Plus.Maths.org
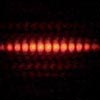

Physics in a minute: The double slit experiment

One of the most famous experiments in physics is the double slit experiment. It demonstrates, with unparalleled strangeness, that little particles of matter have something of a wave about them, and suggests that the very act of observing a particle has a dramatic effect on its behaviour.
To start off, imagine a wall with two slits in it. Imagine throwing tennis balls at the wall. Some will bounce off the wall, but some will travel through the slits. If there's another wall behind the first, the tennis balls that have travelled through the slits will hit it. If you mark all the spots where a ball has hit the second wall, what do you expect to see? That's right. Two strips of marks roughly the same shape as the slits.
In the image below, the first wall is shown from the top, and the second wall is shown from the front.
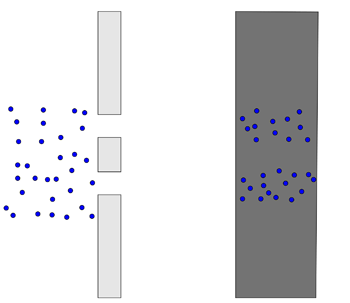
The pattern you get from particles.
Now imagine shining a light (of a single colour, that is, of a single wavelength) at a wall with two slits (where the distance between the slits is roughly the same as the light's wavelength). In the image below, we show the light wave and the wall from the top. The blue lines represent the peaks of the wave. As the wave passes though both slits, it essentially splits into two new waves, each spreading out from one of the slits. These two waves then interfere with each other. At some points, where a peak meets a trough, they will cancel each other out. And at others, where peak meets peak (that's where the blue curves cross in the diagram), they will reinforce each other. Places where the waves reinforce each other give the brightest light. When the light meets a second wall placed behind the first, you will see a stripy pattern, called an interference pattern . The bright stripes come from the waves reinforcing each other.
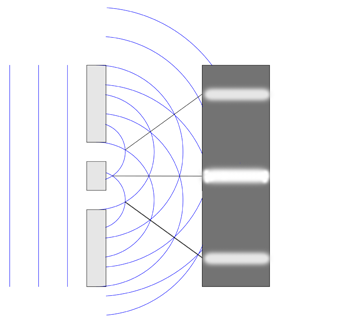
An interference pattern.
Here is a picture of a real interference pattern. There are more stripes because the picture captures more detail than our diagram. (For the sake of correctness, we should say that the image also shows a diffraction pattern , which you would get from a single slit, but we won't go into this here, and you don't need to think about it.)
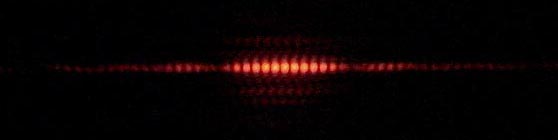
Image: Jordgette , CC BY-SA 3.0 .
Now let's go into the quantum realm. Imagine firing electrons at our wall with the two slits, but block one of those slits off for the moment. You'll find that some of the electrons will pass through the open slit and strike the second wall just as tennis balls would: the spots they arrive at form a strip roughly the same shape as the slit.
Now open the second slit. You'd expect two rectangular strips on the second wall, as with the tennis balls, but what you actually see is very different: the spots where electrons hit build up to replicate the interference pattern from a wave.
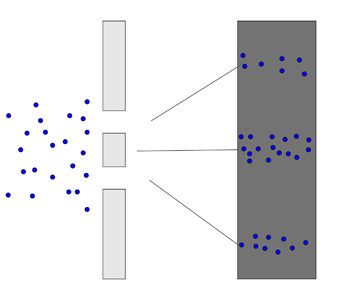
Here is an image of a real double slit experiment with electrons. The individual pictures show the pattern you get on the second wall as more and more electrons are fired. The result is a stripy interference pattern.
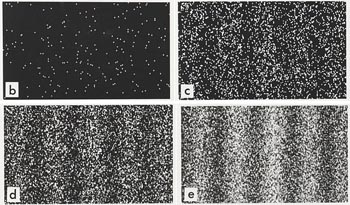
Image: Dr. Tonomura and Belsazar , CC BY-SA 3.0
How can this be?
One possibility might be that the electrons somehow interfere with each other, so they don't arrive in the same places they would if they were alone. However, the interference pattern remains even when you fire the electrons one by one, so that they have no chance of interfering. Strangely, each individual electron contributes one dot to an overall pattern that looks like the interference pattern of a wave.
Could it be that each electrons somehow splits, passes through both slits at once, interferes with itself, and then recombines to meet the second screen as a single, localised particle?
To find out, you might place a detector by the slits, to see which slit an electron passes through. And that's the really weird bit. If you do that, then the pattern on the detector screen turns into the particle pattern of two strips, as seen in the first picture above! The interference pattern disappears. Somehow, the very act of looking makes sure that the electrons travel like well-behaved little tennis balls. It's as if they knew they were being spied on and decided not to be caught in the act of performing weird quantum shenanigans.
What does the experiment tell us? It suggests that what we call "particles", such as electrons, somehow combine characteristics of particles and characteristics of waves. That's the famous wave particle duality of quantum mechanics. It also suggests that the act of observing, of measuring, a quantum system has a profound effect on the system. The question of exactly how that happens constitutes the measurement problem of quantum mechanics.
Further reading
- For an extremely gentle introduction to some of the strange aspects of quantum mechanics, read Watch and learn .
- For a gentle introduction to quantum mechanics, read A ridiculously short introduction to some very basic quantum mechanics .
- For a more detailed, but still reasonably gentle, introduction to quantum mechanics, read Schrödinger's equation — what is it?
Originally published on 05/02/2017.
- Log in or register to post comments
Double slit experiment
The scientist at Washington University found that quasimeasurements cause the zeno effect possibly explaining why the particles do not form a interference pattern if one detects which slit they pass through.
double slit experiment
Seem to be leaving out the fact that the difference occurs when being actively observed.
Heisenberg uncertainty principle
Everything we see is our brain "interpreting" the photons of light reflected off a object. Just like our brains turns 30 FPS and up into a smooth video image. Any experiment that has the word "Observation" in it is flawed. A human used as test equipment for the observation part of a experiment can never be accurate.
It has nothing to do with a human observing anything. It has to do with how one observes things at the atomic and quantum scale. We make these observations by bouncing other particles off of the particles we're interested in examining. At the macro-scale this is not a problem as the particles were bounce off of things are much smaller and have little no affects at the macro.
But at the atomic and smaller scales, the particles we bounce off of things to observe them are similar in "size" (this is a stand in for mass, charge, etc.) to the particles we are trying to observe.
You can think of it like trying to figure out where a billiard ball is by bouncing a golf ball it. That will change the position, spin, etc. of the billiard ball.
I agree with you. Yet, seemingly, the rest of the world either believes - when they do not believe what you've just said - humans have a psychic grip on subatomic particles, or this proves a God exists.
Why not both?
I believe both, and agree with both of you. The two dont have to be mutually exclusive... It actually makes for a more narrow view that way.
Leave room for more
I agree. What we don't know is much greater than what we do and much of what we think we know will change. I think it's best to leave room for many possibilities. Magic is just science undiscovered. If we keep placing boundaries on what's possible and teach others to ignore something for lack of explanations, scientific discovery suffers.
The two slit experiment
The duality of the particle has nothing to do with proving a god exists, just that science is indeterminate and is a duality of possible existences dependent on the observation of a consciousness. Seems like its human consciousness that determines the outcome not any god.
This isn't actually true either. Experiments have shown that even if the photon used to make an observation is of low enough energy that it doesn't alter a large molecules trajectory much, the interference pattern still disappears.
