology
- Art & Architecture
- Bilingual dictionaries
- Classical studies
- Encyclopedias
- English Dictionaries and Thesauri
- Language reference
- Linguistics
- Media studies
- Medicine and health
- Names studies
- Performing arts
- Science and technology
- Social sciences
- Society and culture
- Overview Pages
- Subject Reference
- English Dictionaries
- Bilingual Dictionaries
Recently viewed (0)
- Save Search
- Share This Facebook LinkedIn Twitter
Related Content
'tidal theory' can also refer to....
Tidal Creation Theory
More Like This
Show all results sharing these subjects:
- Earth Sciences and Geography
tidal theory
Quick reference.
Theory of the origin of the solar system, involving the approach near the Sun of another star. This set up tidal forces, and the instability of the Sun resulted in part of its mass being torn off to form the planets. The theory was proposed by Sir James H. Jeans (1877–1946) and Sir Harold Jeffreys.
From: tidal theory in A Dictionary of Earth Sciences »
Subjects: Science and technology — Earth Sciences and Geography
Related content in Oxford Reference
Reference entries.
View all related items in Oxford Reference »
Search for: 'tidal theory' in Oxford Reference »
- Oxford University Press
PRINTED FROM OXFORD REFERENCE (www.oxfordreference.com). (c) Copyright Oxford University Press, 2023. All Rights Reserved. Under the terms of the licence agreement, an individual user may print out a PDF of a single entry from a reference work in OR for personal use (for details see Privacy Policy and Legal Notice ).
date: 25 July 2024
- Cookie Policy
- Privacy Policy
- Legal Notice
- Accessibility
- [185.66.14.236]
- 185.66.14.236
Character limit 500 /500
Tidal Hypothesis of Jeans and Jeffreys - Geography Notes
Amruta Patil
Jul 19, 2024
IAS Exam Latest Updates
- 19 July, 2024 : UPSC CSE Prelims Name-wise Result 2024 PDF Out
- 19 July, 2024 : UPSC Fires Puja Khedkar: Check Official Press Release Here
Sir James Jeans , a British scientist, proposed his ' tidal hypothesis ' to explain the origin of the earth in 1919 , while Harold Jeffreys , another British scientist, suggested modifications to the 'tidal hypothesis' in 1929 , making it more relevant and significant in the context of growing knowledge of the cosmogonic ideas of the first quarter of the twentieth century. The tidal hypothesis is a modern theory about the origins of the planet and solar system. In this article, you will read about the Tidal Hypothesis of Jeans and Jeffreys in detail for the IAS exam.
|

Assumptions of Tidal Hypothesis of Jeans and Jeffreys
- The sun and another invading star formed the solar system.
- The sun was once a massive incandescent gaseous mass of materials.
- Aside from the sun, there was another star in the universe known as an ' intruding star .' This intruding star was much bigger in size than the primitive sun.
- The sun was stationary and rotated on its axis. The ‘intruding star’ was moving along a path in such a way that it was destined to come nearer to the primitive sun.
- The intruding star's tidal force had a significant impact on the surface of the primitive sun.
- According to James Jeans, a massive amount of matter was ejected from the primitive sun as a result of the intruding star's massive gravitational force, which later became the building material for future planets.
- Everyone in the universe attracts every other body with a force that is directly proportional to the product of the two bodies' masses and inversely proportional to the square of the distance between them, according to Newton's law of universal gravitation.
Evolution of Filament
- According to James Jeans, the 'intruding star' was constantly moving closer to the primitive sun, exerting gaseous tidal force (gravitational pull) on its surface.
- As the 'intruding star' got closer to the 'primitive sun,' its gravitational attraction grew stronger, and the tidal force grew stronger as well.
- When the 'intruding star' got close enough to the 'primitive sun’, its gravitational pull reached its maximum, causing a massive cigar-shaped tide to form on the 'primitive sun's outer surface,' with a massive mass of matter ejected from the 'primitive sun' in the shape of a cigar.
- This cigar-shaped substance, which was much thicker in the middle and thinner and sharper at the ends, was dubbed “filament” by James Jeans.
Formation of Planets from the Filament
- According to James Jeans, the cooling and condensation of the filament's incandescent mass of gaseous matter resulted in the formation of eight planets in our solar system.
- After being separated from the sun, the filament began to cool. As the filament cooled, it began to shrink in size.
- The filament's contraction caused it to break into numerous fragments, each of which was condensed to become a new planet. Eight planets were formed as a result of this event.
- The filament of incandescent gaseous matter allowed for the formation of larger planets in the centre (such as Jupiter and Saturn) and smaller planets at the tapering extremities.
- Our sun was created from the remnants of the primitive sun. The sun's gravitational pull and tidal influence on the newly created planets caused the satellites to develop.
- When the amount of matter ejected from planets for the production of new satellites grew so low that its central gravitational force/attraction could no longer hold it together, the processes of satellite formation stopped.
- The size of the planet determined the rate of cooling of the ancient incandescent gaseous planets.
- The larger planets and satellites cooled slowly, whereas the smaller planets and satellites condensed to liquid and then solid forms in a short time. This could explain why larger planets have more satellites while smaller planets have fewer.
- Very small planets were cooled and condensed soon, so no matter could be ejected from their surface due to the tidal effect and thus no satellite could be formed. This is why Mercury, Venus and Pluto do not have any satellites.
Modification by Jeffreys
- In 1929, Harold Jeffreys, a British physicist, updated James Jeans' initial tidal hypothesis and presented his concept as the ' collision hypothesis .'
- Before the birth of our solar system, there were three stars in the universe, according to Jeffreys.
- The first was our primitive sun , the second was its 'companion star,' and the third was an 'intruding star' approaching the 'companion star.'
- As a result, the 'intruding star' collided with the 'compaion star.' Due to head-on collision the companion star was completely smashed and shattered, some shattered portions were scattered in the sky while remaining debris started revolving around the primitive sun.
- However, the impact of the collision and explosion allowed the intruding star to break free from the original sun's gravitational pull and gradually fade away from the cosmos.
- The planets of our solar system were formed from the companion star's debris.
- It should be noted that Jeffreys proposed changes to James Jeans' tidal theory with the goal of removing key fundamental flaws in the tidal hypothesis so that it could survive modern scientific critique.
Criticism of Tidal Hypothesis of Jeans and Jeffreys
- According to B. Levin, the universe is limitless in space and time, and the stars are so far apart that such a close encounter is unlikely.
- James Jeans did not explain the location or fate of the intruding star that triggered tidal, filament-like eruptions on the ancient sun's surface.
- On the basis of mathematical calculations, N.N. Parisky has demonstrated that the tidal hypothesis fails to explain the real distances between the sun and the planets in our current solar system.
- Our solar system's planets are mostly made up of high-atomic-weight elements, whereas the sun's constituent elements (from which the planets are assumed to have formed) are lighter atomic-weight elements, such as hydrogen and helium. The tidal hypothesis falls short of providing a credible explanation for such a strange occurrence.
- James Jeans was unable to explain the technique and mechanism by which materials ejected from the primitive sun condensed.
The tidal hypothesis, as proposed by James Jeans and modified by Harold Jeffreys, enjoyed a long period of popularity and wide acceptance until the end of the first half of the twentieth century, but the hypothesis was severely criticised on various grounds. Even Jeffreys admitted in Guttenberg (1951) that his modified version of the tidal hypothesis needed significant revisions and was incorrect in several places.
Other Relevant Links | |
---|---|
Question: What is tidal hypothesis?
The tidal hypothesis is one of the modern hypotheses of the origin of the earth and the solar system.
Question: Who gave the tidal hypothesis?
In 1919, Sir James Jeans, a British scientist, proposed his "tidal hypothesis" to explain the formation of the earth. In 1929, Harold Jeffreys proposed a modification to the "tidal hypothesis," making it more meaningful and relevant in the light of growing scientific knowledge.
Question: What is the difference between nebular hypothesis and tidal hypothesis?
According to the Nebular hypothesis, a huge swirling cloud of hot gases and dust is where the sun and planets first emerged. The tidal hypothesis states that the sun had experienced a tidal tug from a passing star, but the result was a lengthy filament of gases being dragged from the sun.
Question: Mention any two assumptions of the tidal hypothesis.
Question: What was the criticism of the tidal hypothesis?
The criticisms of the tidal hypothesis are:
- N.N. Parisky has proved on the basis of mathematical calculation that the tidal hypothesis fails to explain the real distances between the sun and the planets in our present solar system.
- James Jeans could not elaborate on the process and mechanism of the condensation of matter ejected from the primitive sun.
Question: Consider the following statements related to tidal hypothesis:
- The tidal hypothesis explains the real distances between the sun and the planets in our present solar system.
- Immanuel Kant, a German philosopher, developed the tidal hypothesis.
Which of the statements given above is/are correct?
(c) Both 1 and 2
(d) Neither 1 nor 2
Answer: (d) See the Explanation
- N.N. Parisky has proved on the basis of mathematical calculation that the tidal hypothesis fails to explain the real distances between the sun and the planets in our present solar system. Hence, statement 1 is incorrect.
- Sir James Jeans, a British scientist, proposed his 'tidal hypothesis' to explain the origin of the earth in 1919, while Harold Jeffreys, another British scientist, suggested modifications to the 'tidal hypothesis' in 1929. Hence, statement 2 is incorrect.
Therefore, option (d) is the correct answer.
Question: Who propounded the tidal hypothesis?
(a) Immanuel Kant
(b) Aristotle
(c) Chamberlain
(d) None of the above
Sir James Jeans, a British scientist, proposed his 'tidal hypothesis' to explain the origin of the earth in 1919, while Harold Jeffreys, another British scientist, suggested modifications to the 'tidal hypothesis' in 1929.
Therefore, option (d) is the correct answer.
UPSC : Polity - Parliament
Upsc : modern history - india under british raj and governance, upsc : geography - projects and programs, upsc : economy - tax structure, upsc : science & tech - chemistry, upsc cse : polity - fundamental rights - 01, daily quiz: up police: mental ability (direction sense), daily quiz: rrb ntpc: (series), daily quiz: ctet: science (science around us), daily quiz: ctet: social studies (human geography), daily quiz: ctet: history (gupta period), daily quiz: delhi police: problem solving (direction & distance), daily quiz: ctet: physics (electricity and magnetism), daily quiz: upsc cse (ias): national movement (1905-1919) - ii, 5 oct daily ca quiz for upsc & state pscs, general science (pyqs) live test for upsc cse 2025 (july 22 - 25), indian polity (pyqs) live test for upsc cse 2025 (july 22 - 25).