Also, null interaction experiments have been done in which there is no contact between particles at all. But if info about which slit can be found, the interference pattern disappears.
Source for that?
Hey, do you have a source on finding information about which slit with out active observation causing the interference pattern to not appear?
Information on the Double Split experiment
Try QED: The Strange Theory of Light and Matter by Richard P. Feynman. It's been a while since I've enjoyed this book, but my recollection is that it covered the topic well.
Thank you! I didn’t think that explanation made sense, since any effect upon the particle being observed would surely be taken into account in these experiments…and because the detection unit (which “catches” the electrons passing through either slit) doesn’t work by shooting particles at them, as far as I know…even if it did, that wouldn’t be ignored as a variable or whatever…right? I assume such interaction isn’t the method of detection anyway; how the materials used in the experiment could potentially influence the subject being measured is exactly what they control for, among other things- the environment itself, the actions taken as it is conducted and how conditions change…etc. I just don’t think what the commenter described is true since, well, I’d assume the researchers would know that sort of thing could skew the results & therefore lead to an incorrect conclusion. Scientists aren’t just straight up missing the impact of what would be such an obvious flaw in these experiments. I mean, in general they either eliminate the possibility of their tools affecting what they’re measuring OR they take that into account as a variable. Usually the second one is only possible if it’s something that like…as long as it’s known, it won’t render their data useless…if that makes sense (so being aware that it is a factor is key) Anyway…
yet this works at the molecular level too
Molecules are much larger than photons, yet you get the same result.
Quantum Games
If a player has two attached low emission lasers either side of head, beamed through a double slit screen at, say, a home movie or scenario created by the player, bounces back as photons via player's retina to the player's neurons, will player perceive or believe he/she is part of the home movie?
Are you Sure?
Placing only 1 detector in front of one of the double slits ALSO collapses the wave function of both slits. This unequivocally proves that it isn’t the measurement method, but the ACT of measurement itself.
For example, we get the wave pattern. We place a detector in front of both holes we then get 2 bands. ..now, if it were the detector interfering as you mentioned, we will take 1 detector away and leave the other. This way only 1 slit has a detector that interacts with the electron as it passes through.
This would mean the slit with the detector produces a band while the slit without the detector produces a partial striped pattern of the wave.
This is not what we observe however. Measuring just 1 slit still causes 2 bands.
This is because even measuring just 1 slit gives us information on the other. It’s the information that is seemingly the cause of the collapse of the wave function.
Process of Elimination
Hello, I like your idea of removing one detector to see if there is a tangible difference. Given how complex the science behind this is, it doesn't seem ethical to have a biased conclusion with such conviction after just 1 adjustment.
Why has no one tried 3,4,5 slits? With those slits, why haven't we tried removing one detector at a time, and swapping out different ones for each slit? Speeding up or slowing down the particle beam? There are so many variables that could lead to comprehensible evidence if the results are as consistent to previous attempts.
However, it just seems this experiment was done 60 years ago and then we just left it as is with no additional input or further experimentation; just copy paste imitations doing the same thing for educational purposes of the original experiment. That just becomes a history lesson, not a science one.
I come here after watching a conspiracy theory and they referenced this experiment as the leading evidence that we live in a simulation, that the universe is just a projection controlled by a program that detects when its being observed like when a video game detects the players position and renders in whats necessary around them. Suggesting that the light particles can bend the rules of physics and time as soon as someone attempts to measure them.
My initial reaction is not to believe this, however I fully appreciate a rebuttal to come armed with objective, comprehensible evidence like any good debate where possible.
I think this experiment needs a make over and we need to breathe new life into it with far more variables to play with, leaving no stone unturned to draw a general trend and any potential outliers to help solve this once and for all. Or at least, as close as our minds will allow us.
We are our own limitations.
Re: Process of Elimination
I agree with you, the whole furore around this experiment has made it more of a history lesson. It's become almost like the Galileo stone/feather thing; i've had people tell me that in normal every-day circumstances, if i dropped a pen and a pebble at exactly the same moment, they would hit the floor at the same time. Even, when i demonstrate this live, people are still adamant in their stance; after-all, i'm no better than Galileo.
This represents a great discrepancy to the original format of experiment by Galileo. I think some of these experiments have been so popularised in pop-culture and pop-science that people have ruled out the possibility of questioning them. Just like the Galileo stone and feather thing, Everyone is still quoting the same concept from decades ago without any iota of desire to question it. This means that some of these science-cum-history concepts are left to grow into "unquestionables" filled with errors.
I think its about time we re-visit the whole premise of this experiment. Let's introduce 3,4, or even 10 slits! Let's do it today with more control over the variables. We can't let this become another one of the "unquestionables". We can't adopt beliefs and never question them. That would be disastrously dogmatic. The opposite of what it means to do real science!
Also, what was the youtube video you watched? thanks
Uh...no. That is definitely not what happens. If so, there would be no conceptual problem. But don't take my word for it. Here's what Richard Feynman said: “I think I can safely say that nobody really understands quantum mechanics." That's because QM seems to suggest that there's a real connection between the mind of the observer and the results obtained. Also, you're not taking into account the results of the quantum eraser phenomenon, which is another aspect of this experiment that suggests the trajectory of an electron in the past can be altered by an experimenter's actions in the present. You'll have to look it up as it's rather lengthy.
Impact of measuring
Thank you so much for this explanation! We have been fretting about this for quite a while. I wish this physical interaction were more clearly included in other explanations of the slit experiment or just quantum mechanics and general
Question for you
You’re saying to observe a particle we’re bouncing particles off of that observed particle? I don’t think that’s the case. What exactly is bouncing off the particle being observed? How is it being directed toward the target particle? With the way they design these experiments, as far as I know, there should be no overt effect like that- certainly not the actual impact of matter as you’re describing. I don’t think anything is being expelled from the detection materials. Or if it were, that would be taken into account- so precise calculations would be made about how it should impact the results. Basically, if something physical was being intentionally shot at the particles and that somehow was the way we detected them, then the scientists performing the research would do that math using specific measurements (including the mass of that projectile matter). I mean if there was anything being directed toward the electrons or whatever, they’d surely have an idea of what forces it would exert and the interaction it should have, etc… Now you could say the electron being observed has some effect upon the detection unit itself (the “quantum observer”) because logically, in order to even register the electron’s presence/position, it must. But I believe that by all known science, there shouldn’t be any effect upon the particle being observed- other than the fact that it’s being observed. That’s kind of the whole point and is exactly what makes this discovery so mind-blowing…right? So I would assume that in these experiments, they’re controlling for those conditions (the observation device having any physical effect or exerting force upon the observed electron, and all possible variables). Do you disagree? I am genuinely curious about what you’re asserting!
What if there are smaller units than photons, which are presently invisible to our instruments? We'll call them units of consciousness (or thought) that we as conscious beings emanate without knowing it, and it is these very tiny units of consciousness (relative to the photon) that influence the photons to behave as they do. In other words the invisible is influencing the visible like the soul influences the body. These same units could explain the placebo effect.
curtesy call
I agree to your theory. Just want to point it out there as a suggestion to proof read your work before submitting it. I have I found a couple of mistakes where a word had been left out.
Curtesy call
I thought it was well thought out and written. Has it been edited since your comment? If we're being semantic you may wish to check your spelling of courtesy.
Objective observation, unlike subjective observation, is very much allowed in conducting data in experiments.
Plus, unless you have robots conducting the experiment ans/or collecting the data, humans are going to be involved.
observer does not need to be human!
A houseplant would work too. Humans cannot see at this level in any case. Machinery is used, and humans don't have to be in the room for the effects to continue. Observation just means measurement, and Wheeler's Delayed Choice and the Quantum Eraser experiments showed the measurement can occur after the photon, electron, or molecule has hit the wall...and it will still change.