UPSC CDS English All India Mock Test
Upsc cds general knowledge all india mock test, upsc civil services prelims csat full test 7, upsc civil services prelims csat full test 6, upsc civil services prelims csat full test 5, upsc civil services prelims csat full test 4, upsc civil services prelims csat full test 3, upsc civil services prelims csat full test 2, upsc civil services prelims csat full test 1, upsc civil services prelims general studies full test 11, upsc civil services prelims 2023: general studies (set - a - held on 28 may), upsc cse 2023 (prelims paper-1: general studies) previous year paper (28-may-2023), upsc civil services prelims 2022: csat official paper, upsc civil services prelims 2022: general studies official paper, upsc cse 2022 (prelims paper-2: csat) previous year paper (05-jun-2022), upsc cse 2022 (prelims paper-1: general studies) previous year paper (5-june-2022), upsc civil services exam (prelims) csat official paper-ii (held on: 2021), upsc civil services exam (prelims) general studies official paper-i (held on: 10 oct 2021), upsc cse 2021 (prelims paper-2: csat) previous year paper (10-oct-2021), upsc cse 2021 (prelims paper-1: general studies) previous year paper (10-oct-2021).
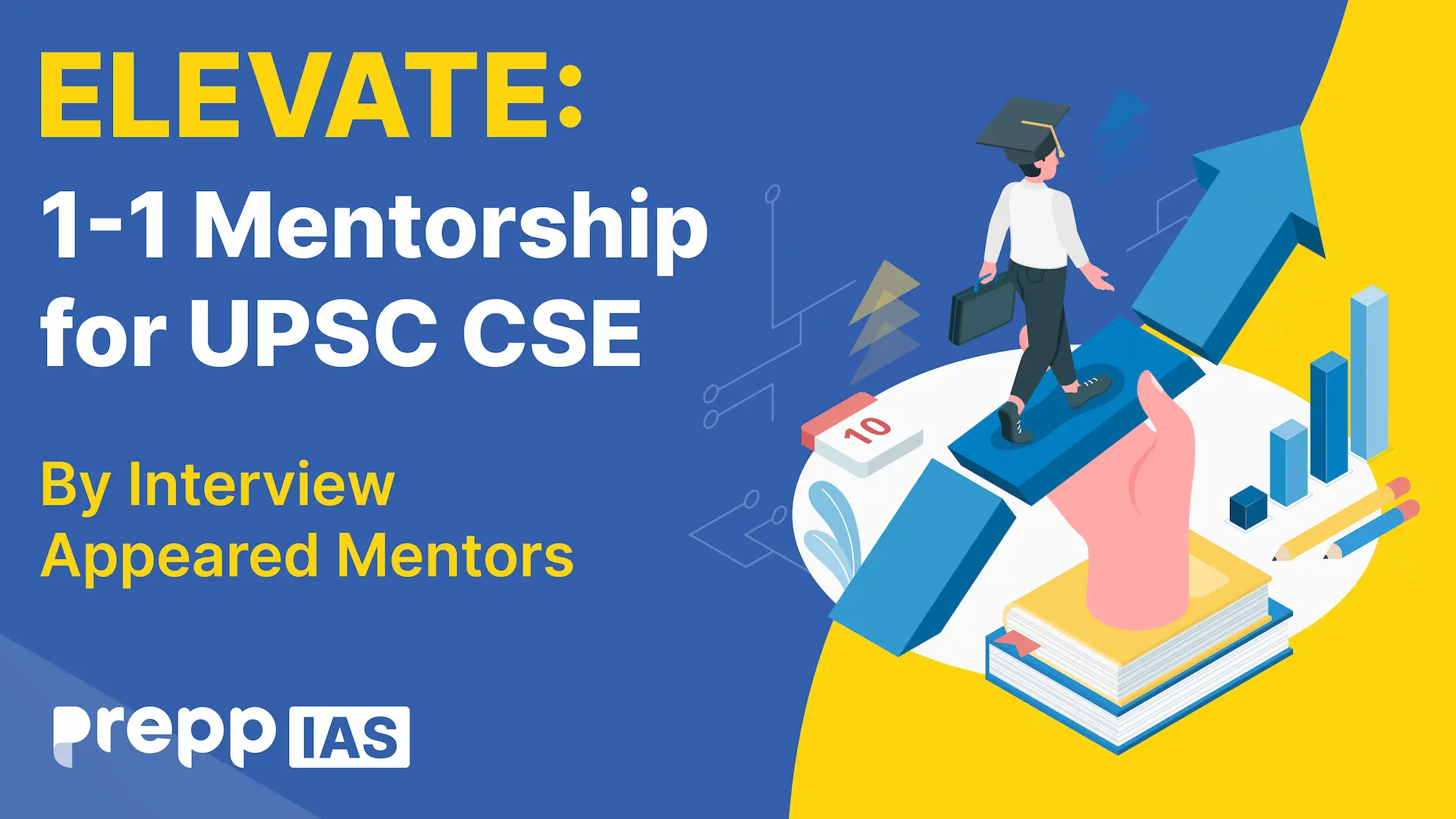
UPSC CSE 2025 Mentorship Course
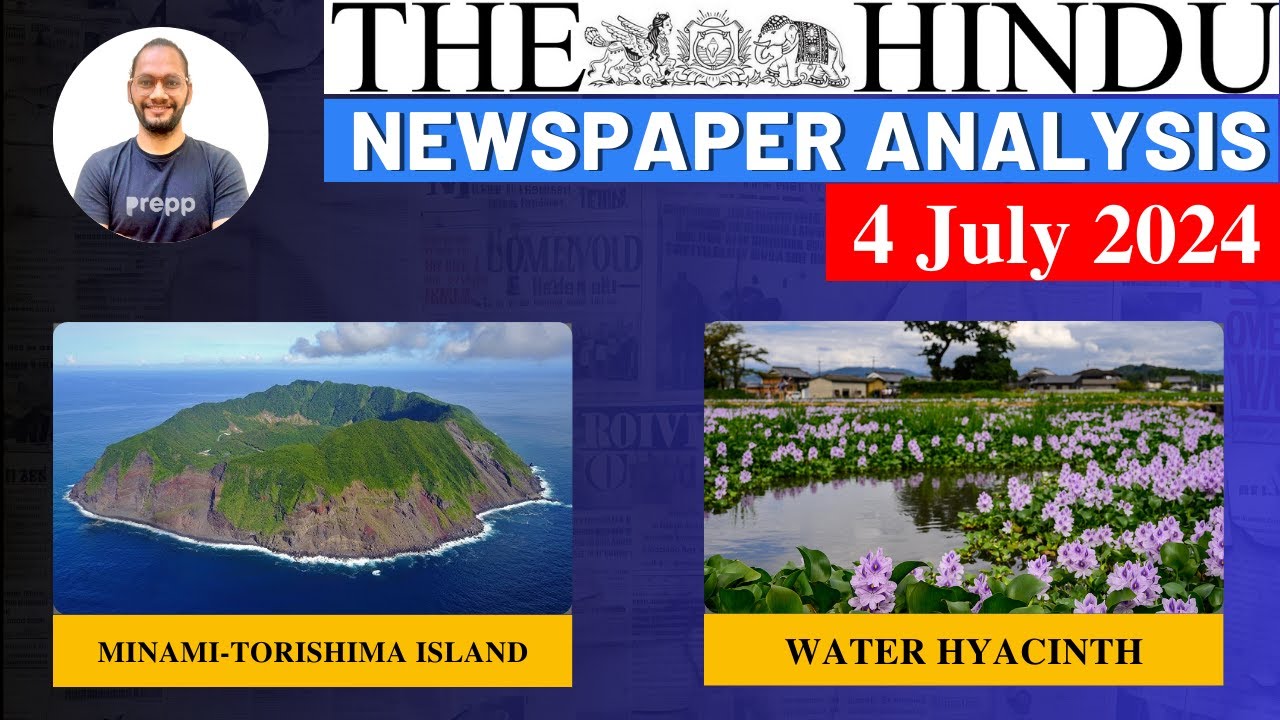
04 July 2024 Daily Current Affairs Analysis
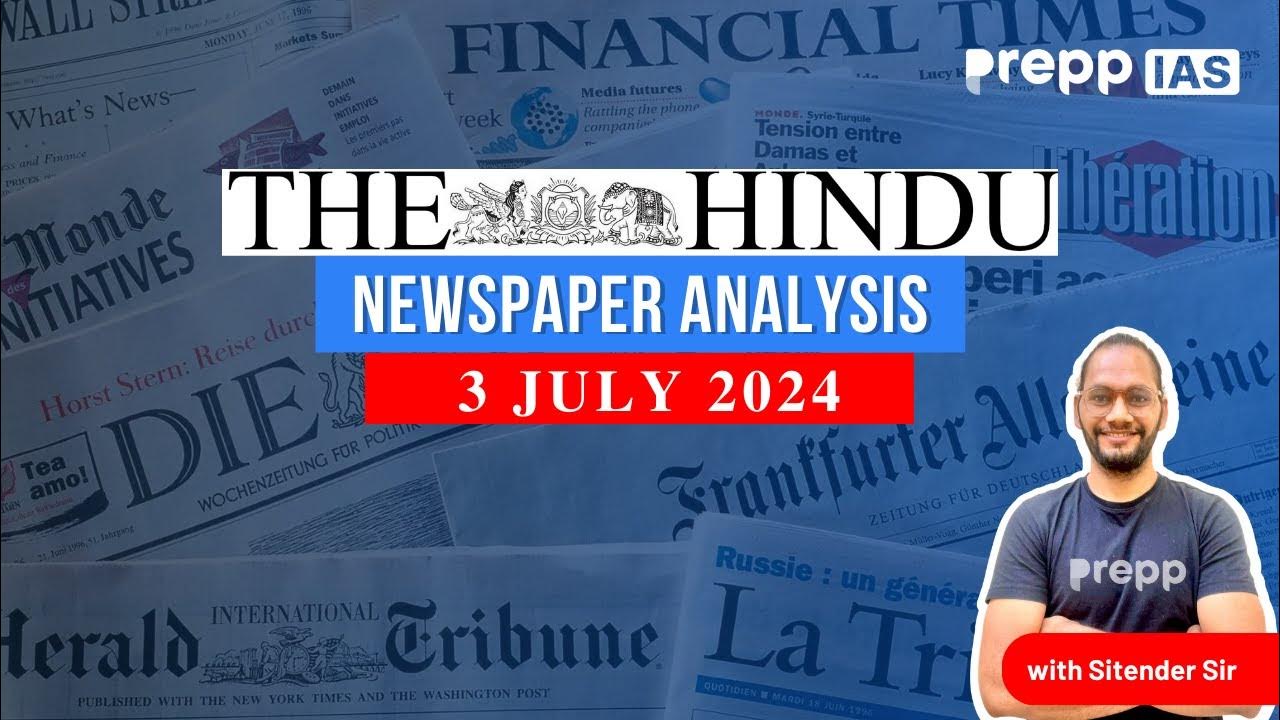
03 July 2024 Daily Current Affairs Analysis
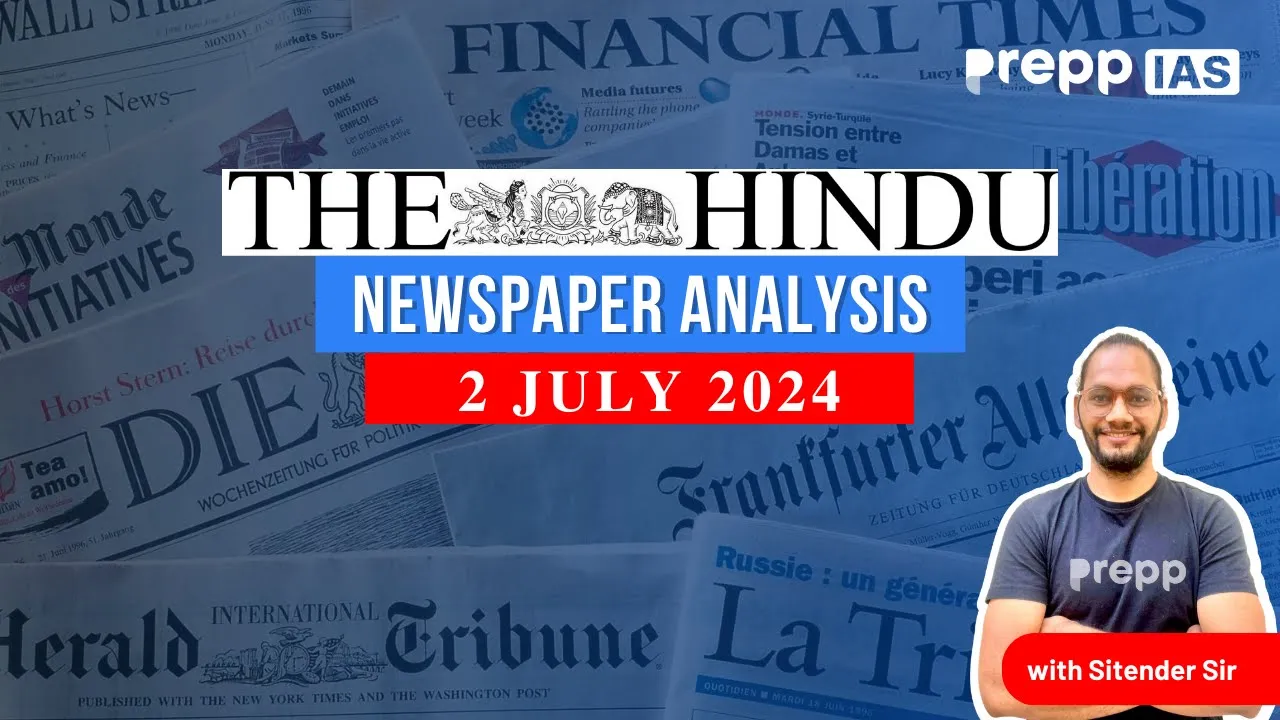
02 July 2024 Daily Current Affairs Analysis
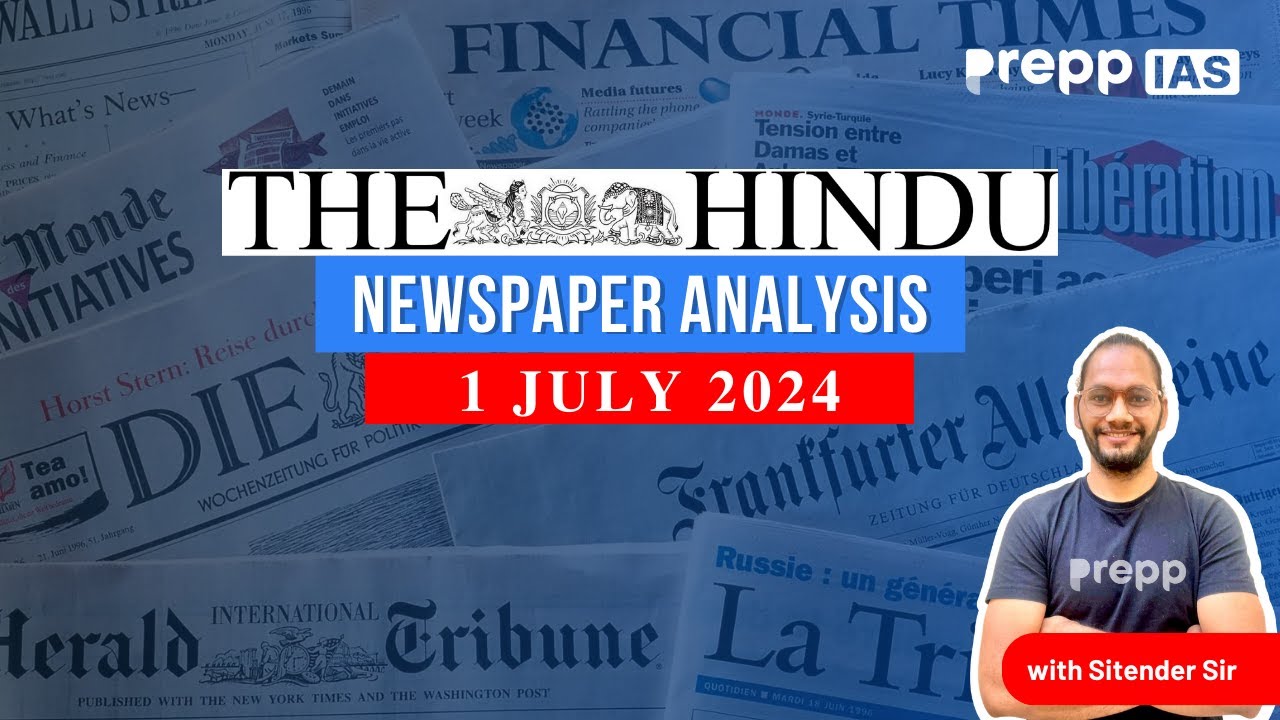