This word is used for convenience, but no conscious observer is required. You can also say “detected”. And by detected what is meant is that information exists that is, in principal, detectable even if not yet technically feasible. Look up “The World’s Smallest Double-Slit Experiment” (2007) and you will find that a single low-energy electron can be an “observer” and collapse the quantum interference pattern of a high-energy electron exiting a single hydrogen molecule.
This is BS. When being observed by a sensor, electrons behave as particles. You lack sufficient understanding of the double slit experiment.
A summarily dismissal of a complex phenomenon that has world class physicists, accompanied by a " You don't understand" line illustrates a simple mind
Deeper understanding is to look at our process of observation.
Everything we recieve through our sences gets interpreted in our brains as being solid, founded by rules/laws/logic & most importantly being OUTSIDE our bodies, ie, being real. Reality is though, that these words you now read, are IN your head as is ALL experiences. Point being, WHY are we being TRICKED to think we are experiencing life OUTSIDE our heads when in fact, we are experiencing life IN our heads, just a observation.
Slit Experiment
The only reason a human may not be good test equipment for observation would be because the person lacks awareness. Human observation can be as accurate as any mechanical scientific devise. It just depends on the awareness of the individual.
by detector , they mean, not human but mechanical
Double Split Experiment
"Seem to be leaving out the fact that the difference occurs when being actively observed" EXACTLY!! This experiment shows that matter is not what we think it is. Scientists have known this for a century yet scientific materialism for some reason still prevails. Matter is a product of Mind. NOT the other way around. For more information read "Ontological Mathematics"
That is not what comes out
That is not what comes out this. "Observer" is a misleading term. It does not specifically refer to humans, nor even conscious creatures, although they can be.
About what you wrote
I do agree with you
double slit Hijinx
Ive noticed this trend everywhere. I chock it up to the materialists clinging to their dead ideology
What happens as the distance between the slits becomes greater ? Is there a relationship between the distance between the slits, and the distance of the source from the slits??
Double slit
What if the light is reacting to the material the slits were cut out from. Maybe electromagnetism causing the light particles to bend and change their direction just like how planets and comets change their orbits when passing near something with mass.

The double slits, are on the
The double slits, are on the both sides of the direction of the flow of light. Also, the slit will be more massive on the two outer edges of the double slits. If it were to be hindered due to presence of slits, wouldn't the effect be more on the outer edges and not on the inner edges, i.e. in the middle.
Double Slit
Such is the problem. Particles don't bend when acted upon by electromagnetic fields. They simply form a trajectory. Bending or warping is the property of a wave. Their trajectory could be altered but I'm sure the material is neutral in all aspects to avoid interference.
Electrons have almost no mass and therefore almost no gravity. Atoms of the slit have a huge mass compared to the electrons. As the electrons passes the slit the gravity of the atoms cause some of the closest electrons to start to spin, the same way water spins when shot through a slit. This spin then sends some of the electrons out of their normal straight line trajectory which causes the apparent wave effect.
If that were the case it would happen if there was only one slit. But it doesn't.
Doesn't bend when "observed".
Electrons spin.
Electrons always have a spin of 1/2. This is a fundemental property of electrons and all fermions. Even if electrons were pushed off their trajectory, how do electrons shot one at a time form an interference pattern?
Because it behaves like wave regardless by itself or by groups
When you say wave, do you mean as in bye bye?
Running paint
If light passes through two slits and it reflects off a object that photon leaves some of itself behind and continues on as if you put paint on your hand and slap a wall then run, how many walls you slap depends on how much paint you got, when light reflects off the object or the slit where does it go is it passing through itself or is it colliding with itself in that direction and handing itself some extra light to continue like say you been running with paint on your hand and you have a train of people that follow you and you are the leader. What if you grab just a finger swap of paint for that extra inch foot mile etc. so when the rays reflects off the rectangle will that rectangle of photons continue colliding into each other and handing itself more photons or snatching some to create a another rectangle and so on till it fades away.
agree with Chris Isaacson
I also thought the materials used may have properties that interfere, and the detector might too.
How does the detector itself work? Does it not rely on an intrinsic property of electrons to function? Connecting the detector to that property then necessarily interferes with the experiment. That interference is then to be expected and can be explained rationally rather than through a spooky effect, quantum effect.
Light/Photon/Electron/Particle interacting with slit material
Agree with you. Details of double/single slit experiments should give more information on the slits material, their dimensions (gap width and distance between slits) and the probability of these materials influencing the path/direction of WAVICLES (waves-cum-particles!). This topic needs to discussed and debated.
The double-slit experiment: Is light a wave or a particle?
The double-slit experiment is universally weird.
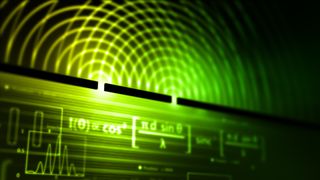
How does the double-slit experiment work?
Interference patterns from waves, particle patterns, double-slit experiment: quantum mechanics, history of the double-slit experiment, additional resources.
The double-slit experiment is one of the most famous experiments in physics and definitely one of the weirdest. It demonstrates that matter and energy (such as light) can exhibit both wave and particle characteristics — known as the particle-wave duality of matter — depending on the scenario, according to the scientific communication site Interesting Engineering .
According to the University of Sussex , American physicist Richard Feynman referred to this paradox as the central mystery of quantum mechanics.
We know the quantum world is strange, but the two-slit experiment takes things to a whole new level. The experiment has perplexed scientists for over 200 years, ever since the first version was first performed by British scientist Thomas Young in 1801.
Related: 10 mind-boggling things you should know about quantum physics
Christian Huygens was the first to describe light as traveling in waves whilst Isaac Newton thought light was composed of tiny particles according to Las Cumbres Observatory . But who is right? British polymath Thomas Young designed the double-slit experiment to put these theories to the test.
To appreciate the truly bizarre nature of the double-split experiment we first need to understand how waves and particles act when passing through two slits.
When Young first carried out the double-split experiment in 1801 he found that light behaved like a wave.
Firstly, if we were to shine a light on a wall with two parallel slits — and for the sake of simplicity, let's say this light has only one wavelength.
As the light passes through the slits, each, in turn, becomes almost like a new source of light. On the far side of the divider, the light from each slit diffracts and overlaps with the light from the other slit, interfering with each other.
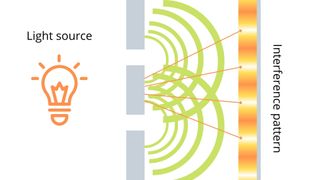
According to Stony Brook University , any wave can create an interference pattern, whether it be a sound wave, light wave or waves across a body of water. When a wave crest hits a wave trough they cancel each other out — known as destructive interference — and appear as a dark band. When a crest hits a crest they amplify each other — known as constructive interference — and appear as a bright band. The combination of dark and bright bands is known as an interference pattern and can be seen on the sensor screen opposite the slits.
This interference pattern was the evidence Young needed to determine that light was a wave and not a particle as Newton had suggested.
But that is not the whole story. Light is a little more complicated than that, and to see how strange it really is we also need to understand what pattern a particle would make on a sensor field.
If you were to carry out the same experiment and fire grains of sand or other particles through the slits, you would end up with a different pattern on the sensor screen. Each particle would go through a slit end up in a line in roughly the same place (with a little bit of spread depending on the angle the particle passed through the slit).
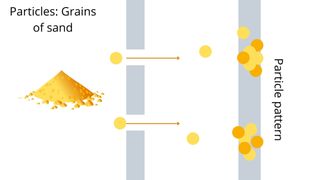
Clearly, waves and particles produce a very different pattern, so it should be easy to distinguish between the two right? Well, this is where the double-slit experiment gets a little strange when we try and carry out the same experiment but with tiny particles of light called photons. Enter the realm of quantum mechanics.
The smallest constituent of light is subatomic particles called photons. By using photons instead of grains of sand we can carry out the double-slit experiment on an atomic scale.
If you block off one of the slits, so it is just a single-slit experiment, and fire photons through to the sensor screen, the photons will appear as pinprick points on the sensor screen, mimicking the particle patterns produced by sand in the previous example. From this evidence, we could suggest that photons are particles.
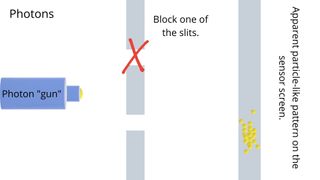
Now, this is where things start to get weird.
If you unblock the slit and fire photons through both slits, you start to see something very similar to the interference pattern produced by waves in the light example. The photons appear to have gone through the pair of slits acting like waves.
But what if you launch photons one by one, leaving enough time between them that they don't have a chance of interfering with each other, will they behave like particles or waves?
At first, the photons appear on the sensor screen in a random scattered manner, but as you fire more and more of them, an interference pattern begins to emerge. Each photon by itself appears to be contributing to the overall wave-like behavior that manifests as an interference pattern on the screen — even though they were launched one at a time so that no interference between them was possible.
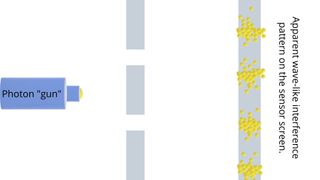
It's almost as though each photon is "aware" that there are two slits available. How? Does it split into two and then rejoin after the slit and then hit the sensor? To investigate this, scientists set up a detector that can tell which slit the photon passes through.
Again, we fire photons one at a time at the slits, as we did in the previous example. The detector finds that about 50% of the photons have passed through the top slit and about 50% through the bottom, and confirms that each photon goes through one slit or the other. Nothing too unusual there.
But when we look at the sensor screen on this experiment, a different pattern emerges.
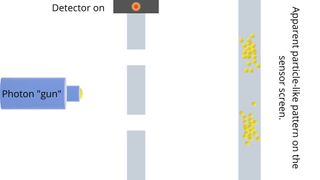
This pattern matches the one we saw when we fired particles through the slits. It appears that monitoring the photons triggers them to switch from the interference pattern produced by waves to that produced by particles.
If the detection of photons through the slits is apparently affecting the pattern on the sensor screen, what happens if we leave the detector in place but switch it off? (Shh, don't tell the photons we're no longer spying on them!)
This is where things get really, really weird.
Same slits, same photons, same detector, just turned off. Will we see the same particle-like pattern?
No. The particles again make a wave-like interference pattern on the sensor screen.
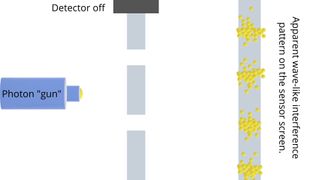
The atoms appear to act like waves when you're not watching them, but as particles when you are. How? Well, if you can answer that, a Nobel Prize is waiting for you.
In the 1930s, scientists proposed that human consciousness might affect quantum mechanics. Mathematician John Von Neumann first postulated this in 1932 in his book " The Mathematical Foundations of Quantum Mechanics ." In the 1960s, theoretical physicist, Eugene Wigner conceived a thought experiment called Wigner's friend — a paradox in quantum physics that describes the states of two people, one conducting the experiment and the observer of the first person, according to science magazine Popular Mechanics . The idea that the consciousness of a person carrying out the experiment can affect the result is knowns as the Von Neumann–Wigner interpretation.
Though a spiritual explanation for quantum mechanic behavior is still believed by a few individuals, including author and alternative medicine advocate Deepak Chopra , a majority of the science community has long disregarded it.
As for a more plausible theory, scientists are stumped.
Furthermore —and perhaps even more astonishingly — if you set up the double-slit experiment to detect which slit the photon went through after the photon has already hit the sensor screen, you still end up with a particle-type pattern on the sensor screen, even though the photon hadn't yet been detected when it hit the screen. This result suggests that detecting a photon in the future affects the pattern produced by the photon on the sensor screen in the past. This experiment is known as the quantum eraser experiment and is explained in more detail in this informative video from Fermilab .
We still don't fully understand how exactly the particle-wave duality of matter works, which is why it is regarded as one of the greatest mysteries of quantum mechanics.
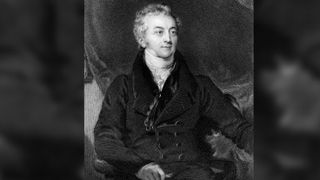
The first version of the double-slit experiment was carried out in 1801 by British polymath Thomas Young, according to the American Physical Society (APS). His experiment demonstrated the interference of light waves and provided evidence that light was a wave, not a particle.
Young also used data from his experiments to calculate the wavelengths of different colors of light and came very close to modern values.
Despite his convincing experiment that light was a wave, those who did not want to accept that Isaac Newton could have been wrong about something criticized Young. (Newton had proposed the corpuscular theory, which posited that light was composed of a stream of tiny particles he called corpuscles.)
According to APS, Young wrote in response to one of the critics, "Much as I venerate the name of Newton, I am not therefore obliged to believe that he was infallible."
Since the development of quantum mechanics, physicists now acknowledge light to be both a particle and a wave.
Explore the double-slit experiment in more detail with this article from the University of Cambridge, which includes images of electron patterns in a double-slit experiment. Discover the true nature of light with Canon Science Lab . Read about fragments of energy that are not waves or particles — but could be the fundamental building blocks of the universe — in this article from The Conversation . Dive deeper into the two-slit experiment in this article published in the journal Nature .
Bibliography
Grangier, Philippe, Gerard Roger, and Alain Aspect. " Experimental evidence for a photon anticorrelation effect on a beam splitter: a new light on single-photon interferences. " EPL (Europhysics Letters) 1.4 (1986): 173.
Thorn, J. J., et al. "Observing the quantum behavior of light in an undergraduate laboratory. " American Journal of Physics 72.9 (2004): 1210-1219.
Ghose, Partha. " The central mystery of quantum mechanics. " arXiv preprint arXiv:0906.0898 (2009).
Aharonov, Yakir, et al. " Finally making sense of the double-slit experiment. " Proceedings of the National Academy of Sciences 114.25 (2017): 6480-6485.
Peng, Hui. " Observations of Cross-Double-Slit Experiments. " International Journal of Physics 8.2 (2020): 39-41.
Join our Space Forums to keep talking space on the latest missions, night sky and more! And if you have a news tip, correction or comment, let us know at: [email protected].
Get the Space.com Newsletter
Breaking space news, the latest updates on rocket launches, skywatching events and more!
Daisy Dobrijevic joined Space.com in February 2022 having previously worked for our sister publication All About Space magazine as a staff writer. Before joining us, Daisy completed an editorial internship with the BBC Sky at Night Magazine and worked at the National Space Centre in Leicester, U.K., where she enjoyed communicating space science to the public. In 2021, Daisy completed a PhD in plant physiology and also holds a Master's in Environmental Science, she is currently based in Nottingham, U.K. Daisy is passionate about all things space, with a penchant for solar activity and space weather. She has a strong interest in astrotourism and loves nothing more than a good northern lights chase!