01 July 2024 Daily Current Affairs Analysis
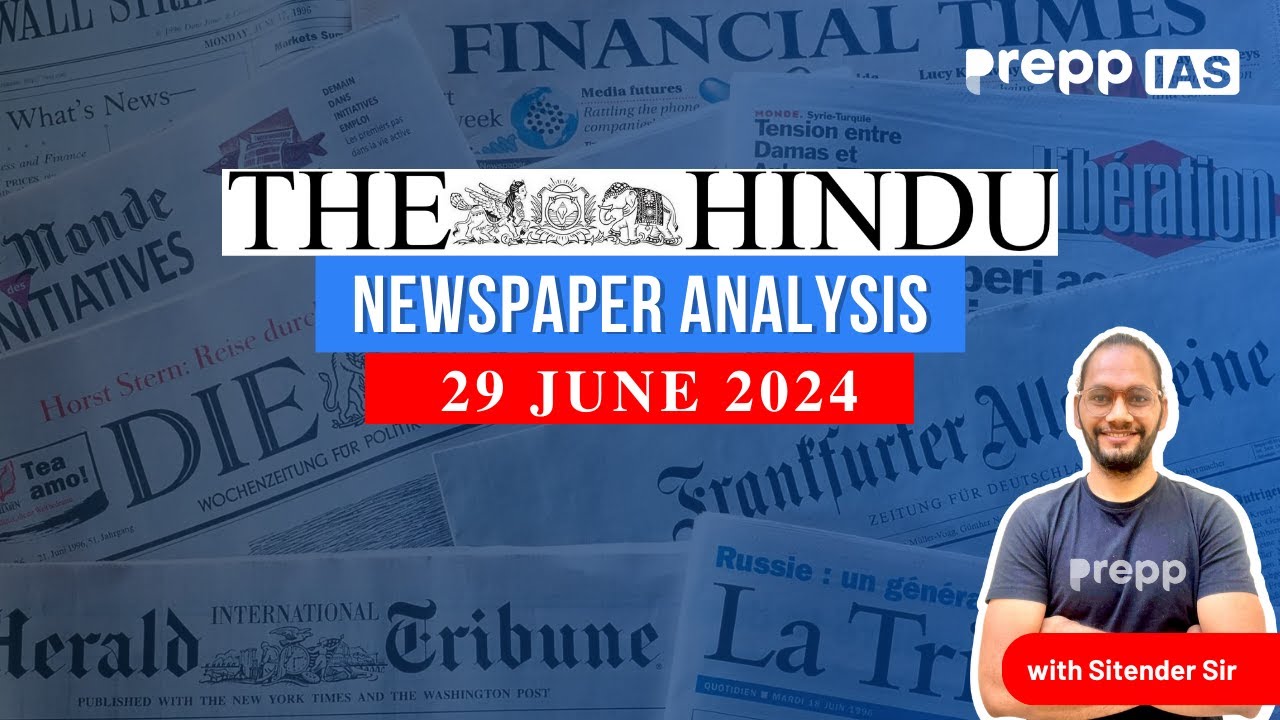
29 June 2024 Daily Current Affairs Analysis
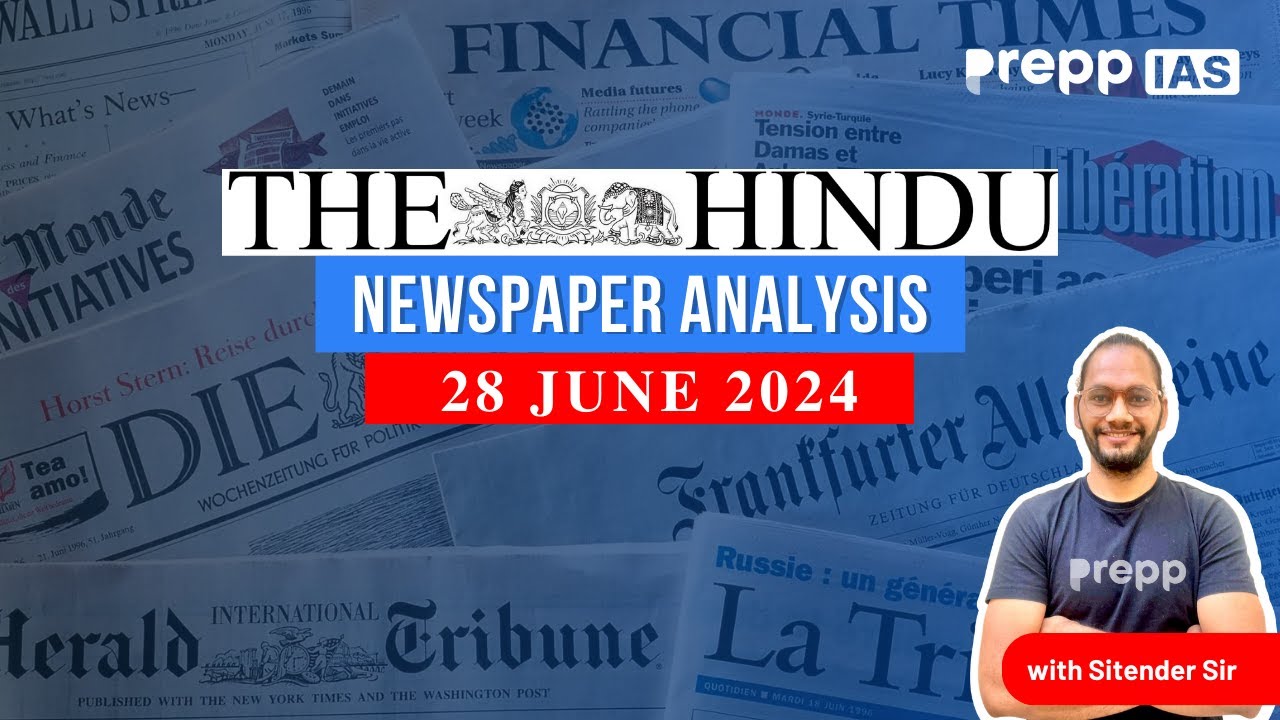
28 June 2024 Daily Current Affairs Analysis
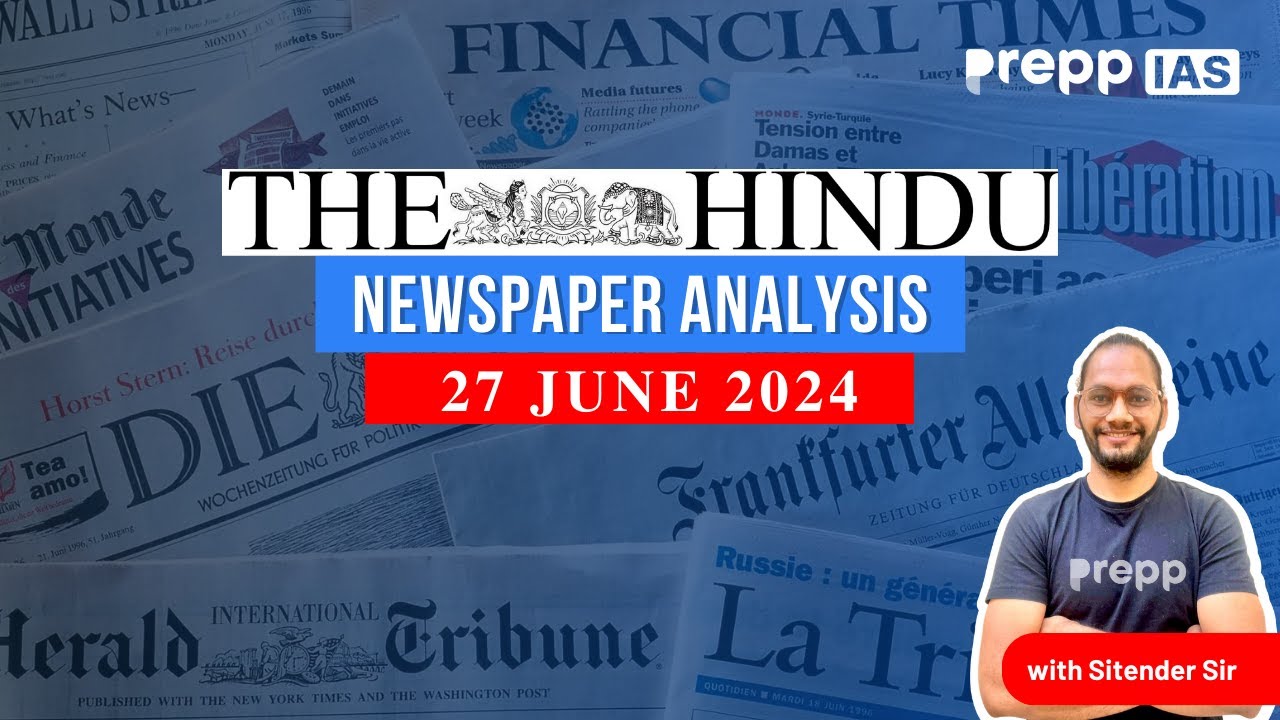
27 June 2024 Daily Current Affairs Analysis
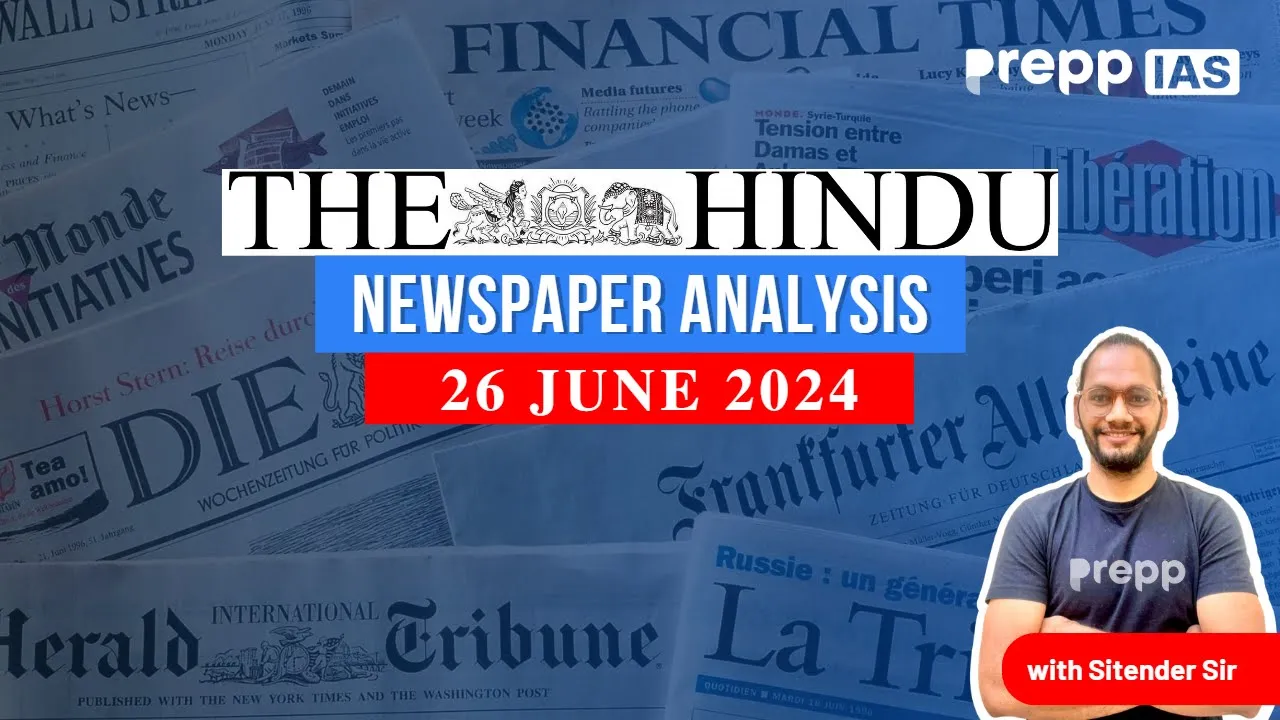
26 June 2024 Daily Current Affairs Analysis
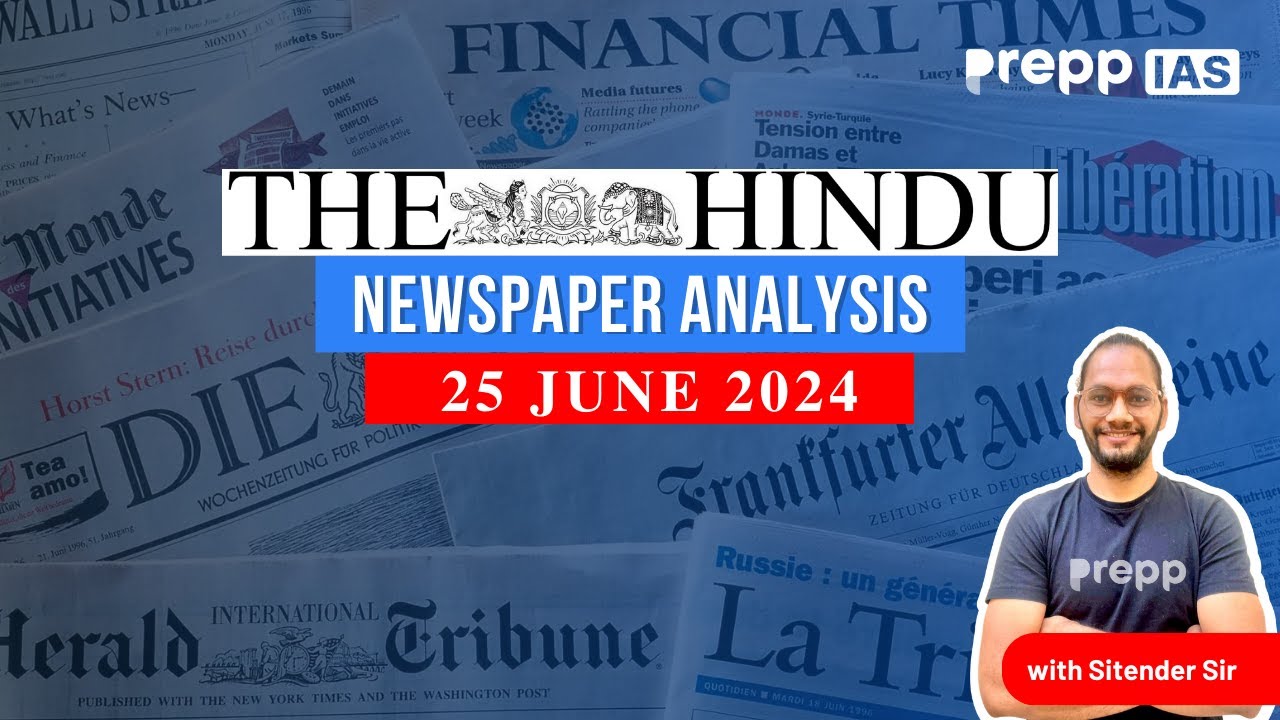
25 June 2024 Daily Current Affairs Analysis

Thank you for visiting nature.com. You are using a browser version with limited support for CSS. To obtain the best experience, we recommend you use a more up to date browser (or turn off compatibility mode in Internet Explorer). In the meantime, to ensure continued support, we are displaying the site without styles and JavaScript.
- View all journals
- Explore content
- About the journal
- Publish with us
- Sign up for alerts
- Published: 31 October 2016
Tidal evolution of the Moon from a high-obliquity, high-angular-momentum Earth
- Matija Ćuk 1 ,
- Douglas P. Hamilton 2 ,
- Simon J. Lock 3 &
- Sarah T. Stewart 4
Nature volume 539 , pages 402–406 ( 2016 ) Cite this article
8945 Accesses
76 Citations
614 Altmetric
Metrics details
- Early solar system
- Rings and moons
In the giant-impact hypothesis for lunar origin, the Moon accreted from an equatorial circum-terrestrial disk; however, the current lunar orbital inclination of five degrees requires a subsequent dynamical process that is still unclear 1 , 2 , 3 . In addition, the giant-impact theory has been challenged by the Moon’s unexpectedly Earth-like isotopic composition 4 , 5 . Here we show that tidal dissipation due to lunar obliquity was an important effect during the Moon’s tidal evolution, and the lunar inclination in the past must have been very large, defying theoretical explanations. We present a tidal evolution model starting with the Moon in an equatorial orbit around an initially fast-spinning, high-obliquity Earth, which is a probable outcome of giant impacts. Using numerical modelling, we show that the solar perturbations on the Moon’s orbit naturally induce a large lunar inclination and remove angular momentum from the Earth–Moon system. Our tidal evolution model supports recent high-angular-momentum, giant-impact scenarios to explain the Moon’s isotopic composition 6 , 7 , 8 and provides a new pathway to reach Earth’s climatically favourable low obliquity.
This is a preview of subscription content, access via your institution
Access options
Subscribe to this journal
Receive 51 print issues and online access
185,98 € per year
only 3,65 € per issue
Buy this article
- Purchase on Springer Link
- Instant access to full article PDF
Prices may be subject to local taxes which are calculated during checkout
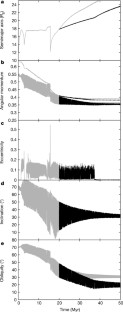
Similar content being viewed by others
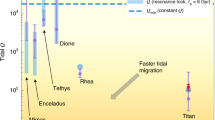
Resonance locking in giant planets indicated by the rapid orbital expansion of Titan
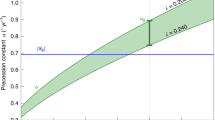
The large obliquity of Saturn explained by the fast migration of Titan

Atmospheric dynamics of a near tidally locked Earth-sized planet
Touma, J. & Wisdom, J. Resonances in the early evolution of the Earth–Moon system. Astron. J. 115 , 1653–1663 (1998)
Article ADS Google Scholar
Ward, W. R. & Canup, R. M. Origin of the Moon’s orbital inclination from resonant disk interactions. Nature 403 , 741–743 (2000)
Article ADS CAS PubMed Google Scholar
Pahlevan, K. & Morbidelli, A. Collisionless encounters and the origin of the lunar inclination. Nature 527 , 492–494 (2015)
Burkhardt, C. Isotopic Composition of the Moon and the Lunar Isotopic Crisis 1–13 (Springer, 2015)
Young, E. D. et al. Oxygen isotopic evidence for vigorous mixing during the Moon-forming giant impact. Science 351 , 493–496 (2016)
Ćuk, M. & Stewart, S. T. Making the Moon from a fast-spinning Earth: a giant impact followed by resonant despinning. Science 338 , 1047–1052 (2012)
Article ADS PubMed CAS Google Scholar
Canup, R. M. Forming a Moon with an Earth-like composition via a giant impact. Science 338 , 1052–1055 (2012)
Article ADS CAS PubMed PubMed Central Google Scholar
Lock, S. J. et al. A new model for lunar origin: equilibration with Earth beyond the hot spin stability limit. Lunar Planet. Sci. Conf. 47 , 2881 (2016)
ADS Google Scholar
Hartmann, W. K. & Davis, D. R. Satellite-sized planetesimals and lunar origin. Icarus 24 , 504–515 (1975)
Cameron, A. G. W. & Ward, W. R. The origin of the moon. Lunar Planet. Sci. Conf. 7 , 120 (1976)
Goldreich, P. History of lunar orbit. Rev. Geophys. 4 , 411–439 (1966)
Touma, J. & Wisdom, J. Evolution of the Earth–Moon system. Astron. J. 108 , 1943–1961 (1994)
Canup, R. M. & Asphaug, E. Origin of the Moon in a giant impact near the end of the Earth’s formation. Nature 412 , 708–712 (2001)
Meier, M. M. M. Earth’s titanium twin. Nat. Geosci. 5 , 240–241 (2012)
Article ADS CAS Google Scholar
Pahlevan, K. & Stevenson, D. J. Equilibration in the aftermath of the lunar-forming giant impact. Earth Planet. Sci. Lett. 262 , 438–449 (2007)
Melosh, H. J. New approaches to the Moon’s isotopic crisis. Phil. Trans. R. Soc. Lond. A 372 , http://dx.doi.org/10.1098/rsta.2013.0168 (2014)
Wisdom, J. & Tian, Z. Early evolution of the Earth–Moon system with a fast-spinning Earth. Icarus 256 , 138–146 (2015)
Ward, W. R. Past orientation of the lunar spin axis. Science 189 , 377–379 (1975)
Chyba, C. F., Jankowski, D. G. & Nicholson, P. D. Tidal evolution in the Neptune–Triton system. Astron. Astrophys. 219 , L23–L26 (1989)
Chen, E. M. A. & Nimmo, F. Tidal dissipation in the early lunar magma ocean and its effect on the evolution of the Earth–Moon system. Icarus 275 , 132–142 (2016)
Tyler, R. H. Strong ocean tidal flow and heating on moons of the outer planets. Nature 456 , 770–772 (2008)
Nicholson, P. D., Cuk, M., Sheppard, S. S., Nesvorny, D. & Johnson, T. V. in Irregular Satellites of the Giant Planets 411–424 (Univ. Arizona Press, 2008)
Tremaine, S., Touma, J. & Namouni, F. Satellite dynamics on the Laplace surface. Astron. J. 137 , 3706–3717 (2009)
Kozai, Y. Secular perturbations of asteroids with high inclination and eccentricity. Astron. J. 67 , 591 (1962)
Article ADS MathSciNet Google Scholar
Tamayo, D., Burns, J. A., Hamilton, D. P. & Nicholson, P. D. Dynamical instabilities in high-obliquity systems. Astron. J. 145 , 54 (2013)
Atobe, K. & Ida, S. Obliquity evolution of extrasolar terrestrial planets. Icarus 188 , 1–17 (2007)
Rubincam, D. P. Tidal friction in the Earth–Moon system and Laplace planes: Darwin redux. Icarus 266 , 24–43 (2016)
Kokubo, E. & Genda, H. Formation of terrestrial planets from protoplanets under a realistic accretion condition. Astrophys. J. Lett. 714 , 21–25 (2010)
Garrick-Bethell, I., Wisdom, J. & Zuber, M. T. Evidence for a past high-eccentricity lunar orbit. Science 313 , 652–655 (2006)
Article ADS MathSciNet CAS MATH PubMed Google Scholar
Keane, J. T. & Matsuyama, I. Evidence for lunar true polar wander and a past low-eccentricity, synchronous lunar orbit. Geophys. Res. Lett. 41 , 6610–6619 (2014)
Peale, S. J. & Cassen, P. Contribution of tidal dissipation to lunar thermal history. Icarus 36 , 245–269 (1978)
Canup, R. M., Levison, H. F. & Stewart, G. R. Evolution of a terrestrial multiple-moon system. Astron. J. 117 , 603–620 (1999)
Peale, S. J. Generalized Cassini’s Laws. Astron. J. 74 , 483 (1969)
Article ADS MATH Google Scholar
Ćuk, M. & Burns, J. A. On the secular behavior of irregular satellites. Astron. J. 128 , 2518–2541 (2004)
Williams, J. G. & Boggs, D. H. Tides on the Moon: theory and determination of dissipation. J. Geophys. Res. Planets 120 , 689–724 (2015)
Webb, D. J. Tides and the evolution of the Earth–Moon system. Geophys. J. 70 , 261–271 (1982)
Bills, B. G. & Ray, R. D. Lunar orbital evolution: a synthesis of recent results. Geophys. Res. Lett. 26 , 3045–3048 (1999)
Ćuk, M. Excitation of lunar eccentricity by planetary resonances. Science 318 , 244 (2007)
Murray, C. D. & Dermott, S. F. Solar System Dynamics (Cambridge Univ. Press, 1999)
Wisdom, J. & Holman, M. Symplectic maps for the N -body problem. Astron. J. 102 , 1528–1538 (1991)
Chambers, J. E., Quintana, E. V., Duncan, M. J. & Lissauer, J. J. Symplectic integrator algorithms for modeling planetary accretion in binary star systems. Astron. J. 123 , 2884–2894 (2002)
Ćuk, M., Dones, L. & Nesvorný, D. Dynamical evidence for a late formation of Saturn’s Moons. Astrophys. J. 820 , 97 (2016)
Touma, J. & Wisdom, J. Lie-Poisson integrators for rigid body dynamics in the solar system. Astron. J. 107 , 1189–1202 (1994)
Vokrouhlický, D., Breiter, S., Nesvorný, D. & Bottke, W. F. Generalized YORP evolution: onset of tumbling and new asymptotic states. Icarus 191 , 636–650 (2007)
Sharma, I., Burns, J. A. & Hui, C.-Y. Nutational damping times in solids of revolution. Mon. Not. R. Astron. Soc. 359 , 79–92 (2005)
Burns, J. A. Elementary derivation of the perturbation equations of celestial mechanics. Am. J. Phys. 44 , 944–949 (1976)
Meyer, J., Elkins-Tanton, L. & Wisdom, J. Coupled thermal-orbital evolution of the early Moon. Icarus 208 , 1–10 (2010); corrigendum Icarus 212, 448–449 (2011)
Laskar, J. et al. A long-term numerical solution for the insolation quantities of the Earth. Astron. Astrophys. 428 , 261–285 (2004)
Lidov, M. L. The evolution of orbits of artificial satellites of planets under the action of gravitational perturbations of external bodies. Planet. Space Sci. 9 , 719–759 (1962)
Innanen, K. A., Zheng, J. Q., Mikkola, S. & Valtonen, M. J. The Kozai mechanism and the stability of planetary orbits in binary star systems. Astron. J. 113 , 1915 (1997)
Carruba, V., Burns, J. A., Nicholson, P. D. & Gladman, B. J. On the inclination distribution of the Jovian irregular satellites. Icarus 158 , 434–449 (2002)
Beletskii, V. V. Resonance rotation of celestial bodies and Cassini’s laws. Celestial Mech. 6 , 356–378 (1972)
Article ADS MathSciNet MATH Google Scholar
Gladman, B., Quinn, D. D., Nicholson, P. & Rand, R. Synchronous locking of tidally evolving satellites. Icarus 122 , 166–192 (1996)
Wisdom, J. Dynamics of the lunar spin axis. Astron. J. 131 , 1864–1871 (2006)
Rambaux, N. & Williams, J. G. The Moon’s physical librations and determination of their free modes. Celestial Mech. Dyn. Astron. 109 , 85–100 (2011)
Wieczorek, M. A., Correia, A. C. M., Le Feuvre, M., Laskar, J. & Rambaux, N. Mercury’s spin-orbit resonance explained by initial retrograde and subsequent synchronous rotation. Nat. Geosci. 5 , 18–21 (2011)
Meyer, J. & Wisdom, J. Precession of the lunar core. Icarus 211 , 921–924 (2011)
Download references
Acknowledgements
This work was supported by NASA’s Emerging Worlds programme, award NNX15AH65G.