Hubble Telescope sees 'stellar volcano' erupt in amazing colors (video, photo)
US Space Force awards SpaceX $730 million to launch at least 9 national-security missions
Comet Tsuchinshan-ATLAS is a Halloween visitor from the spooky Oort Cloud − the invisible bubble that's home to countless space objects
Most Popular
- 2 NASA peers into the blistering hot plasma swirling around 12 black holes
- 3 The Pluto problem: Is it time to rethink our definition of a planet?
- 4 'NASA at a crossroads:' Budget woes, aging infrastructure and hard choices ahead
- 5 What happens when black holes merge?
Thank you for visiting nature.com. You are using a browser version with limited support for CSS. To obtain the best experience, we recommend you use a more up to date browser (or turn off compatibility mode in Internet Explorer). In the meantime, to ensure continued support, we are displaying the site without styles and JavaScript.
- View all journals
- Explore content
- About the journal
- Publish with us
- Sign up for alerts
- 13 June 2023
- Correction 20 June 2023
Particle, wave, both or neither? The experiment that challenges all we know about reality
- Anil Ananthaswamy 0
Anil Ananthaswamy is a writer based in California, and author of Through Two Doors at Once .
You can also search for this author in PubMed Google Scholar
You have full access to this article via your institution.
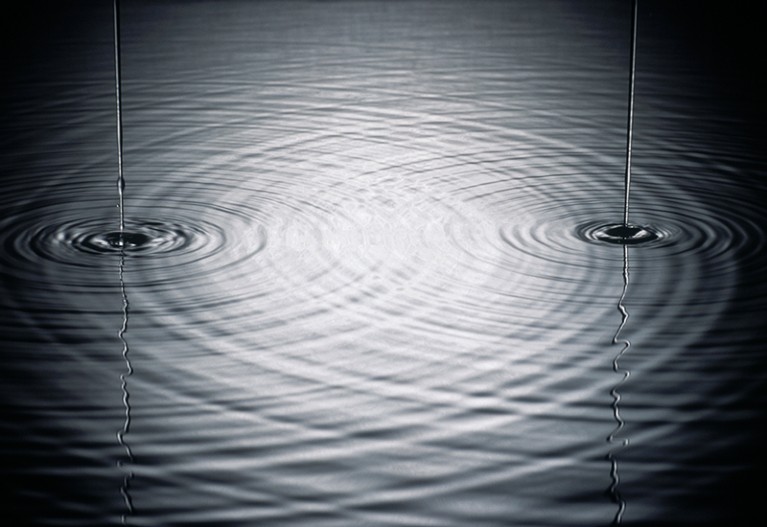
The double-slit experiment’s interference patterns suggest something is in two places at once. Credit: Huw Jones/Getty
Thomas Young, born 250 years ago this week, was a polymath who made seminal contributions in fields from physics to Egyptology . But perhaps his most enduring legacy is proving Isaac Newton wrong about light — and igniting a debate about the nature of reality that still persists.
“The experiments I am about to relate”, he told the Royal Society of London 1 on 24 November 1803, “may be repeated with great ease, whenever the sun shines.” In a simple, modern form, Young’s ‘double-slit’ experiment involves shining light of a single frequency (say, from a red laser) through two fine, parallel openings in an opaque sheet, onto a screen beyond. If light were made of streams of particles, as Newton conjectured, you would expect to see two distinct strips of light on the screen, where the particles pile up after travelling through one slit or the other. But that’s not what happens. Instead, you see many bands of light and dark, strung out in stripes like a barcode: an interference pattern (see ‘Wave–particle weirdness’).
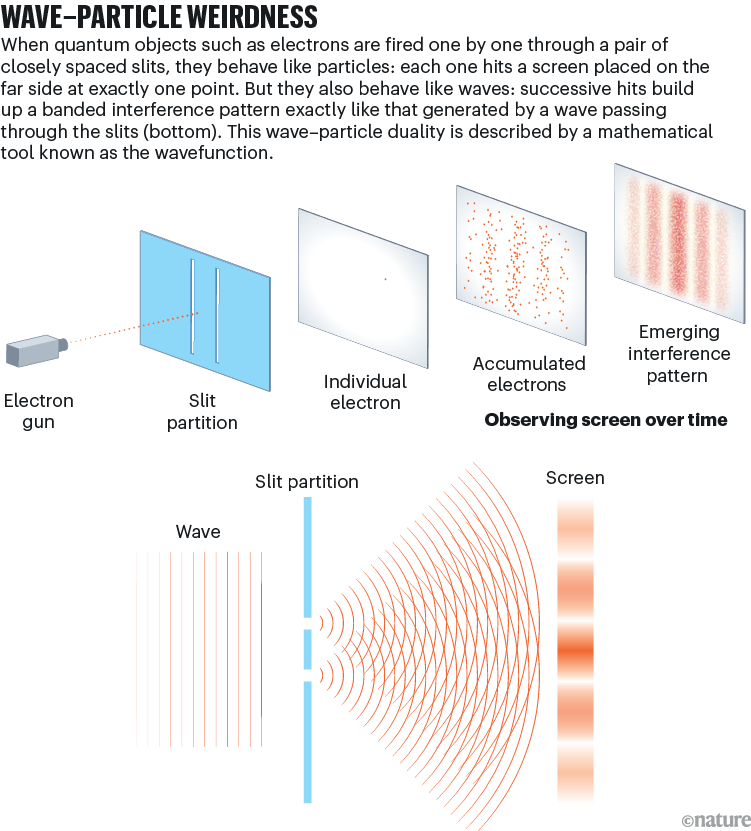
Interference is possible only if light behaves as a wave that strikes both slits at once and diffracts through each, creating two sets of waves on the other side of the slits that propagate towards the screen. Where the crest of one wave overlaps with the crest of the other, you get constructive interference and a patch of light. Where a crest meets a trough, you get destructive interference and darkness.
It’s hard to overstate how wild this discovery was to physicists in Young’s time. But the wildness truly began when Max Planck and Albert Einstein laid the foundations for quantum mechanics in the early twentieth century. Today, quantum mechanics forms a peerlessly accurate framework to explain the basic elements of material reality and their interactions. Pretty early on, it became clear that it implied that light is made of indivisible units of energy called photons — particles, in fact. The amount of energy each carried was proportional to the frequency of the light. Some carry enough of a wallop to knock electrons off atoms of metal, giving us the photoelectric effect that enables today’s solar cells. (It was the study of this effect that led Einstein to his conclusions about light’s particulate nature.)
With the emergence of quantum mechanics, the idea of light as a wave faced a challenge. But it wasn’t as simple as going back to the particle view. Further tests of quantum theory using the double-slit experiment only deepened the mystery. And it hasn’t been solved yet.
Singularly quantum
Imagine, now, that your light source can shoot individual photons of red light at the two slits, while guaranteeing that only one photon goes through the apparatus at any time. A photographic plate on the other side records where the photons land. Classical intuition says each photon can go through only one slit or the other. So, this time, we should see photons accumulating over time and forming two strips of light on the photographic plate. Yet the mathematics of quantum theory implied that the interference pattern would persist.
It was several decades before the technology matured enough to verify these predictions experimentally, using more complex set-ups that were in principle the double-slit. At first, it wasn’t done with photons, but with electrons — entities that we know as particles, but that quantum mechanics predicts act as waves, too. Then, in the 1980s, a team led by Alain Aspect at the Optical Institute in Palaiseau, France, performed the double-slit experiment with single photons 2 . Quantum theory won out: an interference pattern emerged, even when only single particles passed through the slits.