Author information
Authors and affiliations.
Carl Sagan Center, SETI Institute, 189 North Bernardo Avenue, Mountain View, 94043, California, USA
Department of Astronomy, University of Maryland, Physical Sciences Complex, College Park, 20742, Maryland, USA
Douglas P. Hamilton
Department of Earth and Planetary Sciences, Harvard University, 20 Oxford Street, Cambridge, 02138, Massachusetts, USA
- Simon J. Lock
Department of Earth and Planetary Sciences, University of California Davis, One Shields Avenue, Davis, 95616, California, USA
Sarah T. Stewart
You can also search for this author in PubMed Google Scholar
Contributions
M.Ć. designed the study, wrote the software, analysed the data and wrote the paper. Co-authors contributed ancillary calculations, discussed the results and wider implications of the work, and helped edit and improve the paper.

Corresponding author
Correspondence to Matija Ćuk .
Ethics declarations
Competing interests.
The authors declare no competing financial interests.
Additional information
Reviewer Information Nature thanks D. Stevenson and the other anonymous reviewer(s) for their contribution to the peer review of this work.
Extended data figures and tables
Extended data figure 1 semi-analytical model of the lunar tidal evolution..
Evolution of lunar inclination ( a ) and obliquity ( b ) as the Moon evolves from 25 R E to 60 R E using our semi-analytical model (Methods subsection ‘Damping of lunar inclination by obliquity tides’). Initial inclinations were chosen so that the final lunar inclination was the current value of about 5°, while the obliquity was calculated assuming the Moon was in a Cassini state (jumps between 30 R E and 35 R E are due to the transitions between Cassini states 1 and 2) 18 . Love numbers were set at their current values ( k 2,E = 0.3, k 2,M = 0.024) 35 , and the current lunar shape was assumed. The black and red lines plot the solutions for Q M = 10,000 and Q M = 38 (current value), respectively, while Q E was in the range 33–35 range (it was adjusted so that a semimajor axis of 60 R E was reached after 4,500 Myr). The blue line plots a history assuming Q M = 100 interior to 40 R E , and Q M = 38 after the Moon passes that distance. The black line closely resembles the results of studies 11 , 12 that neglected lunar obliquity tides, while the other two curves indicate that the past lunar inclination must have been much larger owing to lunar obliquity tides.
Extended Data Figure 2 Lunar tidal evolution following planetesimal encounters.
Evolution of lunar inclination ( a ) and eccentricity ( b ) following the excitation of the lunar inclination by encounters with planetesimals as proposed by ref. 3 , using our semi-analytical model. The two sets of initial conditions are for the state a = 47 R E , i = 5.8° featured in ref. 3 ( Fig. 1 ), and a possible outcome with a more excited inclination i = 10° (also at a = 47 R E ). The initial eccentricities were estimated as e = 2sin i . The black lines show the evolution assuming Q M = 38 and Q E = 34, with the current Love numbers. The red line plots the evolution for Q M = 100 and the blue line the evolution for Q E = 20. The circle symbol plots the current inclination and eccentricity of the lunar orbit. No combination of tidal parameters can simultaneously match both the current lunar inclination and eccentricity at the same time. A small Q M combined with high e also keeps the Moon from reaching 60 R E (top two lines). Decreasing Q E also does not help, as stronger Earth tides further increase the lunar eccentricity (blue line).
Extended Data Figure 3 A snapshot of the simulation shown in Fig. 1 taken at 12.8 Myr.
The left-hand panels show eccentricity ( a ), lunar inclination and Earth’s obliquity with respect to the ecliptic ( b ), and the argument of perigee of lunar orbit, with the ecliptic as fundamental plane ( c ) versus time over a 500-year period, while the right-hand panels plot lunar eccentricity ( d ) and inclination ( e ) against the Moon’s argument of perigee (over the whole period of 30,000 yr). The eccentricity is clearly correlated with the argument of perigee, as expected for Kozai-type perturbations 24 , 49 . Rapid mutual precession of Earth’s spin axis and the plane of the Moon’s orbit, which are substantially inclined to one another (and to the ecliptic plane), clearly affects both the eccentricity and the perigee precession. This secular behaviour is periodic, with exactly three inclination cycles per one eccentricity cycle, which corresponds to half of the period of precession of the argument of perigee.
Extended Data Figure 4 A snapshot of Q E / k 2,E = 200 simulation shown in Fig. 1 (black line) at 34.6 Myr.
At this time, eccentricity excitation ( a ) and the associated variation of Earth’s obliquity ( b ) are not due to Kozai perturbations, but owing to the slow-varying near-resonant argument Ψ = 3 Ω + 2 ω − 3 γ ( c ), where Ω and γ are longitudes of the lunar ascending node and Earth’s vernal equinox, respectively, and ω is the Moon’s argument of perigee. This near-resonant interaction is responsible for the substantial reduction of Earth’s obliquity seen in Fig. 1 .
Extended Data Figure 5 Early tidal evolution of the Moon with Q E / k 2,E = 200 throughout the simulations.
Extended data figure 6 early tidal evolution of the moon with earth initially having a 2-h spin period., extended data figure 7 map of lunar rotational dynamics close to the cassini state transition..
Outcomes of 512 simulations probing the end states of initially very fast and very slow lunar rotations for 16 different lunar semimajor axes a and 16 different lunar inclinations i . Simulations were run for 1 Myr, except for the rightmost three columns, which were followed for 3 Myr. Each a − i field is described by two symbols, one each for initial rotations of 127 rad yr −1 and 381 rad yr −1 . Green and blue boxes indicate synchronous rotation in Cassini states 1 and 2, respectively. Crosses indicate non-synchronous rotation with stable obliquity, with large orange crosses indicating sub-synchronous rotation, and small magenta crosses plotting super-synchronous states. Red crosses signify variations in obliquity above 1° during the last 50 kyr of the simulation (indicating excited or chaotic spin axis precession).
Extended Data Figure 8 Lunar obliquity close to the Cassini state transition.
Obliquities for four ‘slices’ in inclination (at 5°, 10°, 15° and 20°) from the grid of short simulations shown in Extended Data Fig. 7 (solid red and magenta lines with points; the obliquities and inclinations are in the same order at far left and far right). When two different simulations for the same a and i differed in outcome, we chose the solution within the Cassini state, if available. The blue dashed lines plot the relevant Cassini states calculated using analytical formulae, while the black dashed line at 58.15° plots the upper limit for stable obliquities in the relevant Cassini state. Although the numerical and analytical results agree at the smallest and largest semimajor axes, the large discrepancies in between are due to non-synchronous rotations being dominant at the Cassini state transition.
Extended Data Figure 9 The Moon’s wobble as it approaches the annual resonance in Fig. 4 .
The rotation rate around the longest axis of the Moon ( a ) and the angle between the longest axis and Earth ( b ) during the first phase of lunar tidal evolution (red points in Fig. 4 ) within 29.7 R E , where we accelerated the tidal evolution by a factor of a hundred. The wobble is clearly building up as the Moon is approaching the resonance between its free wobble and Earth’s orbital period at about 29.7 R E . The growth in lunar libration angle is more influenced by increasing lunar obliquity ( Fig. 4b ) than the increase in the amplitude of the wobble.
Extended Data Figure 10 Passage through the annual resonance of the lunar free wobble in Fig. 4 .
Lunar obliquity ( a ), spin rate ( b ), rotation rates around the longest and shortest principal axes ( c ), and the angle between the Moon’s longest axis and Earth ( d ) during the first 1 Myr of the ‘blue’ segment of lunar tidal evolution in Fig. 4 (which was simulated at the nominal rate for tidal evolution). The free wobble (tracked by grey points in c ) experiences a resonance at about 330 kyr, breaking the Moon’s synchronous rotation. Since the Moon is close to the Cassini state transition, it cannot evolve back into Cassini state 1 and it settles into a non-synchronous high-obliquity state 58 .
Supplementary information
Animation of relative orientations of earth's spin and the moon's orbit during the laplace plane transition, following the simulation plotted in black in fig. 1..
The system is seen from the direction of Earth's vernal equinox, the blue arrow is plotted along Earth's spin axis and points to the north, while the lunar orbit is plotted in red. Initially the Earth has a high obliquity, the Moon has low inclination and the Laplace plane is close to Earth's equator. As the animation progresses and the lunar orbit grows due to tidal dissipation, the Laplace plane shifts to the ecliptic plane (horizontal in this view). The moon acquires a large inclination during the Laplace plane transition, while Earth's obliquity decreases. The labels show time, Earth's spin period, total angular momentum (scaled to the present value) and angular momentum of the Earth-Moon system where only the ecliptic component (i.e. that along the vertical axis) of the lunar orbital momentum is taken into account. Unlike total angular momentum, this ecliptic component will be conserved during the Cassini state transition. (AVI 25361 kb)
Animation of relative orientations of lunar figure and orbit during the Cassini state transition, following the simulation plotted in Fig. 4.
The Moon is seen from the direction of the ascending node of lunar orbit, with the ecliptic plane (i.e. the Moon's Laplace plane at this time) parallel to the horizontal axis. The red arrow shows the orientation of the Moon's orbit normal. At first the Moon's orbit normal and spin axis are on the same side of the normal to the ecliptic, indicating that the Moon is in Cassini state 1. Once the Cassini state 1 is destabilized, after some wobbling, the Moon settles in a non-synchronous state somewhat similar to the Cassini state 2 (with the orbit normal and the spin axis being on opposite sides of the normal to the ecliptic). During this time both the inclination and obliquity (which is forced by inclination) are being damped by strong obliquity tides. At the semimajor axis of 35.1 Earth radii, the Moon becomes synchronous again and enters the Cassini state 2, where it stays for the rest of the simulation (this event is visible as a 5-degree jump in obliquity). (AVI 18922 kb)
PowerPoint slides
Powerpoint slide for fig. 1, powerpoint slide for fig. 2, powerpoint slide for fig. 3, powerpoint slide for fig. 4, rights and permissions.
Reprints and permissions
About this article
Cite this article.
Ćuk, M., Hamilton, D., Lock, S. et al. Tidal evolution of the Moon from a high-obliquity, high-angular-momentum Earth. Nature 539 , 402–406 (2016). https://doi.org/10.1038/nature19846
Download citation
Received : 06 April 2016
Accepted : 09 September 2016
Published : 31 October 2016
Issue Date : 17 November 2016
DOI : https://doi.org/10.1038/nature19846
Share this article
Anyone you share the following link with will be able to read this content:
Sorry, a shareable link is not currently available for this article.
Provided by the Springer Nature SharedIt content-sharing initiative
This article is cited by
Early thermal evolution and planetary differentiation of the moon: a giant impact perspective.
- Vishal Goyal
- Sandeep Sahijpal
Journal of Earth System Science (2022)
The Exosphere as a Boundary: Origin and Evolution of Airless Bodies in the Inner Solar System and Beyond Including Planets with Silicate Atmospheres
- Helmut Lammer
- Manuel Scherf
- Petra Odert
Space Science Reviews (2022)
Geochemical Constraints on the Origin of the Moon and Preservation of Ancient Terrestrial Heterogeneities
- Katherine R. Bermingham
Space Science Reviews (2020)
Depletion of potassium and sodium in mantles of Mars, Moon and Vesta by core formation
- E. S. Steenstra
- W. van Westrenen
Scientific Reports (2018)
By submitting a comment you agree to abide by our Terms and Community Guidelines . If you find something abusive or that does not comply with our terms or guidelines please flag it as inappropriate.
Quick links
- Explore articles by subject
- Guide to authors
- Editorial policies
Sign up for the Nature Briefing newsletter — what matters in science, free to your inbox daily.

Chamberlin, thomas chrowder (1843–1928); chamberlin—moulton planetesimal hypothesis
- Reference work entry
- Cite this reference work entry
- Rhodes W. Fairbridge &
- Norriss S. Hetherington
Part of the book series: Encyclopedia of Earth Science ((EESS))
133 Accesses
Chamberlin was an outstanding geologist and planetary scientist who, with F.R. Moulton (a distinguished mathematician and specialist in celestial mechanics), jointly developed the planetesimal hypothesis for the accretionary origin of planets, asteroids and the natural satellites. The term ‘planetesimal’ (q.v.), coined by Chamberlin, described the small, cold particles or ‘dirty snowballs’ (Shapley's term) of the primeval dust cloud believed to have been the source of the solar system (sometimes called the ‘dust-cloud hypothesis’). The nebular hypothesis of Kant—Laplace was vigorously attacked by Chamberlin and rejected. While the Kant—Laplace model required a very hot beginning, Chamberlin and Moulton's model permitted a cold accretion such as indicated by the geochemical evidence in the Earth's Precambrian rocks, which display salt deposits and glacial cycles. Chamberlin was also interested in the study of meteorites and proposed the ‘chondrulitic hypothesis’ for the origin of...
This is a preview of subscription content, log in via an institution to check access.
Access this chapter
Institutional subscriptions
Bibliography
Brush, S. G. (1978) A geologist among astronomers: the rise and fall of the Chamberlin—Moulton cosmogony. J. Hist. Astron., 9, 1–41, 77–104.
Google Scholar
Chamberlin, R. T. (1934) Biographical memoir of Thomas Chrowder Chamberlin, 1843–1928. Natl Acad. Sci. Biogr. Mem., 15, pp. 305–407.
Chamberlin, T. C. (1897) A group of hypotheses bearing on climatic changes, J. Geol., 5, 653–83.
Fenton, C. L. and Fenton, M. A. (1952) Giants of Geology. Garden City, NY: Doubleday, 333 pp.
Fleming, J. R. (1992) T.C. Chamberlin and H 2 O climate feedbacks: a voice from the past. Eos, 73(47), 505–6.
Hetherington, N. S. (1992) Converting an hypothesis into a research program: T.C. Chamberlin, his planetesimal hypothesis, and its effect on research at the Mt Wilson Observatory, in The Earth, the Heavens, and the Carnegie Institution of Washington; Historical Perspectives after Ninety Years (ed. G. A. Good). Washington, DC: American Geophysical Union.
MacMillan, W. D. (1929) The field of cosmogony (development of Chamberlin's planetesimal hypothesis). J. Geol., 27, 341–56.
Mather, K. F. (1971). Chamberlin, Thomas Chrowder. Dict. Sci. Biogr., Vol. 3, pp. 189–91.
Moulton, F. R. (1900) An attempt to test the nebular hypothesis by an appeal to the laws of motion. Astrophys. J., 11, 103–30.
Willis, B. (1929) Memorial of Thomas Chrowder Chamberlin. Geol. Soc. Am. Bull., 40, 23–45.
Cross references
Lowell, Percival ; Nebular hypothesis (Kant—Laplace) ; Planetesimal
Download references
You can also search for this author in PubMed Google Scholar
Rights and permissions
Reprints and permissions
Copyright information
© 1997 Chapman & Hall
About this entry
Cite this entry.
Fairbridge, R.W., Hetherington, N.S. (1997). Chamberlin, thomas chrowder (1843–1928); chamberlin—moulton planetesimal hypothesis. In: Encyclopedia of Planetary Science. Encyclopedia of Earth Science. Springer, Dordrecht. https://doi.org/10.1007/1-4020-4520-4_60
Download citation
DOI : https://doi.org/10.1007/1-4020-4520-4_60
Publisher Name : Springer, Dordrecht
Print ISBN : 978-0-412-06951-2
Online ISBN : 978-1-4020-4520-2
eBook Packages : Springer Book Archive
Share this entry
Anyone you share the following link with will be able to read this content:
Sorry, a shareable link is not currently available for this article.
Provided by the Springer Nature SharedIt content-sharing initiative
- Publish with us
Policies and ethics
- Find a journal
- Track your research
ENCYCLOPEDIC ENTRY
Tidal energy.
Tidal energy is power produced by the surge of ocean waters during the rise and fall of tides. Tidal energy is a renewable source of energy.
Earth Science, Geography, Physical Geography, Social Studies, Economics
Loading ...

Tidal energy is produced by the surge of ocean waters during the rise and fall of tides . Tidal energy is a renewable source of energy . During the 20th century, engineers developed ways to use tidal movement to generate electricity in areas where there is a significant tidal range —the difference in area between high tide and low tide . All methods use special generators to convert tidal energy into electricity . Tidal energy production is still in its infancy . The amount of power produced so far has been small. There are very few commercial -sized tidal power plants operating in the world. The first was located in La Rance, France. The largest facility is the Sihwa Lake Tidal Power Station in South Korea. The United States has no tidal plants and only a few sites where tidal energy could be produced at a reasonable price. China, France, England, Canada, and Russia have much more potential to use this type of energy . In the United States, there are legal concerns about underwater land ownership and environmental impact . Investors are not enthusiastic about tidal energy because there is not a strong guarantee that it will make money or benefit consumers . Engineers are working to improve the technology of tidal energy generators to increase the amount of energy they produce, to decrease their impact on the environment, and to find a way to earn a profit for energy companies. Tidal Energy Generators There are currently three different ways to get tidal energy : tidal streams , barrages , and tidal lagoons . For most tidal energy generators , turbines are placed in tidal streams . A tidal stream is a fast-flowing body of water created by tides . A turbine is a machine that takes energy from a flow of fluid . That fluid can be air (wind) or liquid (water). Because water is much more dense than air, tidal energy is more powerful than wind energy . Unlike wind, tides are predictable and stable . Where tidal generators are used, they produce a steady, reliable stream of electricity . Placing turbines in tidal streams is complex , because the machines are large and disrupt the tide they are trying to harness . The environmental impact could be severe , depending on the size of the turbine and the site of the tidal stream . Turbines are most effective in shallow water. This produces more energy and allows ships to navigate around the turbines . A tidal generator 's turbine blades also turn slowly, which helps marine life avoid getting caught in the system. The world's first tidal power station was constructed in 2007 at Strangford Lough in Northern Ireland. The turbines are placed in a narrow strait between the Strangford Lough inlet and the Irish Sea. The tide can move at 4 meters (13 feet) per second across the strait .