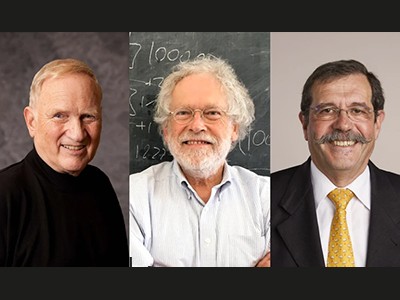
‘Spooky’ quantum-entanglement experiments win physics Nobel
Aspect won a share of the 2022 Nobel prize in physics for his contribution to confirming the predictions of quantum mechanics through experiment. But such experiments leave matters of interpretation wide open. There is simply no way to comprehend what’s happening with minds attuned to the classical world of everyday objects.
When it comes to the double-slit experiment, quantum mechanics does tell a form of story. It says that a photon’s position is described by a mathematical abstraction called the wavefunction — which, as the name suggests, behaves like a wave. This wavefunction, mathematically speaking, hits the two slits, diffracts into two sets of waves and recombines to create the interference pattern. The value of the wavefunction at any location on the photographic plate lets you calculate the probability of finding the photon there. The probability is very high in regions of constructive interference, and very low in regions of destructive interference.
In a sense, then, a photon or any other quantum object acts like both a particle and a wave. This ‘wave–particle duality’ embodies many of the central conceptual mysteries of quantum mechanics that are unresolved to this day. Even if you could know everything about a photon’s initial state, there’s no way to tell exactly where it’ll land on the detector. You have to talk in terms of probabilities given by the wavefunction. These probabilities are borne out only when thousands or tens of thousands of photons are sent through the double slit, one by one.
Before the measurement — in this case, detection by the photographic plate — the mathematics says the particle exists in a superposition of states: in a sense, it has taken both paths, through the right slit and the left. Standard quantum mechanics says that the wavefunction ‘collapses’ when measured, and that the act of observation in some way precipitates that collapse. Before this, the photon has a finite probability of being found in many different regions, but on measurement, the wavefunction peaks at the location in which the photon appears (the probability there equals 1) and is nullified everywhere else (probability equals 0).
It gets even odder. If you can determine which path the photon took on its way to the detector, it acts like a particle that does indeed go through one slit or the other: the interference pattern disappears. But if you cannot glean this ‘which-way’ information, the photon acts like a wave. Whenever there are two or more ways for a photon — or, indeed, any quantum object — to get to a final state, quantum interference occurs.
What’s a wavefunction?
But to generate interference, something has to go through — or at least interact in some way with — both slits. In the mathematics, the wavefunction does the job. Some physicists would say that the wavefunction simply represents information about the quantum system and is not real — in which case it’s hard to explain what interacts with both slits at once. But you can explain the interference pattern if you consider the wavefunction to be real.
This creates its own problems. Imagine a real wavefunction that spreads for kilometres and kilometres before an observer detects the photon. At this point, the wavefunction peaks at the photon’s location, and simultaneously drops to zero everywhere else — over a large, macroscopic distance. This suggests a kind of instantaneous, non-local influence that bothered Einstein no end. One can avoid this with interpretations of quantum theory that don’t collapse the wavefunction, but that opens other cans of worms.
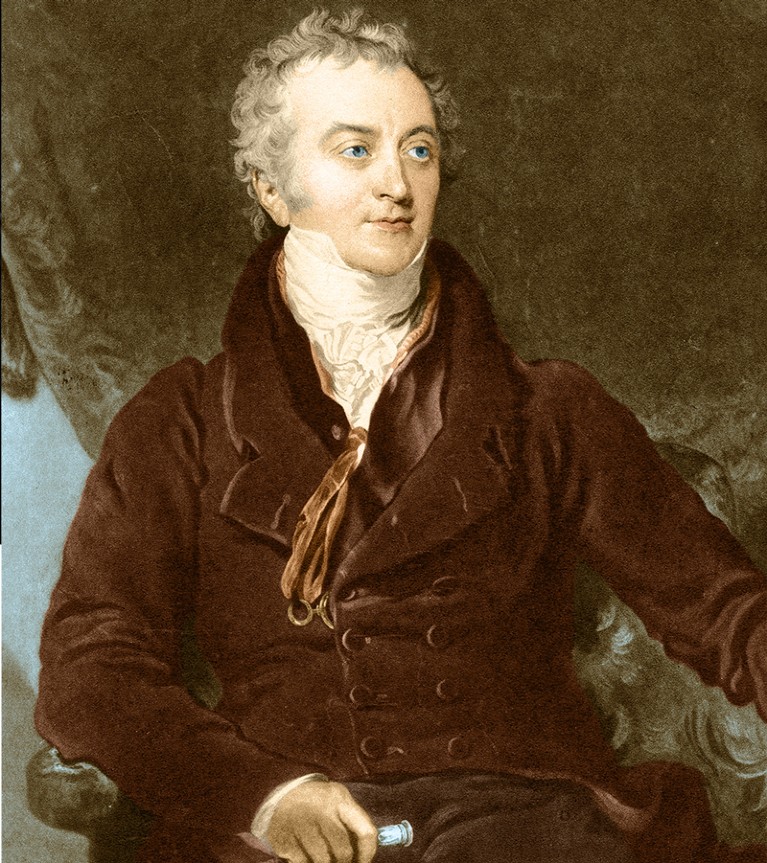
Thomas Young reported the results of the original double-slit experiment in the early nineteenth century. Credit: Photo Researchers/SPL
Perhaps the most notorious is the many-worlds interpretation, the brainchild of US physicist Hugh Everett in the 1950s. This argues that every possible event — in the case of the double slit, a particle going through the left and the right slit — happens, each in its own world. There is no collapse: measurement simply reveals the state of the quantum system in that world. Detractors ask how it’s possible to justify this constant proliferation of worlds, and how, in a many-worlds framework, you can explain why measuring quantum systems yields probabilities, given that there are always definite outcomes in each world.
The de Broglie–Bohm theory, named after quantum pioneers Louis de Broglie and David Bohm, provides another alternative. It says that particles exist with definite positions and momenta, but are guided by an all-encompassing, invisible ‘pilot’ wave, and it’s this wave that goes through both slits. The most profound implication of this theory, that everything is linked to everything else in the Universe by the underlying pilot wave, is one many physicists have trouble accepting.
In the 1970s and 1980s, physicists upgraded the double-slit experiment to seek clarity about the nature of quantum reality, and the perplexing role observation apparently has in collapsing a defined, classical reality out of it. Most notably, John Wheeler at the University of Texas at Austin designed the ‘delayed choice’ thought experiment 3 . Imagine a double-slit set-up that gives the option of gathering or ignoring information about which way the particle went. If you ignore the ‘which-way’ information, you get wave-like behaviour; if you don’t, you get particle-like patterns.
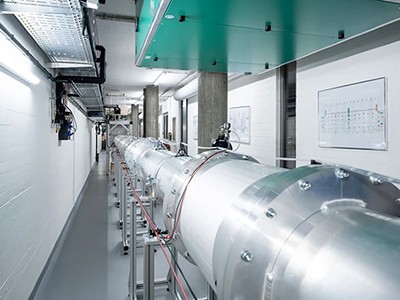
Superconducting qubits cover new distances
With the apparatus on the ‘collect which-way information’ setting, send a photon through the double slits. It should act like a particle and go through one slit or the other. But just before the photon lands on the detector, flip the apparatus to ignore the which-way information. Will the photon, until then supposedly a particle, suddenly switch to being a wave?
Decades later, Aspect’s team performed this experiment with single photons and showed that the answer is yes 4 . Even if the photon had ostensibly travelled through the entire set-up as a particle, switching the apparatus setting so that it ignored which-way information caused it to act like a wave. Did the photon travel back in time and come back through the two slits as a wave? To avoid such nonsensical explanations, Wheeler argued that the only way to make sense of the experiment was to say that the photon has no reality — it’s neither wave nor particle — until it’s detected.
Back in the 1980s, Marlan Scully, then at the University of New Mexico in Albuquerque, and his colleagues came up with a similarly befuddling thought experiment 5 . They imagined collecting the which-way information about a photon by using a second photon ‘entangled’ with the first — a situation in which measuring the quantum state of one tells you about the quantum state of the other. As long as the which-way information can in principle be extracted, the first photon should act like a particle. But if you erase the information in the entangled partner, the mathematics showed, the first photon goes back to behaving like a wave. In 2000, Scully, Yoon-Ho Kim and their colleagues reported performing this experiment 6 . Surprisingly — or unsurprisingly, by this stage — intuition was once again defeated and quantum weirdness reigned supreme.
Larger and still larger
Others are still pushing the double slit in new directions. This year, Romain Tirole at Imperial College London and his colleagues described an experiment in which the slits were temporal: one slit was open at one point in time and the second slit an instant later 7 . A beam of light that goes through these temporal slits produces an interference pattern in its frequency spectrum. Again, the mathematics predicts exactly this behaviour, so physicists aren’t surprised. But it is more proof that the double-slit experiment highlights the lacunae in our understanding of reality, a quarter of a millennium after the birth of the man who devised it.
The double-slit experiment’s place in the pantheon of physics experiments is assured. But it would be further cemented if and when physicists using it were able to work out which theory of the quantum world is correct.
For example, some theories posit that quantum systems that grow bigger than a certain, as-yet-undetermined size randomly collapse into classical systems, with no observer needed. This would explain why macroscopic objects around us don’t obviously work according to quantum rules — but how big does something have to be before it stops acting in a quantum way?
In 2019, Markus Arndt and Yaakov Fein at the University of Vienna and their colleagues reported sending macromolecules called oligoporphyrins, composed of up to 2,000 atoms, through a double slit to see whether they produce an interference pattern 8 . They do, and these patterns can be explained only as a quantum phenomenon. Arndt’s team and others continue to push such experiments to determine whether a line exists between the quantum and the classical world.
Last year, Siddhant Das at the Ludwig Maximilian University of Munich, Germany, and his colleagues analysed the double-slit experiment in the context of the de Broglie–Bohm theory 9 . Unlike standard quantum mechanics, this predicts not just the distribution of particles on the screen that leads to the spatial interference pattern, but also the distribution of when the particles arrive at the screen. The researchers found that their calculations on the distribution of arrival times agreed qualitatively with observations made two decades before, in a double-slit experiment using helium atoms 10 . But it was difficult to prove their case definitively. They are awaiting better data from a similar double-slit experiment done with current technology, to see whether it matches predictions.
And so it goes on, a world away from anything Young or his peers at the Royal Society could have conceived of more than two centuries ago. “Thomas Young would probably scratch his head if he could see the status of today’s experiments,” says Arndt. But that’s because his experiment, so simple in concept, has left us scratching our heads to this day.
Nature 618 , 454-456 (2023)
doi: https://doi.org/10.1038/d41586-023-01938-6
Updates & Corrections
Correction 20 June 2023 : An earlier version of the second picture caption gave the wrong date for when Young reported results of a double-slit experiment.
Young, T. Phil. Trans. R. Soc. 94 , 1–16 (1804).
Google Scholar
Grangier, P., Roger, G. & Aspect, A. Europhys. Lett. 1 , 173 (1986).
Article Google Scholar
Miller, W. A. & Wheeler, J. A. Foundations of Quantum Mechanics in the Light of New Technology (Eds Nakajima, S., Murayama, Y. & Tonomura, A.) 72–84 (World Scientific, 1997).
Jacques, V. et al. Science 315 , 966–968 (2007).
Article PubMed Google Scholar
Scully, M. O. & Drühl, K. Phys. Rev. A. 25 , 2208 (1982).
Kim, Y.-H. et al. Phys. Rev. Lett. 84 , 1 (2000).
Tirole, R. et al. Nature Phys . https://doi.org/10.1038/s41567-023-01993-w (2023).
Fein, Y. Y. et al. Nature Phys. 15 , 1242–1245 (2019).
Das, S., Deckert, D.-A., Kellers, L. & Struyve, W. Preprint at https://arxiv.org/abs/2211.13362 (2022).
Kurtsiefer, Ch., Pfau T. & Mlynek, J. Nature 386 , 150–153 (1997).
Download references
Competing Interests
The author declares no competing interests.
Related Articles
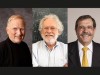
- Quantum physics
- Quantum information
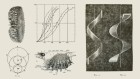
Splendid squirrel sneezes at will
News & Views 22 OCT 24
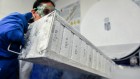
Don’t rush rules for sharing digital genetic-sequence information
Editorial 16 OCT 24
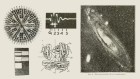
Converting chaos into order: aerial archaeology takes flight in the 1920s
News & Views 15 OCT 24
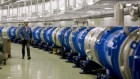
Physicists tame fundamental muon particles into highly controlled beam for first time
News 24 OCT 24
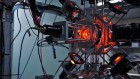
Consider the ethical impacts of quantum technologies in defence — before it’s too late
Comment 22 OCT 24
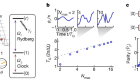
Multi-qubit gates and Schrödinger cat states in an optical clock
Article 09 OCT 24
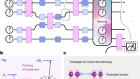
Universal quantum operations and ancilla-based read-out for tweezer clocks
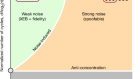
Phase transitions in random circuit sampling
Associate or Senior Editor, Nature Communications (Structural Biology, Biochemistry, or Biophysics)
Job Title: Associate or Senior Editor, Nature Communications (Structural Biology, Biochemistry, or Biophysics) Locations: New York, Philadelphia, S...
New York City, New York (US)
Springer Nature Ltd
Faculty (Open Rank) - Bioengineering and Immunoengineering
The Pritzker School of Molecular Engineering (PME) at the University of Chicago invites applications for multiple faculty positions (open rank) in ...
Chicago, Illinois
University of Chicago, Pritzker School of Molecular Engineering
Postdoctoral Position in Hematology-Oncology – Morizono Lab 2024-2025
The Morizono lab at UCLA is recruiting talented postdoctoral fellows with an interest in the development of gene therapy against HIV-1 infection.
Los Angeles, California
UCLA Department of Medicine - Division of Hematology-Oncology
Associate or Senior Editor (Environmental Social Sciences)
Title: Associate or Senior Editor (Environmental Social Sciences) Organization: Nature Communications Location: New York, Philadelphia, Jersey Ci...
Facility Administrator
APPLICATION CLOSING DATE: December 2nd, 2024 Human Technopole (HT) is an interdisciplinary life science research institute, created and supported b...
Human Technopole
Sign up for the Nature Briefing newsletter — what matters in science, free to your inbox daily.
Quick links
- Explore articles by subject
- Guide to authors
- Editorial policies
Reset password New user? Sign up
Existing user? Log in
Double-slit Experiment
Already have an account? Log in here.
Plane wave representing a particle passing through two slits, resulting in an interference pattern on a screen some distance away from the slits. [1] .
The double-slit experiment is an experiment in quantum mechanics and optics demonstrating the wave-particle duality of electrons , photons , and other fundamental objects in physics. When streams of particles such as electrons or photons pass through two narrow adjacent slits to hit a detector screen on the other side, they don't form clusters based on whether they passed through one slit or the other. Instead, they interfere: simultaneously passing through both slits, and producing a pattern of interference bands on the screen. This phenomenon occurs even if the particles are fired one at a time, showing that the particles demonstrate some wave behavior by interfering with themselves as if they were a wave passing through both slits.