Barrage Another type of tidal energy generator uses a large dam called a barrage . With a barrage , water can spill over the top or through turbines in the dam because the dam is low. Barrages can be constructed across tidal rivers , bays , and estuaries. Turbines inside the barrage harness the power of tides the same way a river dam harnesses the power of a river. The barrage gates are open as the tide rises. At high tide , the barrage gates close, creating a pool, or tidal lagoon . The water is then released through the barrage 's turbines , creating energy at a rate that can be controlled by engineers . The environmental impact of a barrage system can be quite significant . The land in the tidal range is completely disrupted . The change in water level in the tidal lagoon might harm plant and animal life. The salinity inside the tidal lagoon lowers, which changes the organisms that are able to live there. As with dams across rivers, fish are blocked into or out of the tidal lagoon . Turbines move quickly in barrages , and marine animals can be caught in the blades. With their food source limited, birds might find different places to migrate . A barrage is a much more expensive tidal energy generator than a single turbine . Although there are no fuel costs, barrages involve more construction and more machines. Unlike single turbines , barrages also require constant supervision to adjust power output. The tidal power plant at the Rance River estuary in Brittany, France, uses a barrage . It was built in 1966 and is still functioning . The plant uses two sources of energy : tidal energy from the English Channel and river current energy from the Rance River. The barrage has led to an increased level of silt in the habitat . Native aquatic plants suffocate in silt , and a flatfish called plaice is now extinct in the area. Other organisms, such as cuttlefish , a relative of squids , now thrive in the Rance estuary . Cuttlefish prefer cloudy, silty ecosystems . Tidal Lagoon The final type of tidal energy generator involves the construction of tidal lagoons . A tidal lagoon is a body of ocean water that is partly enclosed by a natural or manmade barrier. Tidal lagoons might also be estuaries and have freshwater emptying into them. A tidal energy generator using tidal lagoons would function much like a barrage . Unlike barrages , however, tidal lagoons can be constructed along the natural coastline . A tidal lagoon power plant could also generate continuous power. The turbines work as the lagoon is filling and emptying. The environmental impact of tidal lagoons is minimal . The lagoons can be constructed with natural materials like rock . They would appear as a low breakwater (sea wall) at low tide , and be submerged at high tide . Animals could swim around the structure, and smaller organisms could swim inside it. Large predators like sharks would not be able to penetrate the lagoon, so smaller fish would probably thrive . Birds would likely flock to the area. But the energy output from generators using tidal lagoons is likely to be low. There are no functioning examples yet. China is constructing a tidal lagoon power plant at the Yalu River, near its border with North Korea. A private company is also planning a small tidal lagoon power plant in Swansea Bay , Wales.
DTP Dynamic tidal power (DTP) is one of the newest proposals to harness the power of tides. Using DTP, enormous dams (as long as 50 kilometers (31 miles)) would extend straight from the shore into the open ocean.
Articles & Profiles
Media credits.
The audio, illustrations, photos, and videos are credited beneath the media asset, except for promotional images, which generally link to another page that contains the media credit. The Rights Holder for media is the person or group credited.
Illustrators
Educator reviewer, last updated.
October 19, 2023
User Permissions
For information on user permissions, please read our Terms of Service. If you have questions about how to cite anything on our website in your project or classroom presentation, please contact your teacher. They will best know the preferred format. When you reach out to them, you will need the page title, URL, and the date you accessed the resource.
If a media asset is downloadable, a download button appears in the corner of the media viewer. If no button appears, you cannot download or save the media.
Text on this page is printable and can be used according to our Terms of Service .
Interactives
Any interactives on this page can only be played while you are visiting our website. You cannot download interactives.
Related Resources

Explain the tidal hypothesis.
The evolution of tidal hypotheses in 1919, british scientist sir james jeans developed his "tidal hypothesis" to explain the formation of the earth. the tidal hypothesis is a recent theory for the beginnings of the earth and solar system. jeans' theory was founded on a set of axioms. bernoulli defined the static reaction of the earth's waters to the tidal potential, whereas newton addressed the tide-producing forces. the tidal hypotheses are as follows: once upon a time, the sun was a giant incandescent gaseous mass of the substance. aside from the sun, the cosmos contains another star known as an "intruding star." this star was much bigger than the sun at the time. the sun was similarly stable on its axis, whereas the intruding star was on a predetermined path that would bring it closer to the basic sun. the tidal force "damaged the intruding star" on the surface of the "primitive sun." according to james jeans, the intruding star's immense gravitational field ejected a substantial amount of stuff from the primordial sun, which later provided the building material for future planets..


IMAGES
VIDEO
COMMENTS
The theory of tides is the application of continuum mechanics to interpret and predict the tidal deformations of planetary and satellite bodies and their atmospheres and oceans (especially Earth's oceans) under the gravitational loading of another astronomical body or bodies (especially the Moon and Sun ).
The most widely accepted model of planetary formation is known as the nebular hypothesis.This model posits that, 4.6 billion years ago, the Solar System was formed by the gravitational collapse of a giant molecular cloud spanning several light-years.Many stars, including the Sun, were formed within this collapsing cloud.The gas that formed the Solar System was slightly more massive than the ...
Tidal hypothesis is one of the modern hypotheses of the origin of the earth and the solar system. Jeans postulated his hypothesis on the basis of certain axioms (self-proved facts) as given below: (1) The solar system was formed from the sun and another intruding star. (2) In the beginning the sun was a big incandescent gaseous mass of matter.
The tidal force or tide-generating force is a gravitational effect that stretches a body along the line towards and away from the center of mass of another body due to spatial variations in strength in gravitational field from the other body. It is responsible for the tides and related phenomena, including solid-earth tides, tidal locking ...
tidal theory. tidal theory Theory of the origin of the solar system, involving the approach near the Sun of another star. This set up tidal forces, and the instability of the Sun resulted in part of its mass being torn off to form the planets. The theory was proposed by Sir James H. Jeans (1877-1946) and Sir Harold Jeffreys.
The theory of tidal motion was a subject of great importance in the eighteenth century. In the spring of 1738, the Académie Royale des Sciences in Paris offered a prize for the best theory on the tides, seeking to explain the causes of the flow and the ebb of the sea (le flux et le reflux de la mer).Ocean tides are the rise and fall of seawater levels caused by the linked effects of the ...
Theory of the origin of the solar system, involving the approach near the Sun of another star. This set up tidal forces, and the instability of the Sun resulted in part of its mass being torn off to form the planets. The theory was proposed by Sir James H. Jeans (1877-1946) and Sir Harold Jeffreys. From: tidal theory in A Dictionary of Earth ...
If there had been a prize for best theory of the tides at this time, similar to that discussed in Section 5, then the possible winner would have been René Descartes (1596-1650), often called "the father of modern philosophy" (Wikipedia, 2022k). His theory of 1644 was the only one to suggest two tides per lunar day, based on a decent ...
Tides can be described in terms of waves. For a tidal wave, the crest is a high tide and the trough is a low tide. The height of a tidal wave is the range (or tidal range) and the mean of the high and the low tides is called mean tide level. Figure 12 illustrates some of these fundamental characteristics of tidal waves.
TIDE ANALYSIS AND TIDAL POWER . Professor Zygmunt Kowalik . College of Fisher ies and Ocean Sciences, University of Alaska, Fairbanks . Dr John L Luick . ... theory shows that the maximum power available from the moving fluid is proportional to fluid density times velocity cubed. Because density of sea water is approximately 900
The tidal hypothesis, as proposed by James Jeans and modified by Harold Jeffreys, enjoyed a long period of popularity and wide acceptance until the end of the first half of the twentieth century, but the hypothesis was severely criticised on various grounds. Even Jeffreys admitted in Guttenberg (1951) that his modified version of the tidal ...
Isaac Newton first conceived the Equilibrium Theory of Tides, also called "static" tides. This is the theory that a hypothetical global ocean exists at unchanging equilibrium with the tide producing forces. The Equilibrium Theory of Tides is highly idealized, even to the point of altering the globe's shape to better align with the ...
The tide-generating force (or its corresponding potential) is still relevant to tidal theory, but as an intermediate quantity (forcing function) rather than as a final result; theory must also consider the Earth's accumulated dynamic tidal response to the applied forces, which response is influenced by ocean depth, the Earth's rotation, and ...
In fact, ocean tides are caused by the global effect of the horizontal components of the tidal forces. Export citation and abstract BibTeX RIS. 1. Introduction. Ocean tides are dynamic, but the equilibrium tide theory, which was first proposed by Newton in his great work Principia, assumes they are always static.
SAO/NASA Astrophysics Data System (ADS) Title: A new tidal theory for the origin of the solar system Authors: Woolfson, M. M. Journal: Royal Astronomical Society, Quarterly Journal, vol. 19, June 1978, p. 167-174. Bibliographic Code: 1978QJRAS..19..167W
Here we refer to the giant-impact theory in which the Earth-Moon post-impact angular momentum (AM) was the same as it is now (in agreement with classic lunar tidal evolution studies 11,12) as ...
that we need a theory that can address formation of both planetary and stellar mass companions in one framework (as believed by Kuiper 1951). 3 TIDAL DOWNSIZING HYPOTHESIS 3.1. Basic steps Tidal Downsizing hypothesis is a sequence of four steps, il-lustrated in Figure 1: (1) A gas clump of Jovian mass is born at separation of ∼100 AU from the ...
Figure 11.1.4 11.1. 4 describes how these forces combine to create the tidal forces. At point O in the center of the Earth, the gravitational force (F g) and the inertial force (F r) are equal, and cancel each other out. On the side of Earth closest to the moon, the inward gravitational force (F g) is greater than the outward inertial force (F ...
The term 'planetesimal' (q.v.), coined by Chamberlin, described the small, cold particles or 'dirty snowballs' (Shapley's term) of the primeval dust cloud believed to have been the source of the solar system (sometimes called the 'dust-cloud hypothesis'). The nebular hypothesis of Kant—Laplace was vigorously attacked by Chamberlin ...
Tidal acceleration is an effect of the tidal forces between an orbiting natural satellite (e.g. the Moon) and the primary planet that it orbits (e.g. Earth ). The acceleration causes a gradual recession of a satellite in a prograde orbit (satellite moving to a higher orbit, away from the primary body), and a corresponding slowdown of the ...
Vocabulary. Tidal energy is produced by the surge of ocean waters during the rise and fall of tides. Tidal energy is a renewable source of energy. During the 20th century, engineers developed ways to use tidal movement to generate electricity in areas where there is a significant tidal range —the difference in area between high tide and low tide.
The evolution of tidal hypotheses. In 1919, British scientist Sir James Jeans developed his "tidal hypothesis" to explain the formation of the earth. The tidal hypothesis is a recent theory for the beginnings of the earth and solar system. Jeans' theory was founded on a set of axioms.
The Moon is Earth's only natural satellite.It orbits at an average distance of 384,400 km (238,900 mi), about 30 times the diameter of Earth. Tidal forces between Earth and the Moon have over time synchronized the Moon's orbital period (lunar month) with its rotation period at 29.5 Earth days, causing the same side of the Moon to always face Earth. . The Moon's gravitational pull - and to a ...
The dark forest hypothesis is the conjecture that many alien civilizations exist throughout the universe, but they are both silent and hostile, maintaining their undetectability for fear of being destroyed by another hostile and undetected civilization. It is one of many possible explanations of the Fermi paradox, which contrasts the lack of contact with alien life with the potential for such ...
The Tidal Zone is a showcase of stories set in the SpongeBob universe with a continuous theme of absurdist and abnormality, hosted by the French Narrator who provides the opening and closing narration of each segment. Throughout the special, GrandPat Star is using a time machine in the form of a closet to find his way back home and occasionally appears in each segment.