Niels Bohr proposed the idea of wave-particle duality to explain the results of the double-slit experiment. The idea is that all fundamental particles behave in some ways like waves and in other ways like particles, depending on what properties are being observed. These insights led to the development of quantum mechanics and quantum field theory , the current basis behind the Standard Model of particle physics , which is our most accurate understanding of how particles work.
The original double-slit experiment was performed using light/photons around the turn of the nineteenth century by Thomas Young, so the original experiment is often called Young's double-slit experiment. The idea of using particles other than photons in the experiment did not come until after the ideas of de Broglie and the advent of quantum mechanics, when it was proposed that fundamental particles might also behave as waves with characteristic wavelengths depending on their momenta. The single-electron version of the experiment was in fact not performed until 1974. A more recent version of the experiment successfully demonstrating wave-particle duality used buckminsterfullerene or buckyballs , the \(C_{60}\) allotrope of carbon.
Waves vs. Particles
Double-slit experiment with electrons, modeling the double-slit experiment.
To understand why the double-slit experiment is important, it is useful to understand the strong distinctions between wave and particles that make wave-particle duality so intriguing.
Waves describe oscillating values of a physical quantity that obey the wave equation . They are usually described by sums of sine and cosine functions, since any periodic (oscillating) function may be decomposed into a Fourier series . When two waves pass through each other, the resulting wave is the sum of the two original waves. This is called a superposition since the waves are placed ("-position") on top of each other ("super-"). Superposition is one of the most fundamental principles of quantum mechanics. A general quantum system need not be in one state or another but can reside in a superposition of two where there is some probability of measuring the quantum wavefunction in one state or another.
Left: example of superposed waves constructively interfering. Right: superposed waves destructively interfering. [2]
If one wave is \(A(x) = \sin (2x)\) and the other is \(B(x) = \sin (2x)\), then they add together to make \(A + B = 2 \sin (2x)\). The addition of two waves to form a wave of larger amplitude is in general known as constructive interference since the interference results in a larger wave.
If one wave is \(A(x) = \sin (2x)\) and the other is \(B(x) = \sin (2x + \pi)\), then they add together to make \(A + B = 0\) \(\big(\)since \(\sin (2x + \pi) = - \sin (2x)\big).\) This is known as destructive interference in general, when adding two waves results in a wave of smaller amplitude. See the figure above for examples of both constructive and destructive interference.
Two speakers are generating sounds with the same phase, amplitude, and wavelength. The two sound waves can make constructive interference, as above left. Or they can make destructive interference, as above right. If we want to find out the exact position where the two sounds make destructive interference, which of the following do we need to know?
a) the wavelength of the sound waves b) the distances from the two speakers c) the speed of sound generated by the two speakers
This wave behavior is quite unlike the behavior of particles. Classically, particles are objects with a single definite position and a single definite momentum. Particles do not make interference patterns with other particles in detectors whether or not they pass through slits. They only interact by colliding elastically , i.e., via electromagnetic forces at short distances. Before the discovery of quantum mechanics, it was assumed that waves and particles were two distinct models for objects, and that any real physical thing could only be described as a particle or as a wave, but not both.
In the more modern version of the double slit experiment using electrons, electrons with the same momentum are shot from an "electron gun" like the ones inside CRT televisions towards a screen with two slits in it. After each electron goes through one of the slits, it is observed hitting a single point on a detecting screen at an apparently random location. As more and more electrons pass through, one at a time, they form an overall pattern of light and dark interference bands. If each electron was truly just a point particle, then there would only be two clusters of observations: one for the electrons passing through the left slit, and one for the right. However, if electrons are made of waves, they interfere with themselves and pass through both slits simultaneously. Indeed, this is what is observed when the double-slit experiment is performed using electrons. It must therefore be true that the electron is interfering with itself since each electron was only sent through one at a time—there were no other electrons to interfere with it!
When the double-slit experiment is performed using electrons instead of photons, the relevant wavelength is the de Broglie wavelength \(\lambda:\)
\[\lambda = \frac{h}{p},\]
where \(h\) is Planck's constant and \(p\) is the electron's momentum.
Calculate the de Broglie wavelength of an electron moving with velocity \(1.0 \times 10^{7} \text{ m/s}.\)
Usain Bolt, the world champion sprinter, hit a top speed of 27.79 miles per hour at the Olympics. If he has a mass of 94 kg, what was his de Broglie wavelength?
Express your answer as an order of magnitude in units of the Bohr radius \(r_{B} = 5.29 \times 10^{-11} \text{m}\). For instance, if your answer was \(4 \times 10^{-5} r_{B}\), your should give \(-5.\)
Image Credit: Flickr drcliffordchoi.
While the de Broglie relation was postulated for massive matter, the equation applies equally well to light. Given light of a certain wavelength, the momentum and energy of that light can be found using de Broglie's formula. This generalizes the naive formula \(p = m v\), which can't be applied to light since light has no mass and always moves at a constant velocity of \(c\) regardless of wavelength.
The below is reproduced from the Amplitude, Frequency, Wave Number, Phase Shift wiki.
In Young's double-slit experiment, photons corresponding to light of wavelength \(\lambda\) are fired at a barrier with two thin slits separated by a distance \(d,\) as shown in the diagram below. After passing through the slits, they hit a screen at a distance of \(D\) away with \(D \gg d,\) and the point of impact is measured. Remarkably, both the experiment and theory of quantum mechanics predict that the number of photons measured at each point along the screen follows a complicated series of peaks and troughs called an interference pattern as below. The photons must exhibit the wave behavior of a relative phase shift somehow to be responsible for this phenomenon. Below, the condition for which maxima of the interference pattern occur on the screen is derived.
Left: actual experimental two-slit interference pattern of photons, exhibiting many small peaks and troughs. Right: schematic diagram of the experiment as described above. [3]
Since \(D \gg d\), the angle from each of the slits is approximately the same and equal to \(\theta\). If \(y\) is the vertical displacement to an interference peak from the midpoint between the slits, it is therefore true that
\[D\tan \theta \approx D\sin \theta \approx D\theta = y.\]
Furthermore, there is a path difference \(\Delta L\) between the two slits and the interference peak. Light from the lower slit must travel \(\Delta L\) further to reach any particular spot on the screen, as in the diagram below:
Light from the lower slit must travel further to reach the screen at any given point above the midpoint, causing the interference pattern.
The condition for constructive interference is that the path difference \(\Delta L\) is exactly equal to an integer number of wavelengths. The phase shift of light traveling over an integer \(n\) number of wavelengths is exactly \(2\pi n\), which is the same as no phase shift and therefore constructive interference. From the above diagram and basic trigonometry, one can write
\[\Delta L = d\sin \theta \approx d\theta = n\lambda.\]
The first equality is always true; the second is the condition for constructive interference.
Now using \(\theta = \frac{y}{D}\), one can see that the condition for maxima of the interference pattern, corresponding to constructive interference, is
\[n\lambda = \frac{dy}{D},\]
i.e. the maxima occur at the vertical displacements of
\[y = \frac{n\lambda D}{d}.\]
The analogous experimental setup and mathematical modeling using electrons instead of photons is identical except that the de Broglie wavelength of the electrons \(\lambda = \frac{h}{p}\) is used instead of the literal wavelength of light.
- Lookang, . CC-3.0 Licensing . Retrieved from https://commons.wikimedia.org/w/index.php?curid=17014507
- Haade, . CC-3.0 Licensing . Retrieved from https://commons.wikimedia.org/w/index.php?curid=10073387
- Jordgette, . CC-3.0 Licensing . Retrieved from https://commons.wikimedia.org/w/index.php?curid=9529698
Problem Loading...
Note Loading...
Set Loading...